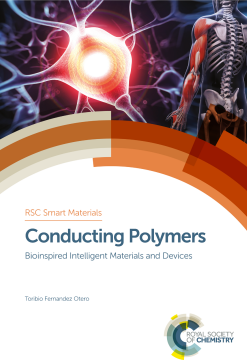
Additional Information
Book Details
Abstract
Conducting polymers are organic, conjugated materials that offer high electrical conductivity through doping by oxidation and a wide range of unique electromechanical and electrochromic characteristics. These properties can be reversibly tuned through electrochemical reactions, making this class of materials good biomimetic models and ideal candidates for the development of novel flexible and transparent sensing devices.
This book comprehensively summarises the current and future applications of conducting polymers, with chapters focussing on electrosynthesis strategies, theoretical models for composition dependent allosteric and structural changes, composition dependent biomimetic properties, novel biomimetic devices and future developments of zoomorphic and anthropomorphic tools.
Written by an expert researcher working within the field, this title will have broad appeal to materials scientists in industry and academia, from postgraduate level upwards.
Table of Contents
Section Title | Page | Action | Price |
---|---|---|---|
Cover | Cover | ||
Conducting Polymers Bioinspired Intelligent Materials and Devices | i | ||
Quote | v | ||
Preface | vii | ||
Acknowledgements | xi | ||
Contents | xiii | ||
Chapter 1 - Life, Bioinspiration, Chemo-Biomimesis and Intelligent Materials | 1 | ||
1.1 Introduction | 1 | ||
1.2 Basic Hypotheses | 1 | ||
1.3 Bioinspiration, Biomimesis, Chemo-Biomimesis, Intelligent Materials and Systems | 2 | ||
1.4 Available Reactive Materials | 3 | ||
1.5 Intrinsic CPs | 4 | ||
1.5.1 Available Material Families | 5 | ||
1.6 Biomimetic Reactive Gels | 6 | ||
References | 8 | ||
Chapter 2 - Electrochemical Methods | 12 | ||
2.1 Introduction | 12 | ||
2.2 Two Electrode Electrochemical Cells | 12 | ||
2.3 Three Electrode Electrochemical Cells | 15 | ||
2.4 Four Electrode Electrochemical Cells | 16 | ||
2.5 Cyclic Voltammetry | 17 | ||
2.5.1 Voltammetric and Coulovoltammetric Responses | 19 | ||
2.5.2 Electrolyte Potential Window | 20 | ||
2.6 Square Potential Steps: Chronoamperometric, Chronocoulometric and Reaction Kinetic Responses | 21 | ||
2.7 Galvanostatic Methodologies: Chronopotentiometric Responses | 23 | ||
2.8 Electrochemical Cells and Methods Using Solid State Electrolytes | 24 | ||
References | 25 | ||
Chapter 3 - Electrosynthesis of Conducting Polymers | 26 | ||
3.1 Introduction | 26 | ||
3.2 Linear Potential Sweep: Monomer Oxidation Potential | 27 | ||
3.3 Electropolymerization by Consecutive Potential Sweeps | 27 | ||
3.3.1 Electropolymerization and Polymer Passivation (Degradation) | 30 | ||
3.4 Electropolymerization at a Constant Potential (Potentiostatic) | 31 | ||
3.5 Electropolymerization by Consecutive Square Potential Waves | 33 | ||
3.6 Electropolymerization by Flow of a Constant Current (Galvanostatic) | 34 | ||
3.7 Tafel Slope Mechanism Using Clean Metal Electrodes | 35 | ||
3.8 Electropolymerization Mechanism | 35 | ||
3.9 Electrochemical and Gravimetric Methodologies | 37 | ||
3.10 Gravimetric Empirical Electropolymerization Kinetics | 38 | ||
3.11 Empirical Kinetics from the Electropolymerization Charge | 40 | ||
3.12 Electrochemical Polymerization Kinetics: Tafel Slopes from Clean Metal Electrodes | 41 | ||
3.13 Tafel Slopes from Polymer-Coated Electrodes | 42 | ||
3.14 Electropolymerization and the Properties of the Electrogenerated Films | 42 | ||
3.15 Analysis of the Polymerization Kinetics | 44 | ||
3.16 Parallel Polymeric Degradation–Cross-Linking During Synthesis | 44 | ||
3.17 Parallel Chemical Polymerization | 46 | ||
3.18 Parallel Adsorption of Macroions | 47 | ||
3.19 Shift of the Molecular Interaction Forces: Electrodissolution | 48 | ||
3.20 Incorporation of Different Material Nanoparticles | 49 | ||
3.21 Polymerization Mechanism | 50 | ||
3.22 General Comments | 50 | ||
3.23 Synthesis of New Polymeric Compounds by Ionic Substitution | 52 | ||
3.24 Electropolymerization Initiated by Electrochemical Reduction | 52 | ||
References | 53 | ||
Chapter 4 - Gel Membrane Electrodes: Electrochemical Reactions | 59 | ||
4.1 Introduction | 59 | ||
4.1.1 Inert and Reactive Electrodes | 60 | ||
4.2 Conducting Polymers as Electroactive Electrodes | 60 | ||
4.3 Electrochemical Reactions | 61 | ||
4.4 Some Considerations Related to Conducting Polymer Reactions | 63 | ||
4.5 Giant Non-Stoichiometry: Transfer of Consecutive Electrons and Continuous Polymer/Ion Composition Evolution | 64 | ||
4.6 Ionic Composition Variation with Stable Physical Integrity | 66 | ||
4.7 Electrochemical Responses from Different Methodologies | 68 | ||
4.7.1 Voltammetric Responses | 69 | ||
4.7.2 Coulovoltammetric Responses: Full Electrochemical Reversibility | 70 | ||
4.7.3 Chronoamperometric Responses | 72 | ||
4.7.4 Chronopotentiometric Responses | 73 | ||
4.8 Detecting Parallel Irreversible Reactions | 73 | ||
4.8.1 Parallel Irreversible Reactions from Films Coating Metal Electrodes | 74 | ||
4.8.2 Parallel Irreversible Reactions from Self-Supported Polymeric Electrodes | 75 | ||
4.8.2.1 Basic Conducting Polymers | 75 | ||
4.8.2.2 Polymer Blends with Organic Macroions | 77 | ||
References | 77 | ||
Chapter 5 - Membrane Composition-Dependent Electrochemical Properties | 81 | ||
5.1 Introduction | 81 | ||
5.2 Electronic Conductivity | 81 | ||
5.3 Volume Variation | 83 | ||
5.4 Color Shift | 83 | ||
5.5 Charge Storage | 86 | ||
5.6 Ionic Storage | 87 | ||
5.7 Transversal Ionic Conductivity and Diffusivity Tuning | 87 | ||
5.8 Material Potential Shift | 87 | ||
5.9 Surface Property Control | 88 | ||
5.10 Ion Delivery | 88 | ||
5.11 Packed Ionic-Conformational Energetic States | 88 | ||
5.12 Chemo-Biomimetic Functions | 89 | ||
5.13 ICM Electro-Chemo-Biomimicry | 90 | ||
References | 90 | ||
Chapter 6 - Reaction-Driven Conformational, Allosteric and Structural Changes | 92 | ||
6.1 Introduction | 92 | ||
6.2 Reversible Chain Molecular Motors | 92 | ||
6.3 Oxidation/Reduction Reactions Drive Macroscopic Structural Changes | 94 | ||
6.4 Reaction-Driven Structural Components | 94 | ||
6.4.1 Reaction-Driven Anion Exchanges | 95 | ||
6.4.2 Reaction-Driven Cation Exchanges | 97 | ||
6.5 Erasing Structural and Chemical Memories: Steady State Responses | 99 | ||
6.6 Other Electrochemical Responses Reveal Reaction-Driven Structural Changes | 100 | ||
6.7 Voltammetric Responses | 100 | ||
6.8 Chronoamperometric Responses | 103 | ||
6.9 Direct Visual Observation of the Oxidation–Relaxation–Nucleation Process | 105 | ||
6.10 Visual Tracing of the Giant Non-Stoichiometric Nature of Conducting Polymers | 107 | ||
6.11 Relaxation–Nucleation Starts at the Polymer/Electrolyte Interface | 108 | ||
6.12 Chronopotentiometric Responses | 110 | ||
6.13 Ion Trapping by Structural Effects | 110 | ||
6.13.1 Ion Trapping During Electropolymerization | 110 | ||
6.13.2 Anion Trapping by Reduction–Compaction During p-Dedoping | 111 | ||
6.13.3 Cation Trapping by Oxidation–Compaction During n-Dedoping or p-Doping | 111 | ||
6.13.4 Low Band-Gap Polymers Trap Anions During p-Dedoping and Cations During n-Dedoping | 111 | ||
6.14 Analytical Evidences of the Ionic Content in Deeply Reduced Films | 113 | ||
6.15 Electronic Conductivity of Deeply Reduced Films | 114 | ||
6.16 Hydrogen Inhibition from Aqueous Solutions | 115 | ||
6.17 In situ Monitoring of Reaction-Driven Dimensional Changes | 115 | ||
6.18 In situ Monitoring of Reaction-Driven Mass Variations | 116 | ||
6.19 Influence of the Charge Balancing Ion Dimensions | 116 | ||
6.20 Solvent Influence | 117 | ||
6.21 Other Reaction-Driven Conformational, Allosteric and Structural Responses from Different Artificial and Biological Materials... | 118 | ||
6.22 Physical-Driven Conformational Changes | 118 | ||
References | 119 | ||
Chapter 7 - Conformational, Allosteric and Structural Chemistry: Theoretical Description | 124 | ||
7.1 Introduction | 124 | ||
7.2 The ESCR Model | 127 | ||
7.2.1 Conformational Relaxation–Nucleation: Relaxation Time, Conformational Energy and Relaxation Energy | 129 | ||
7.2.2 Structure and Chemical Reactions | 130 | ||
7.2.3 Structural Chemical Kinetic (SCK) Model | 131 | ||
7.2.4 Structural Activation Energy | 136 | ||
7.2.5 Structural Reaction Coefficient | 138 | ||
7.2.6 Structural Reaction Orders | 139 | ||
7.2.7 The SCK Model Includes Chemical Kinetic Models | 139 | ||
7.3 Structural Chronoamperometric Responses: Theoretical Simulation | 140 | ||
7.4 Structural Voltammetric Responses: Theoretical Description | 142 | ||
7.5 Structural Coulovoltammetric Responses: Theoretical Description | 145 | ||
7.6 Structural Chronopotentiometric Responses: Theoretical Description | 145 | ||
7.7 Final Considerations | 148 | ||
References | 149 | ||
Chapter 8 - Electro-Chemo-Biomimetic Devices | 152 | ||
8.1 Introduction | 152 | ||
8.2 Artificial Muscles | 153 | ||
8.2.1 Bilayer and Tri-Layer Bending Devices | 155 | ||
8.2.2 Electro-Chemo-Dynamic Characterization of Artificial Muscles | 156 | ||
8.2.3 The Driving Current Controls the Angular Movement of the Polymeric Motor | 157 | ||
8.2.4 The Consumed Charge Controls Displacement and Relative Position | 159 | ||
8.2.5 Artificial Muscles are Robust, Reproducible, Reliable and Faradaic Polymeric Motors | 160 | ||
8.2.6 Dynamic Hysteresis and Creeping Effects Under Cycling | 160 | ||
8.2.7 Artificial Muscles as a Tool to Clarify Reaction-Driven Ionic Exchanges | 162 | ||
8.2.8 Artificial Muscles as Tools to Quantify Relative Solvent Exchanges | 164 | ||
8.2.9 Osmotic and Electro-Osmotic Processes During Actuation | 166 | ||
8.3 Smart Membranes Tune Transversal Ionic Flow | 168 | ||
8.4 Artificial Glands: Smart Chemical Dosage and Smart Drug Delivery | 169 | ||
8.5 Chemical Decontamination and Ionic Concentration | 171 | ||
8.6 Artificial Chemical Synapse (Man–Computer Interface) and a New Hypothesis for Brain Functions | 172 | ||
8.7 Chemo-Ionic-Conformational Memories as Possible Brain Memory Models | 176 | ||
8.8 Smart Surfaces (Wettability and Self-Cleaning Control) | 177 | ||
8.9 Electrochromic Devices: Smart Windows, Glasses and Mirrors | 178 | ||
8.10 All-Organic Batteries and Supercapacitors | 179 | ||
8.11 Sensors, Biosensors and Sensing Devices | 181 | ||
8.11.1 Le Châtelier’s Principle, Chemical Equilibrium and Sensors | 181 | ||
8.11.2 Biochemical Sensors: The New Conformational (Allosteric) Ways | 182 | ||
8.11.3 Mechanical Sensors | 182 | ||
8.12 Challenges | 182 | ||
References | 183 | ||
Chapter 9 - Multi-Tool Devices Mimicking Brain–Organ Intercommunication | 192 | ||
9.1 Introduction | 192 | ||
9.2 Electrochemically and Chemically Driven Multifunctionality | 192 | ||
9.3 Multi-Tool Devices | 193 | ||
9.4 Otero’s Sensing Principle During Reaction | 194 | ||
9.5 Sensing Materials: Reactive Mechanical, Chemical, Thermal or Electrical Sensors | 195 | ||
9.5.1 Reaction-Driven Mechanical Sensors | 195 | ||
9.5.2 Reaction-Driven Thermal Sensors | 195 | ||
9.5.3 Reaction-Driven Chemical Sensors | 197 | ||
9.5.4 Reaction-Driven Electrical Sensors | 197 | ||
9.5.5 New Aspects of Three-Dimensional Structural Reactions: Reacting Material, Consumed Charge and Working Energetic Conditions | 198 | ||
9.6 Two Tools Working Simultaneously in One Device: Sensing Artificial Muscles | 198 | ||
9.6.1 Mechanical Sensing Muscles | 200 | ||
9.6.2 Chemical Sensing Muscles | 200 | ||
9.6.3 Thermal Sensing Muscles | 200 | ||
9.6.4 Electrical Sensing Muscles | 202 | ||
9.6.5 Tactile Artificial Muscles | 202 | ||
9.7 The Multi-Tool Device: One Motor and Several Sensors Working Simultaneously in a Physically Uniform Device | 204 | ||
9.8 Proprioception: Artificial Proprioception from Sensing Artificial Muscles | 204 | ||
9.9 Theoretical Description of Artificial Proprioception | 206 | ||
9.9.1 Potential and Consumed Energy Evolution During Actuation: Stair Functions | 209 | ||
9.10 Dual Actuator–Sensor Systems | 210 | ||
9.10.1 Dual Actuator–Mechanical Sensor: Experiments and Model | 210 | ||
9.10.2 Dual Actuator–Chemical Sensor: Experiments and Model | 216 | ||
9.10.3 Dual Actuator–Thermal Sensor: Experiments and Model | 218 | ||
9.10.4 Dual Actuator–Electrical Sensor: Experiments and Model | 220 | ||
9.11 One Actuator and Several Simultaneous Sensors in One Device | 222 | ||
9.12 Other Multi-Tool Devices | 222 | ||
9.13 Intelligent Electrochemical Materials for Multi-Tool Devices | 223 | ||
References | 223 | ||
Chapter 10 - Final Comments and Challenges | 226 | ||
10.1 Introduction | 226 | ||
10.2 Reactions and Structures | 227 | ||
10.3 Other Artificial Materials Giving Reaction-Driven Structural Responses | 229 | ||
10.3.1 Conducting Polymers Exchanging Cations During p-Doping/p-Dedoping | 229 | ||
10.3.2 Conducting Polymers Exchanging Cations During n-Doping/n-Dedoping | 230 | ||
10.3.3 Monolayers of Bipyridyl Cations | 230 | ||
10.3.4 Very Large Carbon Nanotubes | 230 | ||
10.4 Biological Processes and Conformational Structures | 231 | ||
10.4.1 Muscular Action in Striated Muscles | 232 | ||
10.4.2 Allosteric Chemical Responses from Enzymes | 232 | ||
10.4.2.1 The Enzymatic Activation Energy May Quantitatively Describe Enzymatic Reaction Rates | 233 | ||
10.4.3 Allosteric and Cooperative Effects from Hemoglobin and Other Proteins | 234 | ||
10.4.3.1 Structural Reaction Coefficients can Describe Allosteric Reactions | 235 | ||
10.4.4 Molecular and Viral Activity and Conformational Structure: The Ebola Virus | 235 | ||
10.4.5 Allosteric Effects from Nucleic Acids | 236 | ||
10.4.6 Conformational Movements of Ion Channel Proteins | 236 | ||
10.5 Challenges | 237 | ||
References | 241 | ||
Subject Index | 243 |