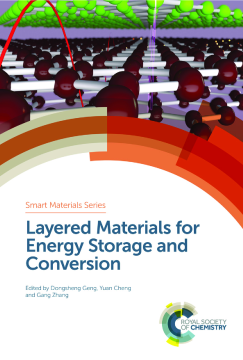
BOOK
Layered Materials for Energy Storage and Conversion
Dongsheng Geng | Yuan Cheng | Gang Zhang
(2019)
Additional Information
Book Details
Abstract
The considerable interest in graphene and 2D materials is sparking intense research on layered materials due to their unexpected physical, electronic, chemical, and optical properties. This book will provide a comprehensive overview of the recent and state-of-the-art research progress on layered materials for energy storage and other applications. With a brief introduction to layered materials, the chapters of this book gather various fascinating topics such as electrocatalysis for fuel cells, lithium-ion batteries, sodium-ion batteries, photovoltaic devices, thermoelectric devices, supercapacitors and water splitting. Unique aspects of layered materials in these fields, including novel synthesis and functionalization methods, particular physicochemical properties and consequently enhanced performance are addressed. Challenges and perspectives for layered materials in these fields will also be presented. With contributions from key researchers, Layered Materials for Energy Storage and Conversion will be of interest to students, researchers and engineers worldwide who want a basic overview of the latest progress and future directions.
Table of Contents
Section Title | Page | Action | Price |
---|---|---|---|
Cover | Cover | ||
Contents | vii | ||
Chapter 1 MO-derived Materials for Extremely Efficient Electrocatalysis | 1 | ||
1.1 Introduction | 1 | ||
1.2 MOF-derived Materials: Structures and Compositions | 2 | ||
1.2.1 Structures of MO-derived Materials | 3 | ||
1.2.1.1 MOF-derived Porous Carbon | 3 | ||
1.2.1.2 MOF-derived Hollow Structures | 4 | ||
1.2.2 Compositions of MOF-derived Materials | 5 | ||
1.2.2.1 Metal-free Nanocarbon | 5 | ||
1.2.2.2 Transition Metal/Metal Compound-decorated Nanocarbon | 7 | ||
1.2.2.3 Micro/Nano-structured MOF-derived Composites | 10 | ||
1.2.2.4 MOF-supported Noble Metal NPs | 14 | ||
1.3 MOF-derived Materials for Efficient Electrocatalysis | 16 | ||
1.3.1 MO-derived Electrocatalysts for the HER | 16 | ||
1.3.2 MO-derived Electrocatalysts for the OER | 21 | ||
1.3.3 MO-derived Electrocatalysts for the ORR | 24 | ||
1.3.3.1 Monometal-MOF-derived Electrocatalysts for the ORR | 25 | ||
1.3.3.2 Bimetal-MOF-derived Electrocatalysts for the ORR | 26 | ||
1.3.3.3 MOFs/Composite-derived Electrocatalysts for the ORR | 30 | ||
1.3.4 MOF-derived Electrocatalysts for CO2 Reduction | 32 | ||
1.4 Summary and Perspectives | 33 | ||
Acknowledgments | 34 | ||
References | 35 | ||
Chapter 2 Two-dimensional Layered Materials for High-performance Lithium-ion Batteries | 39 | ||
2.1 Introduction | 39 | ||
2.2 Graphene and Its Composites as Electrodes in LIBs | 40 | ||
2.2.1 Anodes | 41 | ||
2.2.1.1 Graphene Anodes | 41 | ||
2.2.1.2 Graphene-based Composite Anodes | 46 | ||
2.2.2 Graphene-based Composite Cathodes | 52 | ||
2.3 2D Layered Anode Materials Beyond Graphene | 57 | ||
2.3.1 Xenes | 57 | ||
2.3.2 MXenes | 57 | ||
2.3.3 Transition Metal Chalcogenides | 58 | ||
2.4 Conclusions | 64 | ||
Acknowledgments | 64 | ||
References | 64 | ||
Chapter 3 Intercalation-based Layered Materials for Rechargeable Sodium-ion Batteries | 71 | ||
3.1 Introduction to Sodium-ion Batteries (SIBs) | 71 | ||
3.2 Intercalation Based Layered Electrode Materials for SIBs | 73 | ||
3.2.1 Layered Sodium Cobalt Oxide and Its Derivatives as Cathodes for SIBs | 74 | ||
3.2.2 Layered Sodium Nickel Oxide and Its Derivatives as Cathodes for SIBs | 76 | ||
3.2.3 Layered Sodium Manganese Oxide and Its Derivatives as Cathodes for SIBs | 78 | ||
3.2.4 Layered Sodium Iron Oxide and Its Derivatives as Cathodes for SIBs | 80 | ||
3.2.5 Layered Sodium Chromium Oxide and Its Derivatives as Cathodes for SIBs | 82 | ||
3.2.6 Layered Sodium Vanadium Oxide and Its Derivatives as Cathodes for SIBs | 83 | ||
3.2.7 Layered Sodium Titanium Oxide and Its Derivatives as Cathodes for SIBs | 84 | ||
3.2.8 Na-rich Layered Oxides as Cathode Materials for SIBs | 85 | ||
3.2.9 Layered Na2Ti3O7 as an Anode Material for SIBs | 86 | ||
3.3 Sodium-ion Full Cell Based on Layered Cathode Materials | 89 | ||
3.4 Summary | 90 | ||
Acknowledgments | 90 | ||
References | 90 | ||
Chapter 4 Ionic Liquid Electrolytes for Graphene-based Supercapacitors with an Ultrahigh Energy Density | 95 | ||
4.1 Introduction | 95 | ||
4.2 Graphene-based Electrode Materials | 103 | ||
4.2.1 Functionalized/Functional Graphene Electrodes | 104 | ||
4.2.2 Graphene/Heteroatom Electrodes | 106 | ||
4.2.3 Graphene/Carbon Hybrid Material Electrodes | 107 | ||
4.2.4 Graphene/Polymer Hybrid Material Electrodes | 109 | ||
4.2.5 Conclusion | 111 | ||
4.3 Ionic Liquid Electrolytes | 112 | ||
4.3.1 Single Ionic Liquid Electrolytes | 113 | ||
4.3.2 Mixed Ionic Liquid Electrolytes | 113 | ||
4.3.3 Ionic Liquid/Polymer Electrolytes | 115 | ||
4.3.4 Conclusion | 117 | ||
4.4 Graphene/Ionic Liquid Supercapacitors: Investigation of the Interface of Graphene and Ionic Liquid | 117 | ||
4.5 Supercapacitors for the Next Generation of Wearable and Portable Electronic Devices | 118 | ||
4.6 Ionic Liquids for Other 2D-based Supercapacitors | 120 | ||
4.7 Conclusion | 122 | ||
Acknowledgments | 123 | ||
References | 123 | ||
Chapter 5 Properties and Applications of Layered Thermoelectric Materials | 129 | ||
5.1 Introduction | 129 | ||
5.2 Bi2Te3 | 130 | ||
5.2.1 Materials Development | 130 | ||
5.2.2 Device Performance | 134 | ||
5.3 SnSe | 140 | ||
5.4 BiCuSeO | 147 | ||
5.5 Intercalated Layered Materials | 156 | ||
5.6 Summary | 159 | ||
Acknowledgments | 160 | ||
References | 160 | ||
Chapter 6 Graphene-Carbon Nanotube Hybrid Films for High-performance Photovoltaic Devices | 165 | ||
6.1 Introduction | 165 | ||
6.2 Mathematical Methods for Finding the Equilibrium Configurations of the Super-cells of Graphene-CNT Hybrid Films | 166 | ||
6.2.1 AIREBO | 166 | ||
6.2.2 SCC DFTB | 168 | ||
6.3 Results and Discussion | 169 | ||
6.3.1 Atomistic Models and Band Structure of Mono- and Bilayer Graphene-CNT Hybrid Films | 169 | ||
6.3.2 Electrical Conductivity of Graphene-CNT Hybrid Film | 177 | ||
6.3.3 Optical Conductivity and Optical Properties of Graphene-CNT Hybrid Film | 183 | ||
6.3.4 Prospects for Using Graphene-CNT Hybrid Film in Photovoltaics | 192 | ||
6.4 Conclusion | 194 | ||
Acknowledgments | 195 | ||
References | 195 | ||
Chapter 7 Metal-Organic Frameworks (MOFs) as Potential Hybrid Ferroelectric Materials | 197 | ||
7.1 Introduction | 197 | ||
7.2 Experimental Characterization | 200 | ||
7.2.1 Phase Transition and Anomaly in Dielectric Constant | 200 | ||
7.2.2 Scanning Second Harmonic Generation Microscopy | 201 | ||
7.2.3 Sawyer-Tower Circuit | 203 | ||
7.2.4 Structural Analysis by X-ray Diffraction | 205 | ||
7.2.5 Nanoscale Spectroscopic Measurements | 206 | ||
7.3 Simulation Methods | 211 | ||
7.3.1 Monte Carlo Method with the Ginzburg-Landau Model | 213 | ||
7.3.2 Ab Initio Supported Model Simulation | 214 | ||
7.4 Ferroelectric MOFs | 216 | ||
7.4.1 Intrinsic Ferroelectric MOFs | 216 | ||
7.4.2 Induced Ferroelectric MOFs | 220 | ||
7.4.3 MOFs with Ferroelectric-like Behaviour | 222 | ||
7.5 Dipoles in MOF Lattices | 224 | ||
7.5.1 Molecular Rotators | 224 | ||
7.6 Two-dimensional (2D) MOFs | 225 | ||
7.6.1 Synthesis of 2D MOF Nanosheets | 225 | ||
7.6.1.1 Top-down Methods | 225 | ||
7.6.1.2 Bottom-up Methods | 226 | ||
7.6.2 2D Ferroelectric MOFs | 226 | ||
7.7 Mechanical Properties of MOFs | 228 | ||
7.8 Conclusion | 233 | ||
Acknowledgments | 234 | ||
References | 234 | ||
Chapter 8 Synthetic Techniques and Functionalization Approaches of 2D Transition Metal Dichalcogenides | 245 | ||
8.1 Introduction | 245 | ||
8.2 Micromechanical Cleavage | 247 | ||
8.3 Ion Intercalation-assisted Liquid Exfoliation | 249 | ||
8.3.1 Chemical Intercalation and Exfoliation | 250 | ||
8.3.2 Electrochemical Intercalation and Exfoliation | 253 | ||
8.4 Mechanical Force-assisted Liquid Exfoliation | 254 | ||
8.4.1 Sonication-assisted Liquid Exfoliation | 256 | ||
8.4.1.1 Exfoliation in a ‘‘Good’’ Solvent | 256 | ||
8.4.1.2 Exfoliation in the Presence of a Surfactant/Polymer or Stabilizer | 258 | ||
8.4.2 Shear Force-assisted Liquid Exfoliation | 261 | ||
8.5 Wet-chemical Synthesis Method | 261 | ||
8.5.1 Hydrothermal Synthesis | 262 | ||
8.5.2 Solvothermal Synthesis | 263 | ||
8.5.3 Hot-injection Method | 265 | ||
8.6 Chemical Vapour Deposition | 267 | ||
8.7 Chemical Functionalization and Metal Hybridization of 2D TMD Nanosheets | 270 | ||
8.7.1 Covalent Functionalization | 271 | ||
8.7.2 Coordinate Functionalization | 273 | ||
8.7.3 Metal Hybridization of TMD Nanosheets | 274 | ||
8.8 Conclusions and Outlook | 275 | ||
References | 276 | ||
Chapter 9 High Electrocatalytic Performance of Two-dimensional Layered MoS2-based Materials for the Hydrogen Evolution Reaction | 283 | ||
9.1 Introduction | 283 | ||
9.1.1 Why 2D Layered MoS2 Nanosheets? | 284 | ||
9.2 Properties of MoS2 Nanosheets | 286 | ||
9.3 MoS2-based Materials | 288 | ||
9.4 MoS2-based Electrocatalyst | 288 | ||
9.5 Hydrogen Evolution Reaction | 289 | ||
9.5.1 Emerging Design Strategy for Improving the Electrocatalytic Performance of MoS2 | 291 | ||
9.5.1.1 Engineering Edges | 294 | ||
9.5.1.2 Engineering Defects | 294 | ||
9.5.1.3 Engineering Strain | 296 | ||
9.5.1.4 Doping Engineering | 296 | ||
9.5.1.5 Interlayer Engineering | 299 | ||
9.5.1.6 Interface Engineering | 301 | ||
9.5.1.7 Phase Engineering | 302 | ||
9.5.2 New Outcomes for Tuning the Catalytic Activity of MoS2-based Materials | 303 | ||
9.6 Conclusions and Outlook | 304 | ||
Acknowledgments | 305 | ||
References | 305 | ||
Subject Index | 311 |