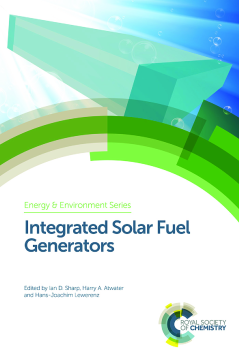
Additional Information
Book Details
Abstract
With the rapid worldwide increase of interest and excitement about the promise of artificial photosynthesis for renewable fuels, the research community is beginning to focus on the challenges of integrating the various components into complete, unassisted solar fuel generators.
Integrated Solar Fuel Generators discusses the scientific and engineering efforts addressing the challenges of building complete integrated artificial photosystems that will form the basis for developing a solar fuels technology. Building on recent substantial progress towards efficient semiconductor light absorbers and robust, earth abundant heterogeneous catalysts for water oxidation and proton reduction by the community, the integration of these components into efficient durable generators suitable for scale-up moves into focus. To succeed, a broad range of materials, processing, and design issues need to be addressed to meet efficiency, stability and scalability requirements.
This book describes the critical areas of research and development towards viable integrated solar fuels systems, the current state of the art of these efforts and outlines future research needs that will accelerate progress towards a deployable technology.
Ian D Sharp is a Staff Scientist at the Joint Center for Artificial Photosynthesis, Lawrence Berkeley National Laborator, USA.
Harry A Atwater is the Howard Hughes Professor of Applied Physics and Materials Science at the California Institute of Technology, USA.
Hans-Joachim Lewerenz is Department Head for Accelerated Discovery at the Joint Center for Artificial Photosynthesis, Lawrence Berkeley National Laborator, USA.
Table of Contents
Section Title | Page | Action | Price |
---|---|---|---|
Cover | Cover | ||
Preface | vii | ||
Contents | xi | ||
Introduction and System Considerations | 1 | ||
Chapter 1: Concepts of Photoelectrochemical Energy Conversion and Fuel Generation | 3 | ||
1.1 Introductory Remarks | 3 | ||
1.2 Semiconductor Junctions and Dark Electrochemical Processes | 5 | ||
1.2.1 Concept of the Classical Silicon Solar Cell | 5 | ||
1.2.2 The Semiconductor-redox Electrolyte Contact | 7 | ||
Electrocatalysis | 79 | ||
Chapter 3: Understanding the Effects of Composition and Structure on the Oxygen Evolution Reaction (OER) Occurring on NiFeOx Catalysts | 81 | ||
3.1 Introduction | 81 | ||
3.2 Thermodynamics of Water Splitting | 82 | ||
3.3 Catalysts for the OER | 83 | ||
3.4 The Structure of FeNiOx | 87 | ||
3.5 Identity of the Active Site in FeNiOx | 96 | ||
3.6 Factors Affecting the OER Activity of NiFeOOH | 101 | ||
3.7 Effects of Additives Other Than Fe on the OER Activity of NiMOx | 103 | ||
3.8 Effects of Additive on the OER Activity of NiFeOx | 105 | ||
3.9 Conclusions | 108 | ||
Acknowledgments | 108 | ||
References | 109 | ||
Chapter 4: Surface Science, X-ray and Electron Spectroscopy Studies of Electrocatalysis | 117 | ||
4.1 Introduction | 117 | ||
4.2 Laboratory Based Methods for Surface Characterization | 120 | ||
4.2.1 UHV-based Surface Science | 120 | ||
4.3 Synchrotron-based in situ and operando Spectroscopy | 125 | ||
4.3.1 Photon-in/photon-out Methods: Experimental Setup for operando Spectroscopy, X-ray Absorption, and High Resolution X-ray Spectroscopy | 126 | ||
4.3.1.1 Experimental Setup for operando Photon-in/photon-out Spectroscopy | 126 | ||
4.3.1.2 X-ray Absorption Spectroscopy | 128 | ||
4.3.1.3 High Resolution X-ray Spectroscopy | 130 | ||
4.3.1.4 Feasibility of High-energy XAS as operando Surface Analysis Tool | 135 | ||
4.3.2 Ambient Pressure XPS | 137 | ||
4.3.2.1 Methods: Tender X-ray APXPS | 145 | ||
4.4 Summary and Outlook | 148 | ||
References | 149 | ||
Chapter 5: Evaluating Electrocatalysts for Solar Water-splitting Reactions | 154 | ||
5.1 Introduction | 154 | ||
5.2 Experimental Considerations | 155 | ||
5.2.1 Cell Design | 155 | ||
5.2.2 Auxiliary Electrode | 156 | ||
5.2.3 Reference Electrodes | 157 | ||
5.2.4 Working Electrode Material | 159 | ||
5.2.5 Catalyst Deposition and Characterization | 160 | ||
5.3 Catalyst Performance | 161 | ||
5.3.1 Elemental Analysis | 163 | ||
5.3.2 Catalytic Activity | 163 | ||
5.3.3 Short-term Stability | 164 | ||
5.3.4 Extended Stability | 165 | ||
5.3.5 Faradaic Efficiency Measurements | 167 | ||
5.3.6 Measuring Catalyst Surface Area | 168 | ||
5.4 Benchmarking Catalyst Performance | 170 | ||
5.4.1 Primary Figure of Merit | 170 | ||
5.4.2 Comparing Electrocatalytic Performance | 172 | ||
5.5 Conclusions | 174 | ||
References | 175 | ||
Semiconductor Light Absorbers | 183 | ||
Chapter 6: Heterojunction Approaches for Stable and Efficient Photoelectrodes | 185 | ||
6.1 Introduction | 185 | ||
6.2 Semiconductor-Electrolyte Interface in the Context of Chemical Conversion | 188 | ||
6.2.1 Overview | 188 | ||
6.2.2 Simple Picture of an Unpinned Semiconductor-Liquid Junction (SLJ) | 188 | ||
6.2.3 Electrically Decoupled Photovoltaic and Catalyst | 192 | ||
6.2.4 Heterojunction Design for Stability and Efficiency | 194 | ||
6.3 JCAP Experimental Work | 194 | ||
6.3.1 Photocathodes | 194 | ||
6.3.2 Photoanodes | 197 | ||
6.4 Summary and Outlook | 205 | ||
Acknowledgments | 205 | ||
References | 205 | ||
Chapter 7: Artificial Photosynthesis with Inorganic Particles | 214 | ||
7.1 Why Particles? | 214 | ||
7.1.1 Photoreactors | 216 | ||
7.2 Absorber Configurations | 218 | ||
7.3 Stability | 219 | ||
7.4 Ideal Limiting Solar-to-hydrogen (STH) Efficiency | 220 | ||
7.5 Experimental Efficiencies | 223 | ||
7.6 Mechanism of Water Splitting Photocatalysis | 224 | ||
7.7 Free Energy of Photocatalysts | 224 | ||
7.8 Light Absorption and Exciton Generation | 225 | ||
7.9 Recombination | 227 | ||
7.9.1 Auger Recombination | 228 | ||
7.9.2 Shockley-Read-Hall Recombination | 229 | ||
7.9.3 Surface Recombination | 230 | ||
7.9.4 Radiative Recombination | 233 | ||
7.9.5 Overall Lifetime | 234 | ||
7.10 Charge Transport | 234 | ||
7.11 Charge Separation | 237 | ||
7.11.1 Junctions | 237 | ||
7.11.2 Electric Dipoles | 244 | ||
7.11.3 Ohmic Contacts | 245 | ||
7.12 Charge Transfer Reactions at the Cocatalyst-Liquid Interface | 247 | ||
7.13 Charge Transfer Reactions at Semiconductor-Liquid Interfaces | 248 | ||
7.13.1 Controlling the Back Reaction | 251 | ||
7.13.2 Photocorrosion | 252 | ||
7.13.3 Electrolyte Effects and pH | 253 | ||
7.13.4 Theoretical Modeling | 255 | ||
7.13.5 Promising Absorber Materials | 256 | ||
7.14 Conclusion | 258 | ||
Acknowledgments | 258 | ||
References | 259 | ||
Chapter 8: Degradation of Semiconductor Electrodes in Photoelectrochemical Devices: Principles and Case Studies | 281 | ||
8.1 Introduction | 281 | ||
8.2 Thermodynamic and Kinetic Requirements for Material Stability | 282 | ||
8.2.1 Thermodynamic Aspects | 282 | ||
New Materials and Components | 305 | ||
Chapter 9: High Throughput Experimentation for the Discovery of Water Splitting Materials | 307 | ||
9.1 Mission-driven Materials Discovery: Introduction and Strategies | 307 | ||
9.1.1 High Throughput Screening for Specific Device Components and Operating Conditions | 307 | ||
9.1.2 General Strategies for Constructing Experimental Screening Pipelines | 309 | ||
9.2 Cross-cutting Capabilities: Materials Synthesis and Data Management | 310 | ||
9.2.1 Inkjet Printing of Functional Metal Oxides | 311 | ||
9.2.2 Combinatorial Physical Vapor Deposition | 314 | ||
9.2.3 Thermal Processing | 316 | ||
9.2.4 Data Management | 317 | ||
9.3 Experimental Pipeline for Discovering OER Electrocatalysts | 319 | ||
9.3.1 The Scanning Droplet Cell and Its Deployment for Electrocatalyst Discovery | 319 | ||
9.3.2 Parallel Screening via Bubble Imaging | 323 | ||
9.3.3 Screening Libraries with Unstable Catalysts | 324 | ||
9.3.4 Materials Characterization for Electrocatalysts | 325 | ||
9.4 Experimental Pipeline for Discovering Photoanodes | 325 | ||
9.4.1 High Throughput Spectroscopy for Band Gap Screening | 325 | ||
9.4.2 Colorimetry as a Parallel Screen | 328 | ||
9.4.3 Photoelectrochemistry with the Scanning Droplet Cell | 329 | ||
9.4.4 Material Characterization of Photoanodes: Linking to Theory | 330 | ||
9.5 Combining Materials and Techniques for Discovery of Integrated Materials | 333 | ||
9.6 Lessons Learned and Future Prospects | 337 | ||
Acknowledgments | 337 | ||
References | 338 | ||
Chapter 10: Membranes for Solar Fuels Devices | 341 | ||
10.1 Transport Challenges in Membranes for Solar Fuels Devices | 342 | ||
10.2 Membrane Materials and Structure | 344 | ||
10.3 Commercial Membranes | 346 | ||
10.4 Transport of Solutes in Membranes | 350 | ||
10.5 Solute Sorption | 352 | ||
10.6 Solute Diffusion | 353 | ||
10.7 Water Sorption | 356 | ||
10.8 Electrical Properties | 359 | ||
10.9 Multicomponent Transport | 363 | ||
10.10 Measurement of Transport Parameters in Membranes | 365 | ||
10.11 Phenomena Affecting Transport: Physical Aging and Degradation | 369 | ||
10.12 JCAP Membrane Research | 371 | ||
10.13 Outlook for Membranes in CO2 Reduction Devices | 376 | ||
List of Symbols | 377 | ||
References | 379 | ||
Devices and Modelling | 387 | ||
Chapter 11: Prototyping Development of Integrated Solar-driven Water-splitting Cells | 389 | ||
11.1 Introduction | 389 | ||
11.2 Materials and Components | 391 | ||
11.2.1 Selection and Design Consideration of Light Absorber Materials | 391 | ||
11.2.1.1 Triple-junction Amorphous Silicon | 392 | ||
11.2.1.2 Monolithic Tandem and Triple-junction Crystalline Silicon | 394 | ||
11.2.1.3 Compound Semiconductor Multi-junction Photovoltaics | 396 | ||
11.2.2 Selection and Design Consideration of Electrolytes | 398 | ||
11.2.2.1 Electrolyte Effect on Transport Losses in a Device | 399 | ||
11.2.2.2 Electrolyte Effect on the Stability of Semiconducting Light Absorbers | 401 | ||
11.2.2.3 Electrolyte Effect on Catalytic Activity, Stability and Optical Transmittance | 402 | ||
11.2.2.3.1 Effect of Unintentional Cation and Anion in Electrolyte on the Catalytic Activity | 402 | ||
11.2.2.3.2 Electrolyte Effect on Activity and Stability | 403 | ||
11.2.2.3.3 Electrolyte Effect on Light Absorption | 404 | ||
11.2.2.3.4 Electrolyte Effect on Electrochromism of Electrocatalysts | 406 | ||
11.2.3 Incorporation of Membrane Separators | 407 | ||
11.2.3.1 Mechanical Compression | 408 | ||
11.2.3.2 Adhesion | 409 | ||
11.2.3.3 Others | 411 | ||
11.2.4 Chassis and Auxiliary Components | 413 | ||
11.2.5 Integration of Protective Layers with Catalysts and Light Absorbers | 415 | ||
11.3 Full Device Characterization and Evaluation | 427 | ||
11.3.1 Different Sources of Lights and Calibration Methods | 427 | ||
11.3.2 Product Collection | 429 | ||
11.3.2.1 Experimental Setup | 429 | ||
11.3.2.2 Measurements | 430 | ||
11.3.2.3 Analysis | 431 | ||
11.3.3 Outdoor Testing | 431 | ||
11.4 System Engineering Approaches | 434 | ||
11.4.1 Introduction to System Engineering | 434 | ||
11.4.2 Development of Hierarchical Requirements | 435 | ||
11.4.3 Testing Plan | 436 | ||
11.4.4 Reviews | 440 | ||
11.5 Conclusion and Outlook | 442 | ||
References | 443 | ||
Chapter 12: High-efficiency Water Splitting Systems | 454 | ||
12.1 The Need for High Efficiency in Solar Fuel Generation | 454 | ||
12.2 Efficiency Limitations and Prospects for Photoelectrochemical Energy Conversion | 456 | ||
12.2.1 Fundamental Limitations: Detailed Balance Limit and Catalysis | 456 | ||
12.2.2 Further Relevant Loss Mechanisms and Mitigation Strategies | 460 | ||
12.2.2.1 Absorption by Electrolyte and Catalyst | 460 | ||
12.2.2.2 Ohmic Resistivity | 462 | ||
12.2.2.3 Non-radiative Recombination | 463 | ||
12.2.2.4 Energetics | 463 | ||
12.2.2.5 Light Management | 464 | ||
12.2.2.6 Gas Bubble Management | 465 | ||
12.2.2.7 Multi-terminal Approaches | 466 | ||
12.2.2.8 Concentration | 466 | ||
12.3 III-V Semiconductor Tandem Structures: A Testbed for High Efficiency | 467 | ||
12.3.1 History of the III-V Compound Semiconductors and High-efficiency Solar Cells | 467 | ||
12.3.2 Highly Efficient III-V Tandem Structures in Solar Water Splitting | 469 | ||
12.3.2.1 Classical III-V Tandem Photoelectrochemistry | 469 | ||
12.3.2.2 Metamorphic Device Concepts | 469 | ||
12.3.2.3 Inverted Metamorphic Device Concepts | 473 | ||
12.4 Efficiency Measurement and Characterization Strategies | 480 | ||
12.4.1 Standard Solar Irradiance vs. Laboratory Light Sources | 480 | ||
12.4.2 Tandem Device Characterization: A Case Study on Common Practice vs. Result Validation | 483 | ||
12.4.3 Utilization of Natural Sunlight and Secondary Illumination Errors | 486 | ||
12.4.4 Differential Spectral Responsivity | 492 | ||
12.4.5 Solar-to-hydrogen Conversion Reference Laboratories | 493 | ||
12.5 Summary and Outlook | 494 | ||
Acknowledgments | 495 | ||
References | 495 | ||
Chapter 13: Continuum-scale Modeling of Solar Water-splitting Devices | 500 | ||
13.1 Introduction | 500 | ||
13.1.1 Definitions | 503 | ||
13.2 Modeling Methodology and Approach | 505 | ||
13.2.1 Model Dimensionality | 508 | ||
13.2.2 History of Modeling within JCAP | 510 | ||
13.3 Governing Equations | 511 | ||
13.3.1 Transport in Electrolytes | 513 | ||
13.3.1.1 Mass Conservation | 513 | ||
13.3.1.2 Acid-Base Equilibria | 513 | ||
13.3.1.3 Mass-species Fluxes | 514 | ||
13.3.1.3.1 Infinitely Dilute Electrolyte | 514 | ||
13.3.1.3.2 Moderately Dilute Electrolyte | 515 | ||
13.3.1.3.3 Concentrated Electrolyte | 515 | ||
13.3.1.4 Membrane Transport | 516 | ||
13.3.1.5 Charge Transport and Conservation | 517 | ||
13.3.1.6 Electron Transport | 518 | ||
13.3.1.7 Product Gases | 518 | ||
13.3.1.8 Momentum Conservation | 519 | ||
13.3.2 Transport in Photoabsorbers | 520 | ||
13.3.2.1 Light Capture | 520 | ||
13.3.2.2 Hole and Electron Transport | 522 | ||
13.3.2.3 Diode Equation | 522 | ||
13.3.3 Kinetics | 523 | ||
13.3.4 Heat Transfer | 524 | ||
13.4 Sample Cases | 526 | ||
13.4.1 Simplifications to the Flux-expressions | 526 | ||
13.4.1.1 Shockley-Queisser | 527 | ||
13.4.1.2 Butler-Volmer | 527 | ||
13.4.1.3 Strongly Acidic or Alkaline Electrolytes | 527 | ||
13.4.1.4 Supporting Electrolyte | 527 | ||
13.4.1.5 Buffered Electrolyte | 527 | ||
13.4.1.6 1-D Approximation to 2-D and 3-D Solar-fuels Generator | 528 | ||
13.4.2 Case Study 1: Design Guidelines and Cell Scaling from 2D PEC Device Models | 528 | ||
13.4.3 Case Study 2: Multiphysics Modeling in Concentrated PEC | 531 | ||
13.5 Summary | 534 | ||
Acknowledgments | 535 | ||
References | 535 | ||
Subject Index | 537 |