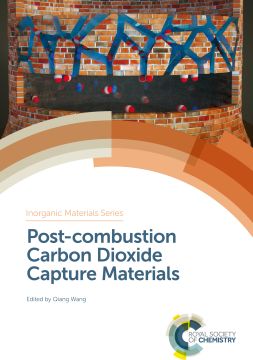
Additional Information
Book Details
Abstract
Inorganic solid adsorbents/sorbents are attractive materials for capturing carbon dioxide (CO2) from flue gases after fossil fuel combustion.
Post-combustion Carbon Dioxide Capture Materials introduces the key inorganic materials used as adsorbents/sorbents with specific emphasis on their design, synthesis, characterization, performance, and mechanism. Dedicated chapters cover carbon-based adsorbents, zeolite- and silica-based adsorbents, metal–organic framework (MOF)-based adsorbents, and alkali-metal-carbonate-based adsorbents. The final chapter discusses the practical application aspects of these adsorbents used in carbon dioxide capture from flue gases.
Edited and written by world-renowned scientists in each class of the specific material, this book will provide a comprehensive introduction for advanced undergraduates, postgraduates and researchers from both academic and industrial fields wishing to learn about the topic.
Table of Contents
Section Title | Page | Action | Price |
---|---|---|---|
Cover | Cover | ||
Post-combustion Carbon Dioxide Capture Materials | i | ||
Preface | v | ||
Contents | vii | ||
Chapter 1 - Carbon-based CO2 Adsorbents | 1 | ||
1.1 Introduction | 1 | ||
1.2 Porous Carbons | 3 | ||
1.2.1 Chemical Activation | 3 | ||
1.2.1.1 KOH as an Activating Agent | 3 | ||
1.2.1.2 Other Chemicals as Activating Agents | 11 | ||
1.2.2 Physical Activation | 13 | ||
1.2.2.1 CO2 as an Activating Agent | 13 | ||
1.2.2.2 Steam as an Activating Agent | 14 | ||
1.2.3 Metal Ion Activation | 15 | ||
1.2.4 Templating Method | 22 | ||
1.2.4.1 Porous Silica as a Hard Template | 25 | ||
1.2.4.2 Zeolite as a Hard Template | 26 | ||
1.2.4.3 Porous Organic Frameworks as Self-templates | 27 | ||
1.2.4.4 Carbide Lattices as Self-templates | 30 | ||
1.2.4.5 Triblock Copolymer as a Soft Template | 32 | ||
1.2.5 Combined Method of Templating and Activation | 34 | ||
1.2.5.1 Two-step Process | 34 | ||
1.2.5.2 One-step Process | 36 | ||
1.3 Graphene-based Porous Materials | 38 | ||
1.3.1 Graphene-based Adsorbents by Chemical Activation | 38 | ||
1.3.2 Graphene-based Adsorbents by Physical Activation | 39 | ||
1.3.3 Graphene-based Adsorbents by Other Techniques | 40 | ||
1.4 Carbon Nanotubes | 41 | ||
1.5 Carbon-based Hybrid Adsorbents | 44 | ||
1.5.1 Carbon–Organic Hybrid Adsorbents | 44 | ||
1.5.2 Carbon–Inorganic Hybrid Adsorbents | 46 | ||
1.6 Effect of Carbon Structure on CO2 Adsorption | 47 | ||
1.6.1 Pore Size Effect | 47 | ||
1.6.1.1 Analysis of Porosity | 47 | ||
1.6.1.2 Micropore Filling Mechanism of CO2 Adsorption | 49 | ||
1.6.1.3 Pore Size Effect at Different Sorption Pressures | 51 | ||
1.6.1.4 Pore Size Effect at Different Adsorption Temperatures | 52 | ||
1.6.2 Surface Chemistry Effect on CO2 Adsorption | 53 | ||
1.6.2.1 Effect of Nitrogen Doping | 55 | ||
1.6.2.2 Effect of Other Heteroatom-doping | 61 | ||
1.7 Summary and Outlook | 64 | ||
Acknowledgements | 65 | ||
References | 65 | ||
Chapter 2 - Zeolite and Silica-based CO2 Adsorbents | 76 | ||
2.1 Introduction | 76 | ||
2.2 (Alkali) Silicates | 79 | ||
2.2.1 Silicate Amine-based Adsorbents | 79 | ||
2.2.1.1 CO2 Absorption in Aqueous Alkanolamine Solutions | 79 | ||
2.2.2 Synthesis of Amine–Silica Adsorbents | 82 | ||
2.2.2.1 Impregnation Method | 82 | ||
2.2.2.2 Post-synthesis Grafting | 83 | ||
2.2.2.3 Direct Condensation | 84 | ||
2.2.3 CO2 Capture by Amine–Silica-based Adsorbents | 84 | ||
2.2.3.1 Amine Impregnated on Silica | 84 | ||
2.2.3.1.1\rEffect of Temperature.As demonstrated by Xu et al.,48,54 a decrease in temperature from 75 °C to 25 °C causes a dramatic decreas... | 84 | ||
2.2.3.1.2\rEffect of CO2 Partial Pressure.CO2 adsorption capacity is also influenced by the partial pressure of CO2 in the gas feed. Regard... | 87 | ||
2.2.3.1.3\rEffect of Water.Xu et al. also studied the influence of water on CO2 adsorption capacity using PEI (50 wt%)/MCM-41. The authors ... | 87 | ||
2.2.3.1.4\rEffect of Percentage of Impregnation.According to the work of Xu et al.,48 the amount of amine (PEI) deposited on MCM-41 mesopor... | 87 | ||
2.2.3.1.5\rEffect of the Support Structure.There is also a correlation between the CO2 adsorption capacities of amines impregnated on diffe... | 87 | ||
2.2.3.1.6\rEffect of the Mesopores Structuring Agent.CO2 capture was tested with tetraethylenepentamine (TEPA) impregnated on MCM-41 and SB... | 88 | ||
2.2.3.2 Amine Grafted on Silica | 88 | ||
2.2.3.2.1\rEffect of CO2 Partial Pressure.In the case of APTES (3-aminopropyltriethoxysilane)-type aminosilanes for grafting on MCM-48-type... | 88 | ||
2.2.3.2.2\rEffect of Water.Similar to the results obtained in the case of amines impregnated on silica, the presence of water during CO2 ad... | 91 | ||
2.2.3.2.3\rEffect of the Support.As shown in Table 2.5, the important influence of the support on CO2 adsorption capacities can also be dem... | 91 | ||
2.2.3.2.4\rEffect of Organosilane Type.Hiyoshi et al.74 have shown that the nature of the aminosilanes used in the synthesis of amine-graft... | 92 | ||
2.3 Alkali Silicate-based Sorbents | 92 | ||
2.3.1 Calcium Silicate (CaSiO3) | 92 | ||
2.3.2 Sodium Metasilicate (Na2SiO3) | 93 | ||
2.3.3 Lithium Orthosilicate (Li4SiO4) and Other Lithium Silicates | 94 | ||
2.3.3.1 Li4SiO4 | 94 | ||
2.3.3.1.1\rInfluence of the Operating Conditions.Theoretically, Li4SiO4 can adsorb up to 0.367 g of CO2 per gram of Li4SiO4 (∼8.34 mmol CO2... | 95 | ||
2.3.3.1.2\rImprovement of the Absorption Performance of Li4SiO4.The improvement of the CO2 adsorption performance of Li4SiO4 requires modif... | 95 | ||
2.3.3.2 Other Lithium Silicates | 98 | ||
2.4 Clays-based Adsorbents | 99 | ||
2.4.1 Phyllosilicates | 99 | ||
2.4.2 Clays for CO2 Capture | 99 | ||
2.4.2.1 Smectite | 100 | ||
2.4.2.2 Montmorillonite | 103 | ||
2.4.2.3 Bentonite | 104 | ||
2.4.2.4 Saponite | 105 | ||
2.4.2.5 Hectorite | 106 | ||
2.4.2.6 Laponite | 107 | ||
2.4.2.7 Sepiolite | 107 | ||
2.5 Mineral Silicates for Carbonation | 108 | ||
2.5.1 Mineral Carbonation | 108 | ||
2.5.2 Silicates as Natural Minerals for Carbonation | 109 | ||
2.5.3 Mineral Pre-treatments | 110 | ||
2.5.4 Thermodynamics of Mineral Carbonation | 110 | ||
2.5.5 Processes for Mineral Carbonation | 111 | ||
2.5.5.1 Mineral Carbonation Ex Situ | 112 | ||
2.5.5.1.1\rMineral Carbonation ‘Ex-Situ’: Aqueous Carbonation.Mineral carbonation in an aqueous medium occurs according to three important ... | 112 | ||
2.5.5.1.2\rMineral Carbonation ‘Ex-Situ’: Gas–Solid Route.The gas–solid method is the easiest approach towards mineral carbonation. The mag... | 112 | ||
2.5.5.2 Mineral Carbonation In Situ | 114 | ||
2.5.5.3 Other Mineral Carbonation Routes | 115 | ||
2.6 Zeolites and Related Materials | 115 | ||
2.6.1 Foreword | 115 | ||
2.6.2 Peculiarities of Zeolites | 116 | ||
2.6.3 CO2 Sorption in Zeolites: Main Issues | 117 | ||
2.6.3.1 Preamble | 117 | ||
2.6.3.2 Key Parameters and Mechanistic Considerations | 119 | ||
2.6.3.3 Zeolite Acid–Base Properties: How Do They Impact the Sorption Features | 126 | ||
2.6.3.4 CO2 Sorption in Alkali-exchanged Zeolites: the ‘Li’ Paradox | 128 | ||
2.6.4 Miscellaneous Parameters | 129 | ||
2.6.4.1 Impact of the Presence of Water | 129 | ||
2.6.4.2 Impact of Sorbent Particle Size and Morphology | 130 | ||
2.6.4.3 Selectivity | 131 | ||
2.6.4.4 Possibility of Dual-site Adsorption | 131 | ||
2.6.5 Zeolite-like Materials as Precursors to Design Performant Li-silicate Sorbents: How to Bridge the Gap Between High Affinity... | 132 | ||
2.7 Outline: Towards an Efficient Chemical Transformation of CO2 into Fuels | 133 | ||
2.7.1 Potential Chemical Valorization of Carbon Dioxide | 134 | ||
2.7.2 Synthesis of Energy Carriers | 134 | ||
2.7.3 Future Prospects | 136 | ||
2.8 Conclusion | 138 | ||
Acknowledgements | 139 | ||
References | 139 | ||
Chapter 3 - Metal–Organic Framework (MOF)-based CO2 Adsorbents | 153 | ||
3.1 Introduction | 153 | ||
3.2 CO2 Adsorption by MOFs With Open Metal Sites | 155 | ||
3.3 CO2 Adsorption by Amine-functionalized MOFs | 158 | ||
3.3.1 In situ Synthesized Amine-functionalized MOFs | 160 | ||
3.3.1.1 Amine-functionalized MOFs With a Structural Motif | 160 | ||
3.3.1.2 Amine-functionalized MOFs Without a Structural Motif | 164 | ||
3.3.2 Post-synthesis Amine-functionalized MOFs | 165 | ||
3.3.2.1 Post-synthesis Functionalization of MOFs via Covalent Bonding | 166 | ||
3.3.2.2 Post-synthetic Functionalization of MOFs via Coordination Bonding | 167 | ||
3.3.3 Physical Incorporation of Amines into Unmodified MOFs | 170 | ||
3.4 CO2 Adsorption by Mixed-ligand-based MOFs | 171 | ||
3.4.1 Pillared-layer Mixed-ligand MOFs (PL-MOFs) | 171 | ||
3.4.2 Cluster-based Mixed-ligand MOFs | 173 | ||
3.5 CO2 Adsorption by Flexible Ligand-based MOFs (FL-MOFs) | 174 | ||
3.5.1 Increasing the Free Pore Volume in FL-MOFs | 175 | ||
3.5.2 Maintaining Porosity in FL-MOFs After the Removal of the Solvent | 177 | ||
3.5.3 Increasing the Gas Binding Affinity in FL-MOFs | 178 | ||
3.6 CO2 Adsorption by MOFs with Interpenetration | 179 | ||
3.7 CO2 Adsorption by Zeolitic Imidazolate Frameworks (ZIFs) | 180 | ||
3.8 CO2 Adsorption by Composite MOFs | 183 | ||
3.8.1 MOF–Carbon Composites | 183 | ||
3.8.2 Composites of MOFs with Other Support Materials | 187 | ||
3.9 CO2 Adsorption by MOFs under Humid Conditions | 189 | ||
3.10 Conclusion and Perspectives | 193 | ||
Acknowledgements | 194 | ||
References | 194 | ||
Chapter 4 - Alkali-metal-carbonate-based CO2 Adsorbents | 206 | ||
4.1 Introduction | 206 | ||
4.1.1 Sodium Carbonate (Na2CO3) | 207 | ||
4.1.2 Potassium Carbonate (K2CO3) | 208 | ||
4.2 CO2 Capture of Na2CO3 and K2CO3 Under Moist Conditions | 209 | ||
4.2.1 CO2 Capture of Na2CO3 Under Moist Conditions | 209 | ||
4.2.1.1 Experimental | 210 | ||
4.2.1.1.1\rSample Preparation.Analytical reagent grade sodium bicarbonate (NaHCO3) was used during experiments of decomposition of NaHCO3 a... | 210 | ||
4.2.1.1.2\rBicarbonate Formation Measurements.The obtained samples were processed with the TG-DTA apparatus using a gas composition of CO2 ... | 210 | ||
4.2.1.1.3\rCrystal Structure and Morphology Measurements.The crystal structures of the products after CO2 occlusion reactions with 10, 20, ... | 211 | ||
4.2.1.2 Results and Discussion | 211 | ||
4.2.1.2.1\rCO2 Capture of Na2CO3 at Different Temperatures.The decomposition of NaHCO3 occurred with TG-DTA under pure N2 gas via the rever... | 211 | ||
4.2.1.2.2\rCO2 Capture of Na2CO3 Under Various CO2 Concentrations.The dependence of the sorptivity of Na2CO3 on CO2 concentration was shown... | 214 | ||
4.2.1.2.3\rCO2 Capture of Na2CO3 Under Various H2O Concentrations.As mentioned in Section 4.2.1.2.2, the bicarbonate formation of Na2CO3 pr... | 222 | ||
4.2.1.3 Conclusions | 227 | ||
4.2.2 Capture of CO2 of K2CO3 Under Moist Conditions | 227 | ||
4.2.2.1 Experimental | 229 | ||
4.2.2.1.1\rSample Preparation.KHCO3 (99.5% chemical purity) was used during the experiments of decomposition of KHCO3 and bicarbonate forma... | 229 | ||
4.2.2.1.2\rBicarbonate Formation Measurements.K2CO3 bicarbonate formation was measured with the TG-DTA apparatus, as shown in Section 4.2.1... | 229 | ||
4.2.2.1.3\rCrystal Structure and Morphology Measurements.The crystal structures of the products after reaction times of 1, 5, 20, 40, 60, a... | 229 | ||
4.2.2.2 Results and Discussion | 229 | ||
4.2.2.2.1\rBicarbonate Formation of K2CO3.KHCO3 decomposed to K2CO3, CO2, and H2O as per the reverse of reaction (4.15). This was confirmed... | 229 | ||
4.2.2.2.2\rExothermic Properties and Temperature Variation.Since the process of bicarbonate formation of K2CO3 leads to a temperature eleva... | 234 | ||
4.2.2.2.3\rStructural Changes in the CO2 Sorption of K2CO3.The bicarbonate formation of K2CO3 was examined by measuring the XRD patterns of... | 236 | ||
4.2.2.2.4\rSample Morphologies with Reaction Time.The morphological variation of K2CO3 was examined via SEM observations before and after C... | 238 | ||
4.2.2.2.5\rCO2 Capture of K2CO3 Under Various H2O Concentrations.As mentioned in the CO2 capture of K2CO3 under different CO2 concentration... | 243 | ||
4.2.2.2.6\rCO2 Capture of K4H2(CO3)3·1.5H2O Under Different H2O or CO2 Concentrations.The CO2 occlusion of K4H2(CO3)3·1.5H2O displayed a sl... | 250 | ||
4.2.2.3 Conclusions | 253 | ||
4.2.3 Improvements of the CO2 Sorptivity of Na2CO3 and K2CO3 | 254 | ||
4.2.3.1 Improvement of the CO2 Sorptivity of Na2CO3 | 254 | ||
4.2.3.2 Improvement of the CO2 Sorptivity of K2CO3 | 255 | ||
4.3 Attempts of Practical Use | 256 | ||
Acknowledgements | 256 | ||
References | 256 | ||
Chapter 5 - Application Status of Post-combustion CO2 Capture | 259 | ||
5.1 Introduction | 259 | ||
5.1.1 GHG Emission | 259 | ||
5.1.2 Pre-combustion Carbon Capture | 260 | ||
5.1.3 Post-combustion Carbon Capture | 261 | ||
5.1.4 Oxy-firing | 263 | ||
5.1.5 Chemical Looping Combustion (CLC) | 264 | ||
5.1.6 Carbon Capture and Storage (CCS) | 264 | ||
5.1.7 Natural Gas Combustion | 265 | ||
5.2 Current Status of CCS Projects | 267 | ||
5.2.1 Large-scale Projects | 268 | ||
5.2.1.1 SaskPower Boundary Dam- CCS | 268 | ||
5.2.1.2 Petra Nova Carbon Capture, Texas, USA | 269 | ||
5.2.1.3 Kemper County Energy Facility (IGCC + CCS) | 271 | ||
5.2.1.4 Callide – Oxy-fuel Combustion and Carbon Storage Demonstration Plant | 272 | ||
5.2.1.5 NET Power Clean Energy Large-scale Pilot Plant | 273 | ||
5.2.2 Small-scale Projects | 273 | ||
5.2.2.1 Fluor Econamine FG PlusSM | 273 | ||
5.2.2.2 MHI KM-CDR Process | 275 | ||
5.2.2.3 Alstom Chilled Ammonia Process (ACAP) | 275 | ||
5.2.2.4 Powerspan ECO2™ Process | 277 | ||
5.2.2.5 Cansolv | 278 | ||
5.2.2.6 Aker Clean Carbon | 279 | ||
5.2.2.7 Alstom Advanced Amine Process | 281 | ||
5.2.2.8 Siemens POSTCAP Amino Acid Salt | 281 | ||
5.3 Environmental and Economic Concerns | 283 | ||
5.4 Conclusion | 285 | ||
References | 286 | ||
Subject Index | 290 |