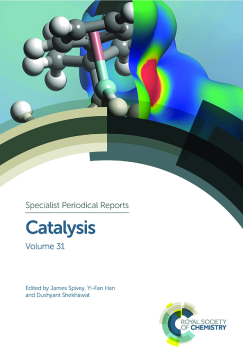
Additional Information
Book Details
Abstract
Catalysts are required for a variety of applications and researchers are increasingly challenged to find cost effective and environmentally benign catalysts to use. This volume looks at modern approaches to catalysis and reviews the extensive literature including direct methane conversion, nanocomposite catalysts for transformation of biofuels into syngas and hydrogen, and catalytic wet air oxidation technology for industrial wastewater treatment. Appealing broadly to researchers in academia and industry, it will be of great benefit to any researcher wanting a succinct reference on developments in this area now and looking to the future.
Table of Contents
Section Title | Page | Action | Price |
---|---|---|---|
Cover | Cover | ||
Preface | vii | ||
Author biographies | x | ||
Contents | xxvii | ||
Catalytic Conversion of Biomass-derived Compounds to C4 Chemicals | 1 | ||
1 Introduction | 1 | ||
2 Biomass-derived platform chemicals for C4 chemical production | 2 | ||
2.1 Succinic acid | 2 | ||
2.2 2,3-Butanediol (23BDO) | 3 | ||
2.3 1,3-Butanediol (13BDO) | 4 | ||
3 C4 Products | 4 | ||
3.1 1,4-Butanediol (14BDO) | 4 | ||
3.2 Gamma-butyrolactone (GBL) | 7 | ||
3.3 Tetrahydrofuran (THF) | 8 | ||
3.4 1,3-Butadiene (BD) | 8 | ||
3.4.1 Ethanol to BD | 10 | ||
3.4.2 23BDO to BD | 12 | ||
3.4.3 13BDO to BD | 16 | ||
3.4.4 14BDO to BD | 17 | ||
3.5 Butene | 18 | ||
3.6 2,3-Butanedione | 21 | ||
3.6.1 MEK to 2,3-Butanedione | 21 | ||
3.6.2 23BDO to 2,3-Butanedione | 22 | ||
3.7 MEK | 22 | ||
3.8 n-Butanol | 23 | ||
3.8.1 Guerbet reaction | 23 | ||
3.8.2 Direct synthesis | 25 | ||
3.9 Isobutanol | 28 | ||
4 Conclusion | 30 | ||
References | 30 | ||
Catalytic wet oxidation: process and catalyst development and the application perspective | 37 | ||
1 Introduction | 37 | ||
2 CWO processes | 38 | ||
2.1 Homogeneous CWO processes | 41 | ||
2.1.1 Loprox process | 41 | ||
2.1.2 Ciba-Geigy process | 42 | ||
2.1.3 IT EnviroScience process | 43 | ||
2.1.4 ATHOS process | 43 | ||
2.1.5 ORCAN process | 44 | ||
2.2 Heterogeneous CWO processes | 45 | ||
2.2.1 Osaka gas process | 45 | ||
2.2.2 Nippon Shokubai process | 46 | ||
2.2.3 Kurita processes | 47 | ||
2.2.4 CALIPHOX process | 48 | ||
2.2.5 DICP process | 49 | ||
2.2.6 Watercatox process | 50 | ||
3 CWO catalysts | 52 | ||
3.1 Homogeneous CWO catalysts | 53 | ||
3.2 Heterogeneous CWO catalysts | 54 | ||
3.2.1 Noble metal catalysts | 54 | ||
3.2.1.1 Pt based catalysts | 55 | ||
3.2.1.2 Ru based catalysts | 55 | ||
3.2.1.3 Pd based catalysts | 57 | ||
3.2.1.4 Au, Ag, Ir and Rh based catalysts | 58 | ||
3.2.2 Non-noble metal catalysts | 58 | ||
3.2.2.1 Cu based catalysts | 59 | ||
3.2.2.2 Fe based catalysts | 59 | ||
3.2.2.3 Ni based catalysts | 60 | ||
3.2.2.4 Mo based catalysts | 60 | ||
3.2.2.5 Ce based catalysts | 61 | ||
3.2.3 Preparation of CWO catalysts | 61 | ||
4 The application perspective of CWO - a case study | 62 | ||
4.1 Properties of the pyrolysis wastewater | 62 | ||
4.2 Treatment of the pyrolysis wastewater | 63 | ||
4.3 CWO of the pyrolysis wastewater | 63 | ||
5 Conclusions | 65 | ||
Acknowledgments | 66 | ||
References | 66 | ||
Opportunities for controlling catalysis by designing molecular environments around active sites: cations supported on amorphous versus crystalline zeolitic silicate supports | 72 | ||
1 Introduction | 72 | ||
2 The molecular environment of a grafted cation active site on a silicate surface: inner- vs. outer-spheres | 73 | ||
2.1 The architecture of an active site: inner-sphere vs. outer-spheres | 73 | ||
2.2 Inner-sphere vs. outer-sphere effects on catalysis: Brønsted acid catalysis by aluminosilicates | 75 | ||
2.2.1 Overview | 75 | ||
2.2.2 Effects of inner-sphere environments | 76 | ||
2.2.3 Effects of outer-sphere environments | 77 | ||
3 Amorphous vs. crystalline zeolitic silicates: supports of identical composition but different structural environments | 79 | ||
3.1 Amorphous silica (SiO2): a disordered support | 79 | ||
3.2 Zeotypes: well-ordered supports | 80 | ||
3.3 Silanols on amorphous silica vs. crystalline zeotype silicates | 81 | ||
3.4 Structural rearrangements of silicates: mechanical rigidity in amorphous vs. zeotype silicates | 83 | ||
4 High external-surface zeotype supports: delaminated zeotypes | 85 | ||
5 Challenges in controlling the incorporation of cations into silicates: a case study of TiIV and FeIII | 88 | ||
5.1 Direct isomorphous substitutions of cations during hydrothermal synthesis | 89 | ||
5.2 Grafting of cation precursors onto silanols: opportunities to control environments through silanol location | 90 | ||
5.3 Effect of density and organization of silanols on grafting | 93 | ||
6 The influence of molecular environment on the catalytic properties of active sites on silicates: a case study of olefin epoxidation catalyzed by TiIV | 96 | ||
6.1 Overview | 96 | ||
6.2 Inner-sphere environment | 98 | ||
6.3 Outer-sphere environment | 100 | ||
6.4 Opportunities for using crystalline zeotype supports for titanosilicate-catalyzed epoxidation in industrial reactors and other emerging applications | 102 | ||
7 Survey of other catalytic systems where the molecular environment on the silicate surface may impact reactivity | 104 | ||
7.1 Alkene metathesis by WOx grafted on silicates | 104 | ||
7.1.1 Overview | 104 | ||
7.1.2 Inner-sphere environment of W | 105 | ||
7.1.3 Outer-sphere environment: evidence of cooperativity with outer-sphere acidity | 108 | ||
7.1.4 Outlook: controlling environments with crystalline supports for olefin metathesis | 109 | ||
7.2 Ethylene polymerization by Cr sites on SiO2 | 109 | ||
7.2.1 Overview | 109 | ||
7.2.2 Inner-sphere environments of Cr | 111 | ||
7.2.3 Outer-sphere: the silica support as an essential component of the active site | 112 | ||
7.2.4 Outlook: controlling environments with crystalline supports | 115 | ||
7.3 Catalysis by silicate defects alone: production of Ɛ-Caprolactam | 115 | ||
7.3.1 Overview | 115 | ||
7.3.2 Active-sites: silanol-rich framework defects | 116 | ||
7.3.3 New opportunities: delaminated zeotypes with enhanced accessibility and control over active site location | 117 | ||
8 Conclusions | 118 | ||
Acknowledgments | 120 | ||
References | 120 | ||
Direct non-oxidative methane conversion in membrane reactor | 127 | ||
1 Direct non-oxidative methane conversion | 127 | ||
1.1 Methane abundance | 127 | ||
1.2 Methane conversion pathways | 128 | ||
1.3 Challenges in direct non-oxidative methane conversion | 130 | ||
2 Catalyst for DNMC in membrane reactor | 133 | ||
2.1 Catalyst for high temperature DNMC | 133 | ||
2.1.1 Metal/zeolite catalyst | 133 | ||
2.1.2 Iron/silica catalyst | 136 | ||
2.2 Catalyst for low temperature DNMC | 137 | ||
2.2.1 Gallium nitride catalyst | 137 | ||
2.2.2 Metal/metal oxide catalyst | 139 | ||
3 Membrane reactor for non-oxidative methane conversion | 140 | ||
3.1 Membrane material | 140 | ||
3.1.1 Polymer membrane | 140 | ||
3.1.2 Nanoporous ceramic membrane | 141 | ||
3.1.3 Palladium membrane | 142 | ||
3.1.4 Dense MIEC ceramic membrane | 143 | ||
3.2 Membrane reactor configuration | 146 | ||
3.3 Membrane reactor performance | 148 | ||
3.3.1 DNMC in Pd-based membrane reactor | 148 | ||
3.3.2 DNMC in MIEC ceramic membrane reactor | 152 | ||
4 Strategies to mitigate membrane reactor deterioration | 157 | ||
4.1 Oxygen permeable membrane to mitigate catalyst deactivation | 157 | ||
4.2 External circuit to improve hydrogen permeation | 158 | ||
5 Summary and outlook | 160 | ||
References | 162 | ||
Understanding atomically dispersed supported metal catalysts: structure and performance of active sites | 166 | ||
1 Introduction | 166 | ||
2 Synthesis techniques | 168 | ||
3 Characterization techniques | 172 | ||
4 Roles of ligands | 174 | ||
4.1 Supports as ligands | 174 | ||
4.1.1 Type of ligands | 174 | ||
4.1.2 Type of metals | 179 | ||
4.1.3 Genesis of catalytically active species | 180 | ||
4.1.4 Activation of reactants by cooperation of metal and support ligands | 180 | ||
4.2 Adsorbates as ligands | 181 | ||
4.2.1 Selectivity control | 181 | ||
4.2.2 Trigger of dynamic structural changes | 184 | ||
4.2.3 Metal migration | 185 | ||
5 Roles of neighboring metal atoms | 186 | ||
6 Conclusion | 189 | ||
References | 190 | ||
Methane activation and conversion on well-defined metal-oxide Surfaces: in situ studies with synchrotron-based techniques | 198 | ||
1 Introduction | 198 | ||
2 Adsorption and activation of methane on oxide and metal/oxide surfaces | 199 | ||
3 Dry-reforming of methane on model metal-oxide surfaces | 203 | ||
4 Direct conversion of methane to methanol on model metal-oxide surfaces | 207 | ||
5 Conclusion and outlook | 212 | ||
Acknowledgments | 213 | ||
References | 213 | ||
Nanocomposite catalysts for transformation of biofuels into syngas and hydrogen: fundamentals of design and performance, application in structured reactors and catalytic membranes | 216 | ||
1 Introduction | 216 | ||
2 Fundamentals of nanocrystalline/nanocomposite materials synthesis | 218 | ||
3 Characterization of nanocomposite materials/catalysts: specificity of real/defect structure, surface properties, oxygen bonding strength, mobility and reactivity | 219 | ||
3.1 Structural features | 219 | ||
3.2 Surface properties | 220 | ||
3.3 Oxygen bonding strength, mobility and reactivity | 221 | ||
4 Catalytic properties in reactions of biogas and biofuels transformation into syngas: effect of the nature of metal nanoparticles and support, metal-support interaction and oxygen mobility/reactivity on activity, kinetic features and stability to coking | 223 | ||
4.1 Ceria-zirconia mixed oxides | 223 | ||
4.2 Perovskite-like active components | 225 | ||
4.2.1. Dry reforming | 225 | ||
4.2.2 Oxygenates reforming | 226 | ||
4.2.2.1 Bulk perovskites | 226 | ||
4.2.2.2 Supported perovskites | 227 | ||
4.3 Spinel-based catalysts | 227 | ||
4.4 Schemes of mechanisms of basic reactions | 228 | ||
4.4.1 Methane partial oxidation and dry reforming | 228 | ||
4.4.2 Ethanol steam reforming and partial oxidation | 228 | ||
5 Design and performance of structured catalysts in the processes of biofuels transformation into syngas and water gas shift at short contact times | 228 | ||
6 Design and performance of catalytic membranes in biofuels selective oxidation/autothermal reforming into syngas | 230 | ||
6.1 Introduction into catalytic membrane reactors | 230 | ||
6.2 Oxygen separation membranes | 231 | ||
6.2.1 Methane partial oxidation | 231 | ||
6.2.2 Methane oxi-dry reforming | 232 | ||
6.3 Hydrogen separation membranes | 232 | ||
7 Mathematical modeling | 234 | ||
Acknowledgments | 236 | ||
References | 236 | ||
In situ and operando analysis of environmental catalysts - studies on reaction mechanism and active site | 242 | ||
1 Introduction | 242 | ||
2 X-ray adsorption | 243 | ||
2.1 Supported metal nanoparticle catalysts | 243 | ||
2.2 Metal oxides | 247 | ||
2.3 Metal-ion-exchanged zeolite catalysts | 247 | ||
3 Infrared spectroscopy | 248 | ||
4 UV-Vis spectroscopy | 253 | ||
5 Raman scattering spectroscopy | 258 | ||
6 Conclusions | 261 | ||
Acknowledgments | 261 | ||
References | 261 | ||
Understanding catalysis for processing glycerol and glycerol-based derivatives for the production of value added chemicals | 267 | ||
1 Introduction | 267 | ||
1.1 The biodiesel manufacturing process and glycerol by-product | 268 | ||
1.2 Crude glycerol composition | 269 | ||
1.3 Glycerol roduction and valorisation | 270 | ||
2 Biological glycerol transformations | 271 | ||
3 Conventional catalytic conversion of glycerol | 271 | ||
3.1 Oxidation | 271 | ||
3.2 Etherification and esterification | 271 | ||
3.3 Hydrogenolysis and dehydroxylation | 272 | ||
3.4 Reforming | 274 | ||
3.4.1 Steam reforming for hydrogen production | 274 | ||
3.4.2 Reforming for synthesis gas production | 275 | ||
3.5 Selective oligomerisation and polymerisation | 276 | ||
3.6 Dehydration | 276 | ||
4 Catalytic conversion of glycerol derivatives | 278 | ||
4.1 Acrolein to allyl alcohol | 278 | ||
4.1.1 Direct conversion of glycerol to allyl alcohol | 281 | ||
4.2 Glycidol | 283 | ||
4.2.1 Pathways via carbonate intermediates | 283 | ||
4.2.2 Pathways via urea | 283 | ||
4.2.3 Pathways via allyl alcohol | 285 | ||
4.3 Epichlorohydrin | 285 | ||
4.4 Glycidyl nitrate | 287 | ||
5 Conclusion and outlook | 289 | ||
References | 289 | ||
Combustion synthesis: a novel method of catalyst preparation | 297 | ||
1 Introduction to combustion synthesis | 297 | ||
1.1 Solution combustion synthesis: general definitions and classifications | 298 | ||
2 Mechanistic considerations in catalyst preparation by combustion synthesis | 303 | ||
2.1 Decomposition of metal nitrates | 304 | ||
2.2 Decomposition of glycine | 306 | ||
2.3 TGA analysis of metal nitrate and glycine combined | 308 | ||
3 Catalytic applications | 310 | ||
3.1 Applications to catalytic hydrogen generation from methanol | 310 | ||
3.2 Hydrogen generation from ethanol | 314 | ||
3.2.1 Unsupported multicomponent NiCu-Fe catalysts | 314 | ||
3.2.2 Ethanol decomposition on Ni supported on alumina catalysts pellets | 322 | ||
3.2.3 Ni supported on fumed silica catalysts | 324 | ||
3.2.4 Ni-Cu supported on Ceria and Silica | 328 | ||
3.3 Applications in fuels cells and electrocatalysis | 334 | ||
3.3.1 Direct alcohol fuel cells | 334 | ||
3.3.2 Proton exchange fuel cells (oxygen reduction reaction) | 337 | ||
Acknowledgments | 341 | ||
References | 342 |