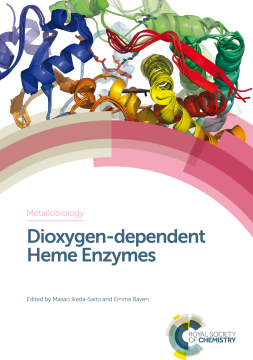
Additional Information
Book Details
Abstract
Aerobic organisms have evolved to utilise the intrinsic oxidising power of oxygen from the atmosphere. This so-called 'activation' of oxygen is often catalysed by a heme-containing enzyme. This book highlights the many and varied catalytic activities of O2-dependent heme–iron enzymes, including monoxygenases and cytochrome P450, dioxygenases, oxidases and model heme systems.
Dioxygen-dependent Heme Enzymes will be a useful resource for postgraduate students and researchers in biochemistry and metallobiology working in, or moving into, research areas involving heme proteins.
Table of Contents
Section Title | Page | Action | Price |
---|---|---|---|
Cover | Cover | ||
Dioxygen-dependent Heme Enzymes | i | ||
Preface | v | ||
Biographies | vii | ||
Contents | xxi | ||
Section I - Model Systems | 1 | ||
Chapter 1 - Dioxygen Binding and Activation Mediated by Transition Metal Porphyrinoid Complexes | 3 | ||
1.1 Introduction | 3 | ||
1.2 Role of Transition Metals in Binding and Activating O2 | 4 | ||
1.3 Metalloproteins That Bind and Transport O2 | 5 | ||
1.4 Activation of O2 by Heme Enzymes | 7 | ||
1.4.1 Heme Monooxygenases | 8 | ||
1.4.1.1 Cytochrome P450 | 8 | ||
1.4.1.2 Nitric Oxide Synthase | 9 | ||
1.4.1.3 Heme Oxygenase | 10 | ||
1.4.2 Heme Dioxygenases | 12 | ||
1.4.2.1 Tryptophan 2,3-Dioxygenase (TDO) and Indoleamine 2,3-Dioxygenase (IDO) | 12 | ||
1.5 Metallo-porphyrin and -Porphyrinoid Models for O2 Binding and Activation | 13 | ||
1.5.1 Iron Complexes | 15 | ||
1.5.1.1 Iron Porphyrins, Phthalocyanines, and Porphyrazines | 15 | ||
1.5.1.2 Iron Corroles and Corrolazines | 21 | ||
1.5.2 Manganese Complexes | 22 | ||
1.5.2.1 Manganese Porphyrins, Phthalocyanines, and Porphyrazines | 22 | ||
1.5.2.2 Manganese Corroles and Corrolazines | 25 | ||
1.6 Summary and Future Directions | 29 | ||
Acknowledgements | 30 | ||
References | 30 | ||
Chapter 2 - Design and Engineering of Heme Enzymes With O2-dependent Catalytic Activity | 37 | ||
2.1 Introduction | 37 | ||
2.2 Structural and Functional Models of Heme-containing Monooxygenases and Dioxygenases | 38 | ||
2.2.1 The Biological Function of the Cytochrome P450 Monooxygenases | 38 | ||
2.2.2 The Active Site and Catalytic Cycle of the Cytochrome P450 Monooxygenases | 38 | ||
2.3 Recent Designs that Utilize Alanine Scanning | 39 | ||
2.4 Semi-rational and Rational Design of the P450 Enzymes | 40 | ||
2.5 P450s as a Model for Dioxygen Activation | 41 | ||
2.6 Heme Dioxygenases | 41 | ||
2.7 Functional Models of the Heme-containing Oxidases | 43 | ||
2.7.1 Biological Functions of Terminal Oxidases | 43 | ||
2.7.2 Structure of Heme–Copper Oxidases | 43 | ||
2.7.3 Biosynthetic Models of Heme–Copper Oxidase in Myoglobin | 43 | ||
2.7.3.1 Functional Model of a Heme–Copper Center in a Mb Scaffold | 43 | ||
2.7.3.2 Fine Tuning the Oxidase Activity with Non-native Heme Cofactors | 44 | ||
2.7.3.3 The Role of Non-heme Metal in Promoting O–O Bond Cleavage | 44 | ||
2.7.3.4 Non-covalent Interactions in Tuning the Reduction Potential and Proton Transfer | 46 | ||
2.7.3.5 Defining the Role of the Active Site Tyrosine by Genetic Incorporation of Tyrosine Analogs | 47 | ||
2.7.3.6 Improving the Oxidase Activity by Optimization of Interfacial Electron Transfer | 48 | ||
2.7.4 Oxygen Activation by de novo Designed Heme Proteins | 49 | ||
2.7.4.1 De novo Designed Heme-binding Maquettes | 49 | ||
2.7.4.2 Oxygen Binding and Activation by Cytochrome c Maquettes | 50 | ||
2.7.4.3 Heme Oxygenase Activity of Heme-binding Maquettes | 51 | ||
2.7.4.4 Electrocatalytic Oxygen Reduction by Mimochromes | 52 | ||
2.8 Heme-binding DNA/RNAzymes | 53 | ||
2.8.1 Heme-binding Aptamers with Oxidase Activity | 53 | ||
2.8.2 Scope of Oxidation Activity by Heme-binding DNA/RNAzymes | 56 | ||
2.9 Conclusions and Future Perspectives | 56 | ||
Acknowledgements | 57 | ||
References | 57 | ||
Chapter 3 - Myoglobin Derivatives Reconstituted with Modified Metal Porphyrinoids as Structural and Functional Models of the Cytochrome P450 Enzymes | 63 | ||
3.1 Introduction | 63 | ||
3.2 Reconstitution of Hemoproteins | 65 | ||
3.3 Mechanistic Studies of Cytochrome P450cam: the Role of the Heme–Propionate Side Chains | 66 | ||
3.3.1 Role of the 6-Propionate Side Chain | 67 | ||
3.3.2 The Role of the 7-Propionate Side Chain | 69 | ||
3.4 Modeling of the P450 Enzymes by Myoglobin with Artificial Cofactors | 71 | ||
3.4.1 Reductive O2 Activation by Flavomyoglobin | 72 | ||
3.4.2 C–H Bond Activation by Myoglobin with Manganese Porphycene | 73 | ||
3.5 Conclusion and Future Prospects | 76 | ||
References | 77 | ||
Chapter 4 - Investigating Heme Enzymes with Expanded Genetic Codes | 79 | ||
4.1 Introduction | 79 | ||
4.2 What Is Genetic Code Expansion | 80 | ||
4.3 Unnatural Amino Acids Used in Structural Studies | 81 | ||
4.3.1 NMR Probes | 81 | ||
4.3.2 Spin Probes | 84 | ||
4.3.3 Infrared Probes | 86 | ||
4.4 Enzyme Activity Improvement | 87 | ||
4.4.1 Altering the Heme Coordination Environment | 88 | ||
4.4.2 Altering the Enantioselectivity or Substrate Binding Though Steric Effects | 90 | ||
4.4.3 Modulating the Redox Potential of the Cofactor | 92 | ||
4.4.4 Protein Electrode Immobilization | 95 | ||
4.5 Conclusion | 96 | ||
References | 97 | ||
Section II - Heme reactivity | 103 | ||
Chapter 5 - What Drives the Rate-determining Step for Oxygen Atom Transfer by Heme Compound I | 105 | ||
5.1 Introduction | 105 | ||
5.2 Valence Bond Modelling of the Mechanism of Cytochrome P450 Compound I | 106 | ||
5.3 Valence Bond Curve Crossing Diagrams | 112 | ||
5.4 Two-parabola Curve Crossing Model | 113 | ||
5.5 Applications of the Two-parabola VB Model | 118 | ||
5.5.1 Case Study 1: Desaturation Versus Hydroxylation Pathways | 118 | ||
5.5.2 Case Study 2: Trends in Substrate Sulphoxidation Reactions | 121 | ||
5.6 Conclusions | 123 | ||
Acknowledgements | 123 | ||
References | 123 | ||
Chapter 6 - Cytochrome P450 Decarboxylases | 127 | ||
6.1 Introduction | 127 | ||
6.2 OleT: A Member of the CYP152 Family of Fatty Acid Hydroxylases | 129 | ||
6.3 Substrate Scope for Decarboxylation | 132 | ||
6.4 Parallels to Other CYP Oxidations | 133 | ||
6.5 Identification of the OleT Oxidant and the Abstraction Steps | 134 | ||
6.6 Origins for Perturbed Radical Recombination | 136 | ||
6.6.1 Electronic Effects | 136 | ||
6.6.2 Structural, Mutagenesis, and Ortholog Studies | 137 | ||
6.7 Future Outlook: Leveraging the P450 Decarboxylases | 139 | ||
References | 140 | ||
Chapter 7 - Oxygen Activation and Long-range Electron Transfer in MauG | 144 | ||
7.1 Introduction: MauG Function and Reactivity | 144 | ||
7.2 Structure of the MauG/preMADH Complex | 146 | ||
7.3 Formation and Stabilization of Bis-Feiv | 148 | ||
7.3.1 The HS Heme | 148 | ||
7.3.2 The LS Heme | 149 | ||
7.3.3 Charge Resonance Stabilization | 150 | ||
7.4 Decay Pathways of Bis-Feiv | 152 | ||
7.4.1 Catalysis by Hole Hopping | 152 | ||
7.4.2 Autoreduction of Bis-Feiv | 153 | ||
7.5 Functional Diversity in the Di-heme CCP Family | 156 | ||
References | 158 | ||
Section III - Oxygenases | 161 | ||
Chapter 8 - Biological Heme Degradation | 163 | ||
8.1 Introduction | 163 | ||
8.1.1 Early Heme Degradation Studies | 164 | ||
8.1.2 Pathogenic Bacterial HOs | 165 | ||
8.2 The HO Catalytic Mechanism | 166 | ||
8.2.1 Heme to Meso-hydroxyheme | 166 | ||
8.2.2 Hydroxyheme to Verdoheme | 170 | ||
8.2.3 Verdoheme to Biliverdin | 171 | ||
8.2.4 Product Release | 171 | ||
8.3 The IsdG Family of Heme Degradation Enzymes | 172 | ||
8.4 Concluding Remarks | 176 | ||
Acknowledgements | 176 | ||
References | 176 | ||
Chapter 9 - Structure, Function and Regulation of Human Heme-based Dioxygenases | 181 | ||
Part A: The Structure and Function of Human TDO and IDO1 | 182 | ||
9.1 Introduction | 182 | ||
9.2 Crystal Structure of hTDO | 183 | ||
9.2.1 Overall Structure | 183 | ||
9.2.2 Active Site Structure | 185 | ||
9.2.3 The JK-Loop, DE-Loop and R144 | 185 | ||
9.2.4 Exosite and Helix–Loop–Helix Motif | 186 | ||
9.2.5 In-crystal Dioxygenase Reaction | 186 | ||
9.2.6 Implication to the Dioxygenase Mechanism | 187 | ||
9.3 Crystal Structure of hIDO1 | 190 | ||
9.3.1 Overall Structure | 190 | ||
9.3.2 Active Site Structure | 191 | ||
9.3.3 Substrate-inhibition and the Inhibitory Substrate Binding Site | 191 | ||
9.3.4 Comparison with hTDO | 193 | ||
9.4 Structures of hIDO1 and hTDO in Complex with Inhibitors | 193 | ||
9.5 Comparison with Bacterial TDOs | 195 | ||
9.6 Comparison with Other Trp Oxidizing Enzymes | 196 | ||
9.6.1 PrnB | 196 | ||
9.6.2 MarE | 198 | ||
9.7 Concluding Remarks | 198 | ||
Part B: In Vivo Regulation of Mammalian TDO and IDO1 | 199 | ||
9.8 Introduction | 199 | ||
9.9 Physiological Regulation of Mammalian Hepatic TDO | 200 | ||
9.9.1 Glucocorticoid (GC)-mediated Transcriptional Regulation | 200 | ||
9.9.2 Heme-mediated Regulation | 200 | ||
9.9.2.1 Heme-mediated Transcriptional Regulation of TDO: A Role for Heme Responsive Elements and/or Heme-dependent TFs | 200 | ||
9.9.2.2 Heme Regulates De Novo TDO Synthesis via Heme-regulated Inhibitor (HRI)-mediated Translation Control | 205 | ||
9.9.2.3 Heme-regulation of TDO-function and Protein Turnover Through Saturation of the Heme-free Apoprotein | 206 | ||
9.9.3 Tryptophan-mediated Substrate Regulation of TDO-protein Turnover | 207 | ||
9.9.4 Negative Regulation of Hepatic TDO Through NAD(P)H-mediated Allosteric Binding | 208 | ||
9.10 In Vivo Pathophysiologic Regulation of IDO1 | 209 | ||
9.10.1 In Vivo Regulation of the Pathophysiologic Function of IDO | 209 | ||
9.10.2 Regulation of IDO1 as a Signaling Molecule | 212 | ||
9.10.3 iNOS-mediated Post-translational Inactivation of IDO | 212 | ||
9.11 Concluding Remarks | 213 | ||
Acknowledgements | 214 | ||
References | 214 | ||
Chapter 10 - Modeling O2-dependent Heme Enzymes: A Quick Guide for Non-experts | 222 | ||
10.1 Introduction | 222 | ||
10.1.1 Modeling Techniques | 223 | ||
10.1.1.1 Bioinformatics | 223 | ||
10.1.1.2 Molecular Modeling | 225 | ||
10.1.1.2.1\rMolecular Mechanics.MM methods are based on classical physic laws and are mainly used to predict and describe molecular structur... | 225 | ||
10.1.1.2.2\rQuantum Mechanics.QM methods provide the most accurate description for an all-atom model by solving the Schrödinger equation.32 ... | 225 | ||
10.1.1.2.3\rQM/MM.This technique combines a QM and MM description of the system, allowing quantum methods to be applied to larger biological... | 226 | ||
10.1.1.2.4\rMolecular Dynamics (MD).MD allows the study of the system dynamics by the iterative resolution of Newton's equations.35 At each ... | 227 | ||
10.1.1.2.5\rDocking Simulations.These techniques propose the preferred bound orientation between molecules, being mostly used in predicting ... | 227 | ||
10.1.1.2.6\rMonte Carlo (MC).In MC based methods, the conformational exploration is obtained by the random (stochastic) motion of the system... | 227 | ||
10.2 Applied Studies | 227 | ||
10.2.1 Bioinformatics | 228 | ||
10.2.1.1 Sequence Based Methods | 228 | ||
10.2.1.1.1\rComputational Phylogenetics.Zuckerkandl and Pauling postulated in 1965 that the difference between two DNA or protein sequences ... | 229 | ||
10.2.1.1.2\rHomology Modelling.With alignment algorithms it is possible to obtain a list of homologs to a given sequence (query) and to sele... | 229 | ||
10.2.1.2 Structure Based Methods | 230 | ||
10.2.1.2.1\rENCoM Server.This server introduces a coarse-grained normal mode analysis tool, shown to improve conformational sampling and cap... | 230 | ||
10.2.1.2.2\rCavity Detection Tools.Protein channels and cavities play important roles in biochemical function, serving as binding or access/... | 230 | ||
10.2.1.2.3\rSubstrate Prediction Methods.One important aspect in CYP is predicting if (and how) molecules will get metabolized, an issue wit... | 230 | ||
10.2.2 Molecular Modeling | 231 | ||
10.2.2.1 Classical Methods | 231 | ||
10.2.2.1.1\rDocking Studies.IDO involvement in immune response and cancer has raised the interest in elucidating its mechanism and in the de... | 231 | ||
10.2.2.1.2\rMolecular Dynamics.MD is the most well-known method for modeling protein dynamics, which has been applied, for example, to under... | 232 | ||
10.2.2.2 Quantum Methods | 233 | ||
10.2.2.2.1\rTDO/IDO.The properties of human IDO/TDO have been extensively studied. The detailed dioxygenase mechanism, however, still remain... | 233 | ||
10.2.2.2.2\rCYP.The important role of CYPs in drug metabolism has attracted the interest of many researchers in addressing the oxygenation m... | 236 | ||
10.3 Conclusion | 237 | ||
References | 237 | ||
Section IV - P450s | 249 | ||
Chapter 11 - Structures of Human Cytochrome P450 Enzymes: Variations on a Theme | 251 | ||
11.1 Introduction | 251 | ||
11.2 Individual Enzymes | 254 | ||
11.2.1 The CYP1 Family | 254 | ||
11.2.2 CYP2A6 | 254 | ||
11.2.3 CYP2A13 | 255 | ||
11.2.4 CYP2B6 | 256 | ||
11.2.5 CYP2C8 | 256 | ||
11.2.6 CYP2C9 | 257 | ||
11.2.7 CYP2C19 | 258 | ||
11.2.8 CYP2D6 | 258 | ||
11.2.9 CYP2E1 | 259 | ||
11.2.10 CYP2R1 | 260 | ||
11.2.11 CYP3A4 | 260 | ||
11.2.12 CYP7A1 | 261 | ||
11.2.13 CYP8A1 | 262 | ||
11.2.14 CYP11A1 | 263 | ||
11.2.15 CYP11B2 | 264 | ||
11.2.16 CYP17A1 | 265 | ||
11.2.17 CYP19A1 | 265 | ||
11.2.18 CYP21A2 | 266 | ||
11.2.19 CYP46A1 | 267 | ||
11.2.20 CYP51A1 | 268 | ||
11.3 Summary | 268 | ||
References | 269 | ||
Chapter 12 - Controlling the Regio- and Stereoselectivity of Cytochrome P450 Monooxygenases by Protein Engineering | 274 | ||
12.1 Introduction | 274 | ||
12.1.1 The Mechanism of CYPs | 275 | ||
12.1.2 A Short Introduction to Protein Engineering Techniques | 276 | ||
12.2 Early Examples of CYP Protein Engineering | 277 | ||
12.3 Recent Developments in the Directed Evolution and Rational Design of CYPs | 278 | ||
12.3.1 Engineering the Substrate Acceptance of the CYPs | 279 | ||
12.3.2 Engineering the Regio- and Stereoselective CYP-catalyzed Oxidation | 280 | ||
12.3.3 Engineering of CYP-catalyzed Promiscuous Reactions | 283 | ||
12.4 Conclusions and Perspectives | 286 | ||
Acknowledgements | 286 | ||
References | 287 | ||
Chapter 13 - Conformational Changes in Cytochrome P450cam and the Effector Role of Putidaredoxin | 292 | ||
13.1 Introduction | 292 | ||
13.2 Conformational Change in Cytochromes P450 | 293 | ||
13.3 Conformational Change in P450cam | 294 | ||
13.4 The Unique Role of Putidaredoxin as an Effector and Electron Donor to P450cam | 296 | ||
13.5 The Importance of Solution Methods to Complement Crystallography | 296 | ||
13.6 Use of Double Electron–Electron Resonance to Measure Conformational Change in P450cam | 297 | ||
13.7 The Nature of Putidaredoxin as an Effector of Conformational Change | 298 | ||
13.8 The P450cam/Pdx Complex | 300 | ||
13.9 A Search for the Intermediate Conformation | 302 | ||
13.10 How Does Pdx Gate the Second Electron Transfer | 303 | ||
13.11 How Does Pdx Binding at the Proximal Site Communicate with the Active Site | 305 | ||
13.12 Summary | 306 | ||
Acknowledgements | 307 | ||
References | 307 | ||
Section V - Oxidases and O2-dependent nitrogen chemistry | 311 | ||
Chapter 14 - Oxygen Reduction and Proton Translocation by Respiratory Cytochrome c Oxidase | 313 | ||
14.1 Introduction | 313 | ||
14.2 The Catalytic Cycle – O2 Activation and Reduction to Water | 317 | ||
14.2.1 Oxygen Binding | 318 | ||
14.2.2 Splitting of the O–O Bond: Formation of the P and F Intermediates | 319 | ||
14.2.3 The Oxidised Enzyme – States OH and O | 320 | ||
14.2.4 Re-reduction of the Binuclear Site | 321 | ||
14.3 Proton Translocation | 321 | ||
14.3.1 Flow-flash Experiments | 321 | ||
14.3.1.1 Electron-coupled Proton Transfer | 322 | ||
14.3.1.2 The Mechanism of Proton Pumping | 322 | ||
14.3.2 Electron Injection Experiments | 323 | ||
14.3.3 Kinetic Gating of the Proton Pump | 324 | ||
14.3.4 Alternative Proton Pump Mechanisms | 326 | ||
14.4 Summary | 327 | ||
Acknowledgements | 328 | ||
References | 328 | ||
Chapter 15 - Structure and Function of Membrane-bound Bacterial Nitric Oxide Reductases | 334 | ||
15.1 NO Decomposition in Biology | 334 | ||
15.2 Bacterial Nitric Oxide Reductases | 335 | ||
15.3 Crystal Structures of the NORs | 336 | ||
15.3.1 Overall Structures | 336 | ||
15.3.2 Active Site Structures | 338 | ||
15.3.3 NO Binding Channel | 340 | ||
15.3.4 Electron Transfer Pathway | 340 | ||
15.3.5 Proton Transfer Pathway | 341 | ||
15.4 NO Reduction Mechanism | 342 | ||
15.5 Insights into the Molecular Evolution of the Respiratory Enzymes | 345 | ||
15.6 NO Dynamics Controlled by a Denitrification Protein Complex | 346 | ||
References | 346 | ||
Chapter 16 - Mechanisms of Nitric Oxide Sensing and Detoxification by Bacterial Hemoproteins | 351 | ||
16.1 Introduction | 351 | ||
16.2 NO Sensors | 352 | ||
16.2.1 Domain Architecture and Physiological Function | 353 | ||
16.2.2 Structures and Sensing Mechanisms | 354 | ||
16.3 NO to Nitrate | 357 | ||
16.4 NO to Nitrous Oxide | 361 | ||
16.5 Conclusion | 364 | ||
Acknowledgements | 364 | ||
References | 364 | ||
Subject Index | 370 |