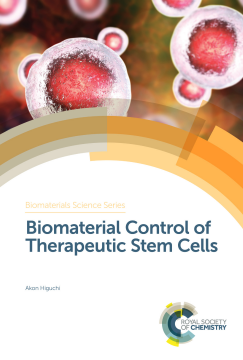
Additional Information
Book Details
Abstract
Human pluripotent stem cells (hPSCs), which cover both human embryonic stem cells (hESCs) and induced pluripotent stem cells (iPSCs), show promise for drug discovery and regenerative medicine applications. These stem cells cannot be cultured on conventional tissue culture dishes but on biomaterials that have specific interactions with the hPSCs. Differentiation is regulated by the biological and physical cues conferred by the biomaterial. This book provides a systematic treatment of these topics bridging the gap between fundamental biomaterials research of stem cells and their use in clinical trials.
The author looks at hPSC culture on a range of biomaterial substrates. Differentiation and control of hESCs and iPSCs into cardiomyocytes, osteoblasts, neural lineages and hepatocytes are covered. The author then considers their translation into stem cell therapies and looks at clinical trials across spinal cord injury, macular degeneration, bone disease and myocardial infarction. Finally, a chapter on future directions closes the book. By using this book, the reader will gain a robust overview of current research and a clearer understanding of the status of clinical trials for stem cell therapies.
Table of Contents
Section Title | Page | Action | Price |
---|---|---|---|
Cover | Cover | ||
Preface | v | ||
Abbreviations | vii | ||
Contents | xiii | ||
Chapter 1 Introduction | 1 | ||
1.1 Introduction | 1 | ||
1.2 Stem Cells | 1 | ||
1.3 The Extracellular Matrix | 4 | ||
1.4 hPSC Culture on Biomaterials | 4 | ||
1.5 hPSC Differentiation on Biomaterials | 5 | ||
1.6 Biomaterials Control hPS Cell Differentiation Fate | 6 | ||
1.7 Stem Cell Therapy Using Biomaterials | 7 | ||
References | 7 | ||
Chapter 2 Adult Stem Cell Culture on Extracellular Matrices and Natural Biopolymers | 12 | ||
2.1 Introduction | 12 | ||
2.2 Chemical and Biological Interactions of ECM Proteins and Stem Cells | 12 | ||
2.3 Collagen | 15 | ||
2.3.1 Collagen Type I Scaffold | 16 | ||
2.3.2 Organic Hybrid Scaffold Made of Collagen Type I | 23 | ||
2.3.3 Scaffolds Using Collagen Type II and Type III | 27 | ||
2.3.4 Hybrid Collagen Scaffold Using Inorganic Materials | 31 | ||
2.3.5 Collagen Scaffolds Immobilized Antibody Targeting Stem Cells | 33 | ||
2.3.6 Differentiation into Endoderm and Ectoderm Lineages Using Collagen Scaffolds | 34 | ||
2.4 Gelatin | 35 | ||
2.4.1 Gelatin Hydrogels and Scaffolds | 35 | ||
2.4.2 Gelatin Hybrid Scaffolds | 38 | ||
2.5 Laminin | 40 | ||
2.6 Fibronectin | 47 | ||
2.7 Vitronectin | 49 | ||
2.8 Fibrin | 51 | ||
2.9 Decellularized ECM | 52 | ||
2.10 Biomaterials with ECM-mimicking Oligopeptides | 58 | ||
2.10.1 MS Cell Differentiation on Self-assembled ECM-peptide Nanofibers | 59 | ||
2.10.2 Osteogenic Induction on ECM-peptide Immobilized Dishes and Scaffolds | 64 | ||
2.10.3 Chondrogenic Induction on ECM-peptide Immobilized Dishes and Scaffolds | 65 | ||
2.10.4 Neural Induction on ECM-peptide Immobilized Dishes and Scaffolds | 68 | ||
2.11 Biomaterials with N-Cadherin Mimicking Oligopeptides | 69 | ||
2.12 Conclusion and Future Perspective | 71 | ||
References | 71 | ||
Chapter 3 Feeder-free and Xeno-free Culture of Human Pluripotent Stem Cells on Biomaterials | 86 | ||
3.1 Introduction | 86 | ||
3.2 Analysis of the Pluripotency of hPS Cells | 86 | ||
3.3 2D Cultivation of hPS Cells on Biomaterials | 94 | ||
3.3.1 hPS Cell Cultivation on ECM-immobilized Surfaces in 2D | 94 | ||
3.3.1.1 FN (Fibronectin) | 95 | ||
3.3.1.2 LN (Laminin) | 97 | ||
3.3.1.3 VN (Vitronectin) | 97 | ||
3.3.2 hPS Cell Cultivation on Oligopeptide-immobilized Surfaces in 2D | 98 | ||
3.3.3 hPS Cell Cultivation on a Recombinant E-cadherin Surface in 2D | 103 | ||
3.3.4 hPS Cell Cultivation on Biomaterials Immobilized with Polysaccharide in 2D | 105 | ||
3.3.5 hPS Cell Cultivation on Synthetic Biomaterials in 2D | 109 | ||
3.3.5.1 Synthetic Polymeric Materials That Maintain the Long-term Cultivation of hPS Cells | 110 | ||
3.3.5.2 Thermoresponsive Polymeric Materials That Maintain the Long-term Cultivation of hPS Cells | 112 | ||
3.3.5.3 Synthetic Microfibrous Scaffolds That Maintain the Long-term Cultivation of hPS Cells | 118 | ||
3.4 Three-dimensional Cultivation of hPS Cells on Biomaterials | 119 | ||
3.4.1 The 3D Cultivation of hPS Cells on Microcarriers | 121 | ||
3.4.2 The 3D Cultivation of hPS Cells Embedded in Hydrogels (Microcapsules) | 126 | ||
3.5 hPS Cell Cultivation on PDL-coated Dishes with Small Molecules | 130 | ||
3.6 Conclusion and Future Perspectives | 131 | ||
Acknowledgments | 133 | ||
References | 133 | ||
Chapter 4 Differentiation Fates of Human ES and iPS Cells Guided by Physical Cues of Biomaterials | 141 | ||
4.1 Introduction | 141 | ||
4.2 Induction Protocols of Human Pluripotent Stem Cells | 145 | ||
4.2.1 EB Formation | 147 | ||
4.2.2 Induction of hPS Cells by EB Generation | 149 | ||
4.2.2.1 Type AB Differentiation of hPS Cells | 150 | ||
4.2.2.2 Type A Differentiation of hPS Cells | 151 | ||
4.2.2.3 Type B Differentiation of hPS Cells | 153 | ||
4.2.2.4 Type C Differentiation of hPS Cells | 153 | ||
4.2.2.5 hPS Cell Induction in Type D | 153 | ||
4.2.3 Induction of hPS Cells Seeded on Materials Directly | 155 | ||
4.2.3.1 Type E Differentiation of hPS Cells (2D Cultivation) | 155 | ||
4.2.3.2 Type E Differentiation of hPS Cells (3D Cultivation) | 156 | ||
4.2.3.3 Type F Differentiation of hPS Cells | 157 | ||
4.2.3.4 Type G Cell Differentiation of hPS Cells | 158 | ||
4.2.3.5 Type H Differentiation of hPS Cells | 160 | ||
4.3 Physical Cues of Materials in hPS Cell Induction | 162 | ||
4.3.1 Effect of Elasticity of Cell Cultivation Biomaterials on Stem Cell Induction | 162 | ||
4.3.1.1 Stiffness of Biomaterials Guides Stem Cell Fate of Differentiation in 2D Cultivation | 163 | ||
4.3.1.2 Pluripotent Maintenance of MS, iPS, and ES Cells on Soft Biomaterials | 171 | ||
4.3.1.3 Mechanism of Stem Cell Induction by Substrate Elasticity and ECM in 2D Cultivation | 173 | ||
4.3.1.4 Biomaterial Stiffness Guides Stem Cell Fate of Differentiation in 3D Cultivation | 175 | ||
4.3.1.5 Results Contradictory to Engler’s Study in 2D Cultivation | 181 | ||
4.3.1.6 Contradictory to Engler’s Research in 3D Cultivation | 185 | ||
4.3.2 Topographic Effects of Biomaterials on the Differentiation Fates of hPS Cells | 186 | ||
4.3.2.1 Preparation of Nano- and Micropatterned Materials | 189 | ||
4.3.2.2 Uniform EB Generation in Micropatterned Surface | 192 | ||
4.3.2.3 Osteogenic and Adipogenic Induction of Stem Cells on Micropatterned Biomaterials | 192 | ||
4.3.2.4 Hepatic, Myogenic, and Chondrogenic Induction of Stem Cells on Micropatterned Biomaterials | 198 | ||
4.3.2.5 NS Cell Induction on Micropatterned Biomaterials | 202 | ||
4.3.3 Stem Cell Induction on Nanofibers | 210 | ||
4.3.3.1 Stem Cell Differentiation on Nanofiber Made by Self-assembly of Amphiphile Peptides | 210 | ||
4.3.3.2 Stem Cell Differentiation on Nanofibers Generated by Electrospinning Methods | 216 | ||
4.3.3.2.1 Size Effect of Nanofibers on Stem Cell Induction | 221 | ||
4.3.3.2.2 Effect of Nanofiber Alignment on Stem Cell Induction | 221 | ||
4.3.3.2.3 Stem Cell Induction on Hybrid Nanofiber | 228 | ||
4.3.3.3 Stem Cell Induction on Nanofibers Prepared Using Phase Separation | 229 | ||
4.3.4 Effect of Electrical and Mechanical Forces of Biomaterials on Induction Fate of hPS Cells | 232 | ||
4.4 Conclusions and Perspectives | 236 | ||
References | 237 | ||
Chapter 5 Biomaterial Control of Differentiation of Human Embryonic Stem Cells and Induced Pluripotent Stem Cells | 252 | ||
5.1 Introduction | 252 | ||
5.2 Induction of hPS Cells into Neural Lineages | 258 | ||
5.2.1 Stromal-induced Differentiation into Neural Lineages | 265 | ||
5.2.2 Induction into Neural Lineages Through EB Generation | 266 | ||
5.2.3 Direct Induction into Neural Lineages on Materials with No EB Generation | 266 | ||
5.2.4 Effect of Cell Cultivation Materials on hPS Cell Induction into Neural Lineages | 269 | ||
5.3 Induction of hPS Cells into Cardiomyocytes | 276 | ||
5.3.1 Efficient Protocols for Inducing hPS Cells into Cardiomyocyte | 277 | ||
5.3.2 Effect of Cell Cultivation Materials on hPS Cell Induction into Cardiomyocytes | 287 | ||
5.4 Induction into Hepatocytes | 296 | ||
5.4.1 Efficient Protocols for hPS Cell Induction into Hepatocytes on Materials | 296 | ||
5.4.2 3D Cultivation Facilitates the Induction of hPS Cells into Hepatocytes | 303 | ||
5.4.3 Effect of Cell Culture Biomaterials on hPS Cell Differentiation into Hepatocytes | 309 | ||
5.5 Differentiation into Insulin-secreting β Cells | 311 | ||
5.6 Conclusions and Perspectives | 316 | ||
Acknowledgments | 317 | ||
References | 318 | ||
Chapter 6 Clinical Trials of Stem Cell Therapies Using Biomaterials | 328 | ||
6.1 Introduction | 328 | ||
6.2 Stem Cell Therapy for Myocardial Infarction (MI) in Clinical Trials | 329 | ||
6.2.1 Clinical Therapies for MI Using hES cells | 330 | ||
6.2.1.1 Fibrin Patch Including hES Cell-derived Cardiac Progenitors | 330 | ||
6.2.1.2 Clinical Trials With Fibrin Patch Including Cardiac Progenitors Derived from HES Cells | 332 | ||
6.2.1.3 Mechanism of Enhanced Function by Fibrin Patches | 333 | ||
6.2.2 Clinical Therapy for MI Using Fetal and Adult Stem Cells | 333 | ||
6.2.2.1 MI Therapy Using Fetal and Adult Stem Cells | 333 | ||
6.2.2.2 MI Therapy Using Human BMN Cells | 337 | ||
6.2.2.3 MI Therapy Using CXCR4+ CD34+ Progenitor Cells | 338 | ||
6.2.2.4 MI Therapy Using Allogeneic and Autologous HMS Cells | 339 | ||
6.2.2.5 MI Therapy Using Autologous Cardiosphere-derived Cells and Cardiac Stem Cells | 340 | ||
6.2.2.6 MI Therapy Using Fetal Stem Cells | 344 | ||
6.2.2.7 MI Therapy Using Adipose-derived Stem (ADS) Cells | 345 | ||
6.2.3 Future Trends of MI Therapy Using Stem Cells | 346 | ||
6.3 Stem Cell Therapy for Macular Degeneration Disease in Clinical Trials | 346 | ||
6.3.1 Macular Degeneration Diseases and Eye Structure | 347 | ||
6.3.2 Bioengineering in Stem Cell Therapies for Macular Degeneration Diseases | 351 | ||
6.3.3 Biomaterial Assists in the Therapies for Macular Degeneration Diseases | 353 | ||
6.3.4 Bioengineering for Clinical Trials Using hES Cell-derived RPE Cells | 358 | ||
6.3.5 Bioengineering for Clinical Trials Using hiPS Cell-derived RPE Sheets | 359 | ||
6.3.6 Bioengineering for Clinical Trials Using Adult Stem Cells | 361 | ||
6.3.7 Clinical Trials Using Fetal Stem Cells | 363 | ||
6.3.8 Future Perspectives of Stem Cell Therapy for Macular Degeneration Diseases | 365 | ||
References | 366 | ||
Chapter 7 Conclusions and Future Perspective on Biomaterial Control of Therapeutic Stem Cells | 374 | ||
7.1 Introduction | 374 | ||
7.2 Chapter 1 | 374 | ||
7.3 Chapter 2 | 374 | ||
7.4 Chapter 3 | 376 | ||
7.5 Chapter 4 | 378 | ||
7.6 Chapter 5 | 379 | ||
7.7 Chapter 6 | 381 | ||
References | 383 | ||
Subject Index | 386 |