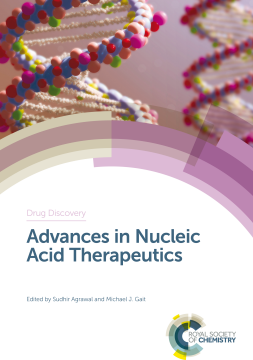
Additional Information
Book Details
Abstract
The sequencing of the human genome and subsequent elucidation of the molecular pathways that are important in the pathology of disease have provided unprecedented opportunities for the development of new therapeutics. Nucleic acid-based drugs have emerged in recent years to yield extremely promising candidates for drug therapy to a wide range of diseases. Advances in Nucleic Acid Therapeutics is a comprehensive review of the latest advances in the field, covering the background of the development of nucleic acids for therapeutic purposes to the array of drug development approaches currently being pursued using antisense, RNAi, aptamer, immune modulatory and other synthetic oligonucleotides. Nucleic acid therapeutics is a field that has been continually innovating to meet the challenges of drug discovery and development; bringing contributions together from leaders at the forefront of progress, this book depicts the many approaches currently being pursued in both academia and industry. A go-to volume for medicinal chemists, Advances in Nucleic Acid Therapeutics provides a broad overview of techniques of contemporary interest in drug discovery.
Table of Contents
Section Title | Page | Action | Price |
---|---|---|---|
Cover | Cover | ||
Advances in Nucleic Acid Therapeutics | i | ||
Foreword | vii | ||
Preface | ix | ||
Contents | xiii | ||
Chapter 1 - History and Development of Nucleotide Analogues in Nucleic Acids Drugs | 1 | ||
1.1 Introduction | 1 | ||
1.2 The Antisense Concept | 2 | ||
1.3 Developments in Oligonucleotide Synthesis | 2 | ||
1.4 Choices for Antisense Oligodeoxynucleotide Modifications | 3 | ||
1.4.1 Backbone Modifications | 3 | ||
1.4.1.1 Phosphorothioates | 4 | ||
1.4.1.2 Non-anionic Internucleotide Linkages | 7 | ||
1.4.1.2.1\rPhosphorodiamidate Morpholino Oligonucleotodes.Phosphorodiamidate morpholino oligonucleotides (PMO) are charge-neutral oligonucl... | 8 | ||
1.4.1.2.2\rPeptide Nucleic Acids.Peptide nucleic acids (PNA) are charge-neutral synthetic mimics of DNA or RNA containing N-2 aminoethylgly... | 9 | ||
1.4.2 Heterocyclic Bases | 9 | ||
1.4.3 Sugar Modifications | 10 | ||
1.4.3.1 Oligoribonucleotides | 10 | ||
1.4.3.2 2ʹ-O-Alkyl Sugars | 10 | ||
1.4.3.3 Bridged Nucleic Acids | 11 | ||
1.5 Gapmers Using Combinations of Modified Oligodeoxy and/or Oligoribonucleotides | 12 | ||
1.6 Antisense Conjugates | 13 | ||
1.7 The Role of Innate Immune Receptors in Nucleic Acid Therapeutics | 14 | ||
1.8 Future Directions | 15 | ||
Acknowledgements | 16 | ||
References | 17 | ||
Chapter 2 - Mechanisms of Antisense Oligonucleotides | 22 | ||
2.1 Introduction | 22 | ||
2.2 RNase H and ASO Action | 23 | ||
2.3 ASOs and Regulation of Splicing | 25 | ||
2.4 ASOs and Activation of Frataxin, a Case Study for an Emerging Mechanism | 27 | ||
2.5 Summary | 29 | ||
Acknowledgements | 29 | ||
References | 29 | ||
Chapter 3 - The Medicinal Chemistry of RNase H-activating Antisense Oligonucleotides | 32 | ||
3.1 Introduction to Gapmers | 32 | ||
3.2 Human RNase H1 | 33 | ||
3.2.1 Biochemistry of Human RNase H1 | 33 | ||
3.2.2 Structural Biology of Human RNase H1 | 34 | ||
3.3 Structure–Activity Relationships of Gap Modifications | 36 | ||
3.3.1 Phosphorothioate (PS) DNA | 38 | ||
3.3.2 Chiral PS DNA | 39 | ||
3.3.3 Methyl Phosphonates, Phosphoramidates, Phosphotriesters and Boranophosphonate DNA | 40 | ||
3.3.4 2ʹ-Fluoro Arabino Nucleic Acids (FANA) | 40 | ||
3.3.5 DNA-like Modifications | 41 | ||
3.4 SAR of Wing Modifications | 41 | ||
3.4.1 2ʹ-O-Methoxyethyl RNA (MOE) | 42 | ||
3.4.2 Locked Nucleic Acids (LNA) and Constrained Ethyl 2ʹ-4ʹ-Bridged Nucleic Acids (cEt) | 42 | ||
3.4.3 α-l-LNA and Related Analogs | 43 | ||
3.4.4 F-HNA and F-CeNA | 43 | ||
3.4.5 Tricyclo DNA (tcDNA) | 44 | ||
3.4.6 Phosphorodiamidate Linked Morpholinos (PMOs) | 44 | ||
3.5 Design of Gapmer ASOs | 45 | ||
3.5.1 MOE Gapmer ASOs | 45 | ||
3.5.2 LNA Gapmers | 46 | ||
3.5.3 cEt Gapmers | 47 | ||
3.5.4 ASO Gapmer Duplexes | 47 | ||
3.6 Control of Protein Binding | 47 | ||
3.6.1 Interaction of Gapmer ASOs with Plasma Proteins | 48 | ||
3.6.2 Interaction of Gapmer-ASOs with Cell-surface Proteins | 48 | ||
3.6.3 Targeting Cell-surface Proteins for Cell-specific Delivery of Gapmers | 49 | ||
3.6.4 Avoiding Interactions with TLR Receptors to Avoid Immune-stimulatory Toxicities | 50 | ||
3.6.5 Optimizing Intracellular Distribution | 51 | ||
3.7 Conclusions | 51 | ||
References | 52 | ||
Chapter 4 - Antisense Technology: Liver Targeting and Beyond for Drug Discovery | 62 | ||
4.1 Introduction | 62 | ||
4.2 Liver Targeting | 64 | ||
4.2.1 The Beginning | 64 | ||
4.2.2 Broadening the Liver-targeting Pipeline | 66 | ||
4.3 Innovations in Liver Targeting | 67 | ||
4.4 Beyond the Liver | 70 | ||
4.5 Conclusions | 72 | ||
References | 73 | ||
Chapter 5 - Oligonucleotide-based Toll-like Receptor Antagonists and Therapeutic Applications | 80 | ||
5.1 Introduction | 80 | ||
5.2 Oligonucleotide-based TLR Antagonists | 82 | ||
5.2.1 Structure–Activity Relationship Studies | 83 | ||
5.2.2 TLR Antagonists | 83 | ||
5.2.3 Inhibitory Activity of TLR Antagonists | 85 | ||
5.2.4 Inhibitory Activity of Clinical Candidates | 85 | ||
5.3 Studies of TLR Antagonists in Disease Models | 87 | ||
5.3.1 Psoriasis | 87 | ||
5.3.2 Systemic Lupus Erythematosus (SLE) | 88 | ||
5.3.3 Rheumatoid Arthritis (RA) | 89 | ||
5.3.4 Duchenne Muscular Dystrophy (DMD) | 90 | ||
5.3.5 MyD88 L265P-positive B Cell Lymphoma (BCL) | 90 | ||
5.3.6 Restenosis and Atherosclerosis | 91 | ||
5.3.7 Inflammatory Bowel Disease (IBD) | 92 | ||
5.3.8 HIV-1 | 92 | ||
5.4 Clinical Development of Lead TLR Antagonist Candidates | 93 | ||
5.4.1 Moderate-to-severe Plaque Psoriasis | 93 | ||
5.4.2 Waldenström's Macroglobulinemia | 94 | ||
5.5 Conclusions | 96 | ||
Acknowledgements | 96 | ||
References | 96 | ||
Chapter 6 - Splicing Modulation for Therapeutics | 103 | ||
6.1 Introduction | 103 | ||
6.1.1 RNA Splicing | 103 | ||
6.1.1.1 Splicing Machinery and Sequence Motifs Required for RNA Splicing | 104 | ||
6.1.1.2 Other Sequence Motifs that Influence RNA Splicing | 104 | ||
6.1.2 Constitutive Splicing | 106 | ||
6.1.3 Alternative Splicing | 106 | ||
6.1.4 Cryptic Splicing | 108 | ||
6.2 Therapeutic Exon Skipping Options | 108 | ||
6.2.1 Antisense Oligonucleotides (ASOs) and Chemical Modifications | 108 | ||
6.2.2 Restoring Cryptic Splicing | 109 | ||
6.2.2.1 β-Thalassemia | 109 | ||
6.2.2.2 Ataxia Telangiectasia | 110 | ||
6.2.3 Reading Frame Restoration | 111 | ||
6.2.3.1 Duchenne Muscular Dystrophy | 111 | ||
6.2.3.2 Dystrophic Epidermolysis Bullosa | 115 | ||
6.2.4 Exon Inclusion | 116 | ||
6.2.4.1 Spinal Muscular Atrophy | 116 | ||
6.2.4.2 Glycogen Storage Disease Type II or Pompe Disease | 118 | ||
6.2.5 Generating Less Protein or Non-toxic Protein | 118 | ||
6.2.5.1 Hutchinson–Gilford Progeria Syndrome | 118 | ||
6.2.5.2 Huntington Disease | 119 | ||
6.3 Future Perspectives, Towards Additional Approved Splice-modulating ASOs | 120 | ||
6.3.1 The Challenge of Personalized Medicine Development | 120 | ||
6.3.2 ASO Delivery | 121 | ||
6.3.3 Future Perspective | 121 | ||
References | 122 | ||
Chapter 7 - Targeting Toxic Repeats | 126 | ||
7.1 Introduction | 126 | ||
7.2 Expanded Repetitive Sequences and Human Disease | 127 | ||
7.2.1 Repeat Instability | 127 | ||
7.2.2 Molecular Mechanisms of Disease | 128 | ||
7.2.2.1 Loss-Of-Function | 129 | ||
7.2.2.2 Gain-Of-Function | 130 | ||
7.3 Why are Expanded Repeats so Special as Therapeutic Targets | 130 | ||
7.3.1 Structures Formed by Expanded Repeats | 131 | ||
7.3.1.1 R-Loop | 131 | ||
7.3.1.2 Triple Helix | 131 | ||
7.3.1.3 Imperfect Hairpin | 131 | ||
7.3.1.4 Quadruplexes | 133 | ||
7.3.2 Structures of Repeat-Expanded Transcripts | 133 | ||
7.3.3 Structural Implications for Therapeutic Targeting | 134 | ||
7.4 Therapeutics to Target Expanded Repeats | 135 | ||
7.4.1 Therapeutic Strategies | 135 | ||
7.4.2 Small Molecules Targeting Repeat Structures | 136 | ||
7.4.3 Antisense Strategies | 138 | ||
7.4.3.1 ASOs Directed Against DNA | 138 | ||
7.4.3.2 ASOs Directed Against RNA | 139 | ||
7.4.3.3 RNA Interference | 140 | ||
7.4.3.4 Other Antisense Strategies | 141 | ||
7.4.4 CRISPR/Cas9, TALEN, ZFN and Other Protein Effectors | 141 | ||
7.5 Challenges for Multisystemic Repeat Diseases | 142 | ||
Abbreviations | 143 | ||
Acknowledgements | 144 | ||
References | 144 | ||
Chapter 8 - Research and Development of Oligonucleotides Targeting MicroRNAs (miRNAs) | 151 | ||
8.1 Introduction: MicroRNA Biogenesis and Functions | 151 | ||
8.2 miRNAs as Targets for Drugs | 154 | ||
8.3 AntimiR Oligonucleotides as Drugs | 154 | ||
8.3.1 The Development of AntimiR Medicinal Chemistry | 154 | ||
8.3.1.1 2ʹ-O-Methyl-modified AntimiRs | 155 | ||
8.3.1.2 Antagomirs | 157 | ||
8.3.1.3 2ʹ-O-Methoxyethyl AntimiRs | 158 | ||
8.3.1.4 2ʹ-Fluoro-modified AntimiRs | 158 | ||
8.3.1.5 Locked Nucleic Acids and Constrained Ethyl Derivatives | 159 | ||
8.3.1.6 Peptide Nucleic Acids | 161 | ||
8.3.1.7 Morpholino Oligonucleotides | 162 | ||
8.3.2 New Insights into Mechanisms of Oligonucleotide-based miRNA Targeting | 162 | ||
8.3.2.1 AntimiR-mediated Tailing and Trimming of miRNAs | 163 | ||
8.3.2.2 Targeting miRNAs in the RISC | 163 | ||
8.3.2.3 AntimiR-mediated Unloading of miRNAs from RISC | 164 | ||
8.3.3 New Chemistries and Alternative Approaches for Targeting miRNAs | 165 | ||
8.4 AntimiRs in Clinical Trials | 167 | ||
8.4.1 AntimiRs Targeting miR-122 for Treatment of HCV | 167 | ||
8.4.2 AntimiR-targeting of Other miRNAs in Clinical Studies | 169 | ||
8.5 Conclusions | 170 | ||
Acknowledgement | 171 | ||
References | 171 | ||
Chapter 9 - Oligonucleotide Targeting of Long Non-coding RNAs | 181 | ||
9.1 Introduction | 181 | ||
9.2 History of lncRNAs | 182 | ||
9.3 Biology and Functions of lncRNA | 185 | ||
9.3.1 lncRNAs as Regulators of Transcription | 186 | ||
9.3.2 lncRNA as Regulators of Post-transcriptional Processing | 187 | ||
9.3.2.1 Splicing | 188 | ||
9.3.2.2 Polyadenylation | 188 | ||
9.3.2.3 mRNA Stability | 188 | ||
9.3.2.4 Competing Endogenous RNAs | 188 | ||
9.3.2.5 Interactions with miRs | 189 | ||
9.3.2.6 Endogenous siRNA and miRNA Generation | 189 | ||
9.3.3 lncRNA as Regulators of Translation | 189 | ||
9.4 Classification of lncRNA | 190 | ||
9.4.1 Functional Classification | 191 | ||
9.4.2 Genomic Classification | 191 | ||
9.5 Targeting of Long Non-coding RNA by Oligonucleotides | 192 | ||
9.5.1 Antisense Oligonucleotides | 192 | ||
9.5.2 siRNAs | 192 | ||
9.5.3 CRISPR and Other Approaches | 193 | ||
9.6 Therapeutic Applications | 194 | ||
9.6.1 Neurology and Psychiatry | 194 | ||
9.6.1.1 Dravet's Syndrome (DS) | 194 | ||
9.6.1.2 Angelman's Syndrome (AS) | 195 | ||
9.6.1.3 Spinal Muscular Atrophy (SMA) | 195 | ||
9.6.1.4 Alzheimer's Disease (AD) | 196 | ||
9.6.1.5 Fragile X Mental Retardation 1 (FMR1) | 197 | ||
9.6.1.6 Psychiatric Diseases | 197 | ||
9.6.2 Oncology | 198 | ||
9.6.3 Cardiology | 201 | ||
9.6.4 Gastroenterology | 202 | ||
9.7 Perspectives | 202 | ||
References | 203 | ||
Chapter 10 - Conjugate-mediated Delivery of RNAi-based Therapeutics: Enhancing Pharmacokinetics–Pharmacodynamics Relationships of Medicinal Oligonucleotides | 206 | ||
10.1 Introduction | 206 | ||
10.2 Chemical Stabilization as a Prerequisite for Conjugate-mediated Delivery of siRNAs: Effects on Clearance, Distribution and S... | 207 | ||
10.3 Modulating Biodistribution of Therapeutic Oligonucleotides Using Conjugated Modalities: Targeted versus Broad Functional Del... | 213 | ||
10.3.1 Broad Functional Delivery of Conjugated siRNAs | 213 | ||
10.3.2 Targeted Delivery of Conjugated siRNAs | 216 | ||
10.4 Productive Delivery of Therapeutic Oligonucleotides: Overcoming the Endosomal Barrier | 218 | ||
10.5 The Effects of the Route of Administration: Local versus Systemic Delivery | 219 | ||
10.5.1 Local Delivery of Conjugated siRNAs | 219 | ||
10.5.2 Systemic Delivery of Conjugated siRNAs | 221 | ||
10.6 Enhancing PK Properties of Conjugated siRNAs: Reducing Clearance Kinetics and Accelerating Target Tissue Uptake | 223 | ||
10.7 Conjugation Chemistry for RNAi-based Therapeutics: Future Perspectives | 224 | ||
Acknowledgements | 226 | ||
References | 226 | ||
Chapter 11 - Liver-targeted RNAi Therapeutics: Principles and Applications | 233 | ||
11.1 Introduction | 233 | ||
11.2 The Role of Chemistry | 234 | ||
11.3 Liver-specific Delivery of siRNA | 237 | ||
11.3.1 Ionizable Lipid Nanoparticles (iLNPs) | 238 | ||
11.3.2 Lipid-conjugated siRNA provided Proof of Concept for RNAi Therapeutics | 239 | ||
11.3.3 Discovery of GalNAc Conjugates | 240 | ||
11.4 Clinical Candidates | 240 | ||
11.4.1 ONPATTROTM (Patisiran) | 240 | ||
11.4.2 Inclisiran | 254 | ||
11.4.3 Givosiran | 255 | ||
11.4.4 Fitusiran | 256 | ||
11.4.5 TTRsc02 | 256 | ||
11.4.6 Revusiran | 256 | ||
11.5 Conclusions and Outlook | 257 | ||
References | 257 | ||
Chapter 12 - Advances and Challenges of RNAi-Based Anti-HIV Therapeutics | 266 | ||
12.1 Introduction | 266 | ||
12.2 Potential Targets for Anti-HIV-1 RNAi Therapeutics | 268 | ||
12.2.1 Targeting the HIV-1 Viral Genome | 268 | ||
12.2.2 Targeting Host Factors | 270 | ||
12.3 Challenges in Obtaining Effective Anti-HIV-1 RNAi Activity | 272 | ||
12.3.1 Key Barriers to In Vivo RNAi Efficacy | 272 | ||
12.3.2 The Need for Rational Design and for Chemical Modifications | 274 | ||
12.3.3 The Need for Combinatorial RNAi | 275 | ||
12.4 Recent Progress and Clinical Development of Anti-HIV-1 RNAi | 276 | ||
12.4.1 In Vivo Delivery of Anti-HIV-1 RNAi Effectors | 276 | ||
12.4.2 Ex vivo Delivery of Anti-HIV-1 shRNAs | 281 | ||
12.4.3 Clinical Development of Anti-HIV-1 RNAi | 282 | ||
12.5 Conclusions and Perspective | 283 | ||
Conflict of Interest Declaration | 284 | ||
Acknowledgements | 285 | ||
References | 285 | ||
Chapter 13 - Nucleic Acid Innate Immune Receptors | 292 | ||
13.1 Introduction | 292 | ||
13.2 Toll-like Receptors | 293 | ||
13.2.1 TLR3 Recognizes dsRNA | 293 | ||
13.2.2 TLR7 and TLR8 Recognize ssRNA and Guanosine or Uridine | 293 | ||
13.2.3 TLR9 Recognizes CpG-DNA | 294 | ||
13.2.4 Chaperones Regulate the Maturation of NA-sensing TLRs | 295 | ||
13.2.5 Unc93B1 Regulates the Balance of TLR7 and TLR9 Responses | 296 | ||
13.2.6 Proteolytic Cleavage of NA-sensing TLRs is Essential for Their Function | 296 | ||
13.2.7 Trafficking of TLR7 and TLR9 is Essential for Type I Interferon Production in pDCs | 297 | ||
13.3 Nucleic Acids Sensing in the Cytoplasm | 298 | ||
13.3.1 Cytosolic DNA Sensors Recognize dsDNA | 298 | ||
13.3.2 RIG-I and MDA5 Recognize dsRNA and Activate MAVS to Induce Immune Responses | 299 | ||
13.4 Conclusions | 299 | ||
References | 300 | ||
Chapter 14 - Synthetic Agonists of Toll-like Receptors and Therapeutic Applications | 306 | ||
14.1 Introduction | 306 | ||
14.1.1 RIG-I-like Receptors | 307 | ||
14.1.2 AIM2-like Receptors (ALRs) | 308 | ||
14.1.3 NOD-like Receptors, NLRP3 Inflammasome | 308 | ||
14.1.4 Cyclic GMP–AMP Synthase (cGAS) and the STING Pathway | 309 | ||
14.1.5 Toll-like Receptors (TLR) | 309 | ||
14.2 Agonists of TLR3 | 310 | ||
14.2.1 Synthetic Agonists of TLR3 | 310 | ||
14.3 Agonists of TLR 7 and TLR 8 | 311 | ||
14.3.1 Synthetic Agonists of TLR7 and TLR8 | 312 | ||
14.3.2 Preclinical Studies of Agonists of TLR7 and TLR8 | 314 | ||
14.4 Agonists of TLR9 | 315 | ||
14.4.1 Synthetic Agonists of TLR9 | 316 | ||
14.4.1.1 Role of the 5ʹ-end | 317 | ||
14.4.1.2 Synthetic Immune-stimulatory Motifs | 319 | ||
14.4.1.3 Secondary Structures | 320 | ||
14.5 Therapeutic Applications of Synthetic Agonists of TLR9 | 322 | ||
14.5.1 Preclinical Studies in Cancer | 322 | ||
14.5.2 Treatment for Asthma and Allergies | 324 | ||
14.5.3 Use as Vaccine Adjuvants | 325 | ||
14.6 Clinical Development of Synthetic Agonists of TLR9 | 325 | ||
14.6.1 Clinical Trials in Hepatitis C Patients | 325 | ||
14.6.2 Clinical Trials in Cancer | 326 | ||
14.7 Conclusions | 327 | ||
References | 328 | ||
Chapter 15 - Prostate-specific Membrane Antigen (PSMA) Aptamers for Prostate Cancer Imaging and Therapy | 339 | ||
15.1 Introduction | 339 | ||
15.1.1 Aptamers and SELEX | 339 | ||
15.1.2 DNA vs. RNA Aptamers | 342 | ||
15.2 Prostate Specific Membrane Antigen (PSMA) Aptamers | 343 | ||
15.2.1 PSMA | 343 | ||
15.2.2 DNA and RNA PSMA Aptamers | 345 | ||
15.2.2.1 PSMA RNA Aptamers | 345 | ||
15.2.2.2 PSMA DNA Aptamers | 347 | ||
15.3 PSMA Aptamers Applications | 348 | ||
15.3.1 Imaging and Diagnostic Applications | 348 | ||
15.3.1.1 Radio-imaging Applications | 348 | ||
15.3.1.2 Computed Tomography (CT) Applications | 349 | ||
15.3.1.3 Magnetic Resonance Imaging (MRI) Applications | 349 | ||
15.3.1.4 Optical Imaging Applications | 350 | ||
15.3.2 PSMA Aptamers as Therapeutic Inhibitors | 351 | ||
15.3.3 Targeted Delivery Applications | 351 | ||
15.3.3.1 PSMA Aptamer RNAi Conjugates | 351 | ||
15.3.3.2 PSMA Aptamer–CRISPR Conjugates | 355 | ||
15.3.3.3 Bispecific PSMA Aptamer Conjugates | 356 | ||
15.3.3.4 PSMA Aptamer Protein Toxin Conjugates | 356 | ||
15.3.3.5 PSMA Aptamer Small-molecule Conjugates | 357 | ||
15.3.3.6 PSMA Aptamer Functionalized Nanoparticles | 357 | ||
15.4 Conclusions | 359 | ||
15.5 Future Perspectives | 359 | ||
Acknowledgements | 361 | ||
References | 361 | ||
Chapter 16 - Aptamers and Clinical Applications | 367 | ||
16.1 Introduction | 367 | ||
16.2 Recent Preclinical Studies of Aptamer Drugs | 369 | ||
16.2.1 Aptamer Structures | 369 | ||
16.2.1.1 SOMAmers and X-Aptamers | 369 | ||
16.2.1.2 Thioaptamers | 373 | ||
16.2.1.3 Photo-regulated Aptamers | 374 | ||
16.2.2 Non-ocular Diseases | 376 | ||
16.2.2.1 Cardiovascular and Cerebrovascular Diseases | 376 | ||
16.2.2.2 Alzheimer's Disease | 377 | ||
16.2.2.3 Antiviral Applications | 378 | ||
16.2.2.4 Antibacterial Applications | 380 | ||
16.2.2.5 Antiparasitic Applications | 381 | ||
16.3 Recent Studies of Aptamer-based Targeting of Drugs | 381 | ||
16.4 Clinical Studies of Aptamer Drugs Registered in ClinicalTrials.gov | 384 | ||
16.4.1 Completed Clinical Studies | 385 | ||
16.4.2 Terminated or Withdrawn Studies | 390 | ||
16.4.3 Active Studies | 391 | ||
16.5 Conclusions and Prospects | 392 | ||
References | 394 | ||
Chapter 17 - CRISPR-based Technologies for Genome Engineering: Properties, Current Improvements and Applications in Medicine | 400 | ||
17.1 Introduction | 400 | ||
17.2 Sequence-specific CRISPR Nucleases and Improved Variants | 401 | ||
17.2.1 Cas 9 from Streptococcus pyogenes, Orthologues and Variants | 401 | ||
17.2.1.1 CRISPR Systems from the Bacterial Immune Response | 401 | ||
17.2.1.2 Variation Around the SpCas9–sgRNA Complex to Improve Activity | 403 | ||
17.2.1.3 Expanding the Target Repertoire | 404 | ||
17.2.2 Expanding Targeted Functions with “CRISPR Fusions” | 404 | ||
17.2.2.1 Catalytically Dead CRISPR Systems | 404 | ||
17.2.2.2 Multiplexing Strategies | 405 | ||
17.2.3 Specificity of CRISPR Systems | 405 | ||
17.2.3.1 Approaches to Determine Specificity | 406 | ||
17.2.3.2 Computational Tools for Guide RNA Selection | 406 | ||
17.2.3.3 Approaches to Improve Specificity | 407 | ||
17.3 Genome Editing Mechanisms and Current Improvements | 408 | ||
17.3.1 Principles: Genome Editing Takes Place During Repair of DSB Breaks Induced by Cas9 | 408 | ||
17.3.1.1 End-joining Pathways | 409 | ||
17.3.1.2 Homology-directed Repair | 409 | ||
17.3.1.3 Regulation of DNA Repair Pathways During the Cell Cycle | 410 | ||
17.3.2 Improvement of HDR-based CRISPR Strategies for Programmable Genome Modification | 410 | ||
17.3.2.1 Strategies to Repress the NHEJ-mediated Repair | 410 | ||
17.3.2.2 Strategies to Enhance HDR | 412 | ||
17.3.2.2.1\rMain Pathways in HDR-mediated DSB Repair.In HDR-mediated repair, the DSB ends are resected to expose 3ʹ ssDNA tails. Resection s... | 412 | ||
17.3.2.2.2\rDNA Homology Template Donors for HDR-mediated Genome Editing.In HDR-mediated genome editing, cells accept different types of DNA... | 412 | ||
17.3.2.2.3\rFusion of Cas9 to HDR Partners.Several approaches to enhance HDR include the fusion to Cas9 of another protein thereby combined ... | 413 | ||
17.3.2.2.4\rModifications of the Cell Cycle During Gene Editing and Other Approaches.Orthwein et al. showed that the replacement of partner ... | 413 | ||
17.4 Epigenome Editing and Base Editing with the CRISPR Systems | 414 | ||
17.4.1 Modulation of Transcription, CRISPRa and CRISPRi Systems | 414 | ||
17.4.2 Modulation of Chromatin Status | 416 | ||
17.4.3 Base Editors | 417 | ||
17.5 Applications in Medicine and Challenges | 419 | ||
17.5.1 Applications in Biomedical Research | 419 | ||
17.5.2 The Delivery Challenge | 420 | ||
17.5.3 The Genome Editing Precision Challenge | 422 | ||
17.5.4 Clinical Trials Based on Genome Editing | 422 | ||
17.6 Conclusions | 423 | ||
References | 424 | ||
Chapter 18 - Therapeutic Potential of Ribozymes | 434 | ||
18.1 Introduction | 434 | ||
18.2 Trans-cleaving Ribozymes | 435 | ||
18.3 Ribozyme-mediated Genetic Repair | 439 | ||
18.3.1 Spliceosome-mediated RNA Trans-splicing (SMaRT) | 440 | ||
18.3.2 Group I Intron Ribozyme-mediated Trans-splicing | 441 | ||
18.3.3 Twin Ribozyme-mediated RNA Repair | 443 | ||
18.3.4 Correction of Genetic Disorders by Retro-homing Group II Introns | 445 | ||
18.4 Conclusions | 445 | ||
References | 447 | ||
Chapter 19 - Large-scale Automated Synthesis of Therapeutic Oligonucleotides: A Status Update | 453 | ||
19.1 Introduction | 453 | ||
19.2 Chemical Modifications in Clinical and Commercial Products | 454 | ||
19.2.1 First-generation Backbone Modifications – the Phosphorothioate (PS) Internucleotide Linkage | 454 | ||
19.2.2 Second-generation Sugar Modifications | 455 | ||
19.3 The Oligonucleotide Manufacturing Process | 457 | ||
19.3.1 Use of an Automated Synthesizer | 457 | ||
19.3.2 Starting Materials | 458 | ||
19.3.2.1 Nucleoside-loaded Solid Support | 458 | ||
19.3.2.2 Nucleoside Phosphoramidites | 460 | ||
19.3.2.3 Sulfur-transfer Reagents | 462 | ||
19.3.3 Reagent-related Impurities | 462 | ||
19.3.4 The Four-step Synthesis Cycle | 464 | ||
19.3.5 Cleavage and Deprotection | 465 | ||
19.3.6 The Purification Process | 466 | ||
19.3.7 The Lyophilization Process | 468 | ||
19.4 Analytical Protocols | 468 | ||
19.5 Synthesis Yield and Product Purity | 470 | ||
19.6 Conclusions and Future Outlook | 470 | ||
References | 471 | ||
Chapter 20 - Preclinical and Clinical Drug-metabolism, Pharmacokinetics and Safety of Therapeutic Oligonucleotides | 474 | ||
20.1 Introduction | 474 | ||
20.2 Oligonucleotide Chemistries and Mode of Action (MOA) | 475 | ||
20.2.1 Chemistry and Design Considerations of Therapeutic ONDs | 475 | ||
20.2.1.1 OND Chemistry | 476 | ||
20.2.1.2 OND Design | 476 | ||
20.2.2 Delivery Approaches | 478 | ||
20.3 Distribution, Metabolism and Pharmacokinetics (DMPK) | 479 | ||
20.3.1 DMPK Properties of ONDs | 479 | ||
20.3.1.1 Plasma and Tissue Pharmacokinetics | 479 | ||
Anti-drug Antibodies.Increasing experience with longer-term treatment duration of PS gapmer and non-gapmer ASOs in animal studie... | 481 | ||
20.3.1.2 Tissue Distribution | 481 | ||
20.3.1.3 Productive Uptake | 482 | ||
20.3.1.4 Metabolism | 484 | ||
20.3.1.5 Excretion | 485 | ||
20.3.2 Delivery Strategies | 485 | ||
20.3.2.1 Local Delivery | 486 | ||
20.3.2.2 Advanced Formulation Approaches | 486 | ||
20.3.2.3 Targeted Delivery | 487 | ||
20.4 Class Profile of Toxicity | 488 | ||
20.5 Hybridization-dependent Toxicities | 490 | ||
20.5.1 On-target Safety and Exaggerated Pharmacology | 490 | ||
20.5.2 Off-target Pharmacology | 491 | ||
20.6 Hybridization-independent Toxicities | 493 | ||
20.6.1 Effects Related to Transient Protein Binding | 494 | ||
20.6.1.1 Inhibition of the Coagulation Cascade | 494 | ||
20.6.1.2 Complement System Activation | 494 | ||
20.6.2 Immune-mediated Effects | 496 | ||
20.6.2.1 Pro-inflammatory Effects | 496 | ||
20.6.2.1.1\rManifestations of Immune Stimulation.The manifestations of immune stimulation by DNA- and RNA-based ONDs have been described in ... | 496 | ||
20.6.2.1.2\rPro-inflammatory Mechanisms.The pro-inflammatory properties of non-formulated ONDs stem from the activation of the innate immune... | 498 | ||
20.6.2.1.3\rMitigating Proinflammatory Effects.Chemical modifications can modify the immune-stimulatory potential of ONDs of a given sequenc... | 499 | ||
20.6.2.1.4\rImmunogenicity – Anti-drug Antibodies (ADAs).PS backbone ONDs were long considered to be devoid of antigenic properties based on... | 500 | ||
20.6.2.2 Thrombocytopenia | 500 | ||
20.6.3 Toxicity in High-exposure Organs | 505 | ||
20.6.3.1 Liver Toxicity | 505 | ||
20.6.3.1.1\rGalNAc-conjugation.At a given dose, GalNAc conjugation significantly increases hepatocyte uptake of both single-stranded and dou... | 506 | ||
20.6.3.1.2\rToxic Sequence Motifs.Important efforts have been made to better understand the sequence component and define particular sequenc... | 507 | ||
20.6.3.1.3\rMechanistic Hypotheses.The understanding of the mechanism involved in the high-affinity gapmer hepatotoxicity has greatly evolve... | 507 | ||
20.6.3.2 Kidney Toxicity | 509 | ||
20.6.3.2.1\rTubular Effects.The morphological and functional tubular changes in animal studies follow a predictable course, whereby initial ... | 509 | ||
20.6.3.2.2\rGlomerular Effects.Glomerular lesions are not commonly observed with PS ONDs and 2ʹMOE gapmers,234 but glomerulopathies were a r... | 511 | ||
20.7 Getting to the Clinic | 511 | ||
20.7.1 Regulatory Considerations for Preclinical Development | 511 | ||
20.7.2 Precision Medicine and Opportunity for Accelerated Timelines | 515 | ||
20.8 Conclusions | 515 | ||
Acknowledgements | 516 | ||
References | 517 | ||
Subject Index | 532 |