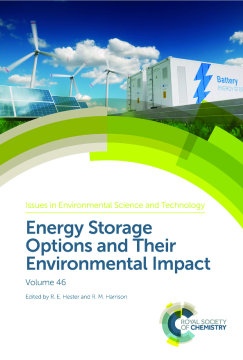
Additional Information
Book Details
Abstract
Recent decades have seen huge growth in the renewable energy sector, spurred on by concerns about climate change and dwindling supplies of fossil fuels. One of the major difficulties raised by an increasing reliance on renewable resources is the inflexibility when it comes to controlling supply in response to demand. For example, solar energy can only be produced during the day. The development of methods for storing the energy produced by renewable sources is therefore crucial to the continued stability of global energy supplies.
However, as with all new technology, it is important to consider the environmental impacts as well as the benefits. This book brings together authors from a variety of different backgrounds to explore the state-of-the-art of large-scale energy storage and examine the environmental impacts of the main categories based on the types of energy stored.
A valuable resource, not just for those working and researching in the renewable energy sector, but also for policymakers around the world.
Table of Contents
Section Title | Page | Action | Price |
---|---|---|---|
Cover | Cover | ||
Preface | v | ||
Contents | ix | ||
Editors | xv | ||
List of Contributors | xvii | ||
Energy Sources and Supply Grids – The Growing Need for Storage | 1 | ||
1 Introduction | 2 | ||
2 Energy Sources | 3 | ||
2.1 Generation of Electricity from Combustion of Fossil Fuels | 3 | ||
2.2 Nuclear Power | 7 | ||
2.3 Renewables: Solar, Wind, Wave, Tidal and Hydro | 9 | ||
2.4 Geothermal, Combined Heat and Power, Biomass Combustion and Waste Incineration | 13 | ||
3 Operation of Electricity Networks | 16 | ||
3.1 Transmission Network | 18 | ||
3.2 Distribution Network | 19 | ||
3.3 Distributed Generation | 19 | ||
3.4 Mini Grids | 20 | ||
4 Stabilisation of the Electricity Grid | 23 | ||
4.1 System Support Services | 23 | ||
4.2 Impact of Renewables on Operation of Electricity Grid | 25 | ||
4.3 Corrective Measures for Mitigating RoCoF | 27 | ||
4.4 Demand-side Solutions and Smart Grids | 28 | ||
4.5 Need for Energy Storage | 30 | ||
5 Electric Vehicles and the Electricity Grids | 31 | ||
5.1 Slow Charging | 33 | ||
5.2 Fast Charging | 33 | ||
5.3 End-of-life Usage | 35 | ||
5.4 Implications of Connecting Electric Vehicles to the Electricity Grid | 35 | ||
6 Conclusion | 36 | ||
References | 38 | ||
Mechanical Systems for Energy Storage – Scale and Environmental Issues. Pumped Hydroelectric and Compressed Air Energy Storage | 42 | ||
1 Introduction | 42 | ||
2 Pumped Hydroelectric Storage - Introduction to the Technology, Geology and Environmental Aspects | 45 | ||
2.1 Efficiencies and Economics | 49 | ||
2.2 UK Deployment of PHS | 51 | ||
2.3 Environmental and Regulatory Factors in PHS | 53 | ||
3 Compressed Air Energy Storage - Introduction to the Technologies, Geology and Environmental Aspects | 65 | ||
3.1 Applications of CAES | 69 | ||
3.2 CAES Configurations - DCAES, ACAES/AACAES, ICAES | 69 | ||
3.3 Geological Storage Options | 73 | ||
3.4 Operational Modes of CAES ‘Reservoirs' | 78 | ||
3.5 UK Potential for Deployment of CAES | 80 | ||
3.6 Planning and Regulatory Environment for CAES | 81 | ||
3.7 Environmental Performance, Emissions, Sustainability and Economics of CAES Systems | 85 | ||
3.8 Safety Record of CAES and Some Potential Risks (Human and Environmental) | 97 | ||
Acknowledgments | 98 | ||
References | 98 | ||
Electrochemical Energy Storage | 115 | ||
1 Introduction | 116 | ||
1.1 Electrolytic and Voltaic Cells | 116 | ||
1.2 Batteries, Fuel Cells and Flow Batteries | 117 | ||
2 Lead-Acid Batteries | 118 | ||
2.1 Fundamental Aspects of Lead-Acid Batteries | 119 | ||
2.2 Electrodes | 121 | ||
2.3 Cell Designs | 122 | ||
2.4 Cycle Depth | 124 | ||
2.5 Environmental Aspects | 124 | ||
3 Lithium and Lithium-ion Batteries | 125 | ||
3.1 Basic Theory, Structure and Operation | 125 | ||
3.2 Materials | 127 | ||
3.3 Electrolytes | 127 | ||
3.4 Separators | 129 | ||
3.5 Sustainability of Lithium-ion Batteries | 129 | ||
4 Other Battery Chemistries | 130 | ||
4.1 Sodium-Sulfur Batteries | 130 | ||
4.2 Nickel-Metal Hydride Batteries | 131 | ||
5 Fuel Cells | 131 | ||
5.1 Low-temperature Fuel Cells | 132 | ||
5.2 High-temperature Fuel Cells | 135 | ||
5.3 Fuel Cells for Energy Storage | 136 | ||
5.4 Environmental Issues with Hydrogen Production and Distribution | 137 | ||
6 Flow Batteries | 138 | ||
6.1 Traditional Redox Flow Batteries: The All-vanadium Flow Battery | 139 | ||
6.2 Hybrid Flow Batteries: The Zinc-Bromine Flow Battery | 141 | ||
6.3 Slurry Flow Batteries: The All-iron Flow Battery | 141 | ||
6.4 Other Flow Battery Systems | 142 | ||
7 Summary and Conclusions | 142 | ||
References | 144 | ||
Electrical Storage | 150 | ||
1 Introduction | 150 | ||
2 Supercapacitor and Supercapattery | 151 | ||
2.1 Basics of Energy Storage Devices | 151 | ||
2.2 Pseudobattery-type Electrode Materials | 156 | ||
2.3 Supercapattery Performance | 163 | ||
2.4 Prospects and Future | 165 | ||
3 Superconducting Magnetic Energy Storage (SMES) | 166 | ||
3.1 Basic Aspects of SMES | 166 | ||
3.2 State-of-the-Art, Trends and Challenges for SMES | 167 | ||
4 Flywheels, Flywheel Batteries and Synchronous Condensers | 169 | ||
4.1 Fundamental Theory of Mechanical Energy Storage | 169 | ||
4.2 Basic Aspects of Flywheels | 171 | ||
4.3 Basic Aspects of Synchronous Motors, Generators and Condensers | 175 | ||
4.4 Current Trends and Challenges for Flywheels | 177 | ||
References | 179 | ||
Photochemical Energy Storage | 184 | ||
1 Introduction | 184 | ||
2 Classes of Solar Fuels and Feedstocks | 186 | ||
2.1 Sustainable H2 Production | 188 | ||
2.2 Sustainable Carbon Fuels Through CO2 Reduction | 189 | ||
3 Reaction Enhancement and Selectivity by Catalysis | 192 | ||
4 Current Status of Light-driven Fuel Production | 194 | ||
4.1 PV-driven Electrolysis of Water to Generate H2 | 194 | ||
4.2 PV-driven Electrolysis for CO2 Reduction | 196 | ||
4.3 Photochemical and Photoelectrochemical Cells | 198 | ||
5 Summary and Conclusions | 204 | ||
References | 204 | ||
Thermal and Thermochemical Storage | 210 | ||
1 Introduction | 210 | ||
2 Latent Heat Storage | 211 | ||
2.1 Principle of LHS | 211 | ||
2.2 Materials for LHS | 212 | ||
2.3 Encapsulation and Composite Technology for LHS | 212 | ||
2.4 Heat Exchangers for LHS | 215 | ||
2.5 Applications of LHS | 216 | ||
3 Thermochemical Energy Storage | 219 | ||
3.1 Principle of TCES | 219 | ||
3.2 Variety of TCES | 222 | ||
3.3 Material and Reactor Technologies for TCES | 223 | ||
3.4 Applications of TCES | 225 | ||
3.5 Challenges and Barriers to Implementation | 226 | ||
References | 226 | ||
Smart Energy Systems | 228 | ||
1 Smart Energy Systems | 229 | ||
1.1 General Objectives | 229 | ||
1.2 Reducing the Need for Fuels | 230 | ||
1.3 Smart Electric, Thermal and Gas Grids | 232 | ||
1.4 Coupling of Energy Sectors | 233 | ||
2 Potential of Smart Energy Systems and Sector Coupling | 236 | ||
2.1 IDA Energy Vision 2050 | 236 | ||
2.2 Smart Energy Europe | 238 | ||
2.3 The Energy System Analysis Tool EnergyPLAN | 240 | ||
3 The Need for Storage in a Smart Energy Systems Perspective | 241 | ||
3.1 Assessment of Storage Needs: A Function of the Demands | 241 | ||
3.2 Comparison of Costs for Different Storage Types | 242 | ||
4 The Relevance of Storage in a Smart Energy System | 247 | ||
4.1 Renewable Fuels | 247 | ||
4.2 Large-scale Hydroelectric Storage | 250 | ||
4.3 Local Electric Storage in Electric Vehicles | 252 | ||
4.4 Thermal Storage | 253 | ||
5 Conclusion | 255 | ||
References | 257 | ||
Life-cycle Analysis for Assessing Environmental Impact | 261 | ||
1 Introduction to Life-cycle Assessment | 262 | ||
2 Life-cycle Assessment of Energy Storage Systems | 263 | ||
3 Selection of Impact Indicators | 264 | ||
4 Case Study 1: Life-cycle Assessment of Pumped Hydroelectric Storage and Battery Storage | 267 | ||
4.1 Goal and Scope | 267 | ||
4.2 Description of Compared Systems and Functional Equivalency | 268 | ||
4.3 Underlying Data | 269 | ||
4.4 Results | 271 | ||
4.5 Sensitivity Analysis | 273 | ||
4.6 Discussion | 274 | ||
5 Case Study 2: Life-cycle Assessment of Different Lithium-ion Battery Chemistries for a Small-scale Energy System | 276 | ||
5.1 Goal and Scope | 276 | ||
5.2 Underlying Data | 277 | ||
5.3 Results | 280 | ||
5.4 Discussion | 285 | ||
6 Case Study 3: Life-cycle Assessment of Energy Scenarios with Various Uses of Heat and Battery Storage for a Small-scale Energy System | 286 | ||
6.1 Goal and Scope | 286 | ||
6.2 Description of Compared Systems and Functional Equivalency | 287 | ||
6.3 Underlying Data | 288 | ||
6.4 Results | 288 | ||
6.5 Discussion | 290 | ||
7 Conclusion | 291 | ||
Abbreviations | 292 | ||
Acknowledgments | 292 | ||
References | 293 | ||
Business Opportunities and the Regulatory Framework | 296 | ||
1 Introduction | 297 | ||
2 Economic Value of Storage | 301 | ||
2.1 Matching Technologies to Applications | 301 | ||
2.2 Merit Order of Alternative Storage Options | 305 | ||
2.3 Location and Energy Density of Storage Units | 307 | ||
2.4 Optimal Sizing of Storage Units | 308 | ||
2.5 Economic Impact of Aging of Batteries | 309 | ||
2.6 Prosumer Concept | 309 | ||
2.7 Energy Cloud Concepts | 310 | ||
3 Value Creation for Business Models | 310 | ||
3.1 Subsidies and Tariff Schemes | 310 | ||
3.2 Economic Value from Energy (Self-) Supply | 311 | ||
3.3 Economic Value from Ancillary Services | 312 | ||
3.4 Economic Value from Arbitrage | 314 | ||
3.5 Virtual Power Plants (VPPs) with Storage | 316 | ||
4 Regulatory Considerations | 319 | ||
5 Conclusion | 320 | ||
References | 320 | ||
Subject Index | 327 |