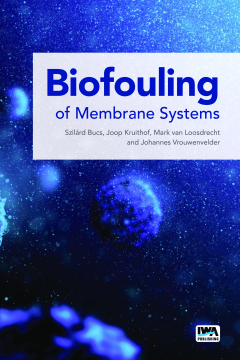
BOOK
Biofouling of Membrane Systems
Szilárd Bucs | Joop Kruithof | Mark C. M. van Loosdrecht | Johannes Simon Vrouwenvelder
(2018)
Additional Information
Book Details
Abstract
Because of the uneven distribution of fresh water in time and space and the increasing human population, a large number of regions are experiencing water scarcity and stress. Membrane-based desalination technologies like reverse osmosis have the potential to solve the fresh water crisis in coastal areas. However, in many cases membrane
performance is restricted by biofouling.
Biofouling of Membrane Systems gives a comprehensive overview on the state of the art strategies to control biofouling in spiral wound reverse osmosis membrane systems and point to possible future research directions. Despite the fact that much research and development has been done to overcome biofouling in spiral wound membrane systems used for water treatment, biofouling is still a major practical problem causing performance decline and increased energy demand. Biofouling of Membrane Systems is divided into three sections including modelling and numerical analysis, non-destructive characterization and feed spacer geometry optimization. It focuses on the development of biomass in the feed channel of the membrane module and its effect on pressure drop
and hydrodynamics.
This book can be used to develop an integral strategy to control biofouling in spiral wound membrane systems. An overview of several potential complementary approaches to solve biofouling is given and an integrated approach for biofouling control and feed spacer design is proposed.
Table of Contents
Section Title | Page | Action | Price |
---|---|---|---|
Cover | Cover | ||
Contents | v | ||
Preface | xiii | ||
List of authors | xv | ||
Chapter 1: New approaches to characterizing and understanding biofouling of spiral wound membrane systems | 1 | ||
INTRODUCTION | 1 | ||
BIOFOULING STUDIES | 2 | ||
RECENT MICROBIOLOGICAL RESEARCH INTO CHARACTERIZING BIOFOULING | 3 | ||
FLOW CELL STUDIES | 5 | ||
MATHEMATICAL MODELLING | 6 | ||
CONCLUSION | 8 | ||
Chapter 2: Effect of flow velocity substrate concentration and hydraulic cleaning on biofouling of reverse osmosis feed channels | 9 | ||
INTRODUCTION | 9 | ||
BIOFOULING MODEL DESCRIPTION | 10 | ||
Model assumptions and equations | 11 | ||
Model solution | 13 | ||
RESULTS AND DISCUSSION | 14 | ||
Effect of cross-flow velocity on biofilm development in the feed channel | 14 | ||
Biofilm influence on the permeate flux and pressure drop | 17 | ||
Hydraulic membrane cleaning | 19 | ||
Effect of inlet substrate concentration on biofouling | 20 | ||
Reproducibility of biofouling simulations | 24 | ||
CONCLUSIONS | 26 | ||
Chapter 3: Modelling the effect of biofilm formation on reverse osmosis performance: flux, feed channel pressure drop and solute passage | 29 | ||
INTRODUCTION | 29 | ||
MODEL DEVELOPMENT | 31 | ||
Model geometry | 31 | ||
Model equations | 34 | ||
Fluid flow | 34 | ||
Mass balances | 37 | ||
Biofilm sub-model | 38 | ||
Model parameters | 39 | ||
Model solution | 40 | ||
RESULTS | 43 | ||
Biofilm development in the feed channel | 43 | ||
Biofilm formation | 43 | ||
Substrate distribution | 45 | ||
Biomass detachment | 46 | ||
Effect of biofouling on global membrane performance | 46 | ||
Permeate flux | 48 | ||
Salt passage | 49 | ||
Local effects of biofouling | 50 | ||
Concentration polarization | 50 | ||
Hydraulic resistance | 51 | ||
Feed channel pressure drop | 54 | ||
DISCUSSION | 55 | ||
Concentration polarization and biofilm permeability | 57 | ||
Spacer importance | 58 | ||
Further model development and use | 59 | ||
CONCLUSIONS | 59 | ||
Chapter 4: Combined biofouling and scaling in membrane feed channels a new modelling approach | 61 | ||
INTRODUCTION | 61 | ||
MODEL DESCRIPTION | 63 | ||
Geometry, phases, computational domains | 63 | ||
Fluid flow and solute transport | 64 | ||
Fluid flow | 64 | ||
Solute transport | 65 | ||
Foulant development | 69 | ||
Initiation of fouling, attachment and nucleation | 70 | ||
Growth of foulant layer | 70 | ||
Detachment | 71 | ||
Model solution | 71 | ||
RESULTS | 73 | ||
Dynamics of combined fouling in the feed channel | 73 | ||
Biofilm enhanced concentration polarization and scaling | 75 | ||
Effect of combined fouling on process performance | 77 | ||
Parameter sensitivity studies | 79 | ||
DISCUSSION | 80 | ||
Complexity of biofilm–precipitate interactions | 80 | ||
Benefits, limitations and extensions of the model | 82 | ||
CONCLUSIONS | 83 | ||
Chapter 5: Effect of different commercial feed spacers on biofouling of reverse osmosis membrane systems a numerical study | 85 | ||
INTRODUCTION | 85 | ||
MODEL DESCRIPTION | 86 | ||
Spacer geometry | 87 | ||
Computational domain | 88 | ||
Liquid flow, substrate transport and biofilm formation | 89 | ||
Liquid flow | 90 | ||
Substrate transport and reaction | 90 | ||
Biofilm formation | 91 | ||
Model solution | 92 | ||
RESULTS | 92 | ||
Effect of linear flow velocity | 92 | ||
Effect of bacterial cell load | 96 | ||
Biomass location | 96 | ||
Spacer geometry | 98 | ||
Spacer shape and channel porosity | 99 | ||
Spacer thickness | 102 | ||
DISCUSSION | 102 | ||
Numerical model evaluation | 104 | ||
Importance of feed spacer for biofouling | 104 | ||
CONCLUSIONS | 105 | ||
Chapter 6: In-situ biofilm characterization in membrane systems using Optical Coherence Tomography: Formation, structure, detachment and impact of flux change | 107 | ||
INTRODUCTION | 107 | ||
MODEL DESCRIPTION | 109 | ||
Experimental set-up | 109 | ||
Experiments and operational conditions | 111 | ||
Effect of permeate flux variations | 112 | ||
OCT and biofilm image data processing | 112 | ||
RESULTS | 113 | ||
Biofilm development | 113 | ||
Biofilm detachment | 115 | ||
Effect of permeate flux variation on biofilm thickness and resistance | 116 | ||
DISCUSSION | 119 | ||
Suitability of Optical Coherence Tomography | 119 | ||
Biofilm compaction | 120 | ||
Biofilm structures | 122 | ||
Perspective of OCT studies | 122 | ||
Application aspects | 123 | ||
CONCLUSIONS | 123 | ||
Chapter 7: Early non-destructive biofouling detection and spatial distribution: Application of oxygen sensing optodes | 125 | ||
INTRODUCTION | 125 | ||
Biofouling in NF/RO membranes | 125 | ||
Planar optodes | 126 | ||
MATERIALS AND METHODS | 127 | ||
Experimental setup description | 127 | ||
Operating condition | 128 | ||
Dye and optode description | 129 | ||
Imaging system | 129 | ||
Image calculation and analysis | 130 | ||
Analysis of spatial indicators | 131 | ||
Cross-flow versus stop-flow imaging | 131 | ||
RESULTS | 132 | ||
Pressure drop | 135 | ||
Oxygen concentration during cross-flow MFS operation | 136 | ||
Oxygen concentration during stop-flow MFS operation | 137 | ||
Comparison of the three methods | 138 | ||
DISCUSSION | 140 | ||
Early detection of biofouling development | 140 | ||
Spatially resolved biofouling development information | 141 | ||
Effect of stop-flow imaging on biofouling development | 142 | ||
Practical applications and future studies | 143 | ||
CONCLUSIONS | 143 | ||
Chapter 8: Chemical cleaning of biofouling in reverse osmosis membranes evaluated using magnetic resonance imaging | 145 | ||
INTRODUCTION | 145 | ||
MATERIAL AND METHODS | 147 | ||
Flow cells | 147 | ||
Fouling | 147 | ||
Cleaning | 148 | ||
MRI | 148 | ||
RESULTS AND DISCUSSIONS | 149 | ||
Fouling and image analysis | 149 | ||
Different cleaning solutions | 152 | ||
Cleaning mechanism | 154 | ||
Effect of fouling time | 157 | ||
Link to feed channel pressure drop | 158 | ||
CONCLUSIONS | 159 | ||
Chapter 9: Early non-destructive biofouling detection in spiral wound RO membranes using a mobile earth’s field NMR | 161 | ||
INTRODUCTION | 161 | ||
BACKGROUND | 163 | ||
Relevant nuclear magnetic resonance (NMR) theory | 163 | ||
NMR and biofilms | 164 | ||
MATERIALS AND METHODOLOGY | 165 | ||
NMR methods employed | 165 | ||
RO membrane module and test conditions | 167 | ||
RESULTS AND DISCUSSION | 169 | ||
Visualisation of final fouled module | 169 | ||
EF NMR relaxation measurements | 172 | ||
Spin-echo measurements of total NMR signal | 174 | ||
Practical implications and further studies | 176 | ||
Potential field of EF NMR use | 176 | ||
Measurement of membrane element fouling in pressure vessels during operation | 176 | ||
Further research | 177 | ||
CONCLUSIONS | 177 | ||
Chapter 10: Experimental and numerical characterization of the water flow in spacer-filled channels of spiral-wound membranes | 179 | ||
INTRODUCTION | 179 | ||
MATERIAL AND METHODS | 181 | ||
Experimental | 181 | ||
Flow cell | 181 | ||
Particles | 183 | ||
Visualization | 183 | ||
Calculation and visualization of velocity fields | 185 | ||
Numerical model | 185 | ||
Calculation and visualization of velocity fields | 186 | ||
Statistic similarity | 187 | ||
RESULTS | 187 | ||
Flow pattern change over channel height | 187 | ||
Spatial reproducibility | 189 | ||
Steady and unsteady flow – effect of different flow velocities | 189 | ||
Measurements compared with CFD model | 193 | ||
DISCUSSION | 195 | ||
Flow regimes | 196 | ||
Solute transfer | 197 | ||
CFD model | 197 | ||
Further studies | 198 | ||
CONCLUSIONS | 198 | ||
Chapter 11: Spacer geometry and particle deposition in spiral wound membrane feed channels | 199 | ||
INTRODUCTION | 199 | ||
MATERIAL AND METHODS | 201 | ||
Experiments | 201 | ||
Flow cells and cross-flow setup | 201 | ||
Membranes, spacer and particles | 203 | ||
Fouling protocol | 204 | ||
Imaging | 204 | ||
Numerical model | 204 | ||
Model geometry | 205 | ||
Fluid flow calculations | 205 | ||
Particle trajectory and deposition | 206 | ||
Model solution | 207 | ||
RESULTS | 209 | ||
Development of deposition pattern in time | 210 | ||
Effect of feed spacer orientation on particle deposition | 210 | ||
Diamond orientation | 210 | ||
Ladder orientation | 216 | ||
Effect of cross-flow velocity | 216 | ||
Effect of permeate production | 218 | ||
DISCUSSION | 220 | ||
Importance of hydrodynamic conditions and spacer geometry | 221 | ||
Spacer geometry and orientation | 221 | ||
Flow velocity | 222 | ||
Permeation effects | 222 | ||
Implications for practice | 223 | ||
Further studies | 224 | ||
CONCLUSIONS | 225 | ||
Chapter 12: Characterization of feed channel spacer performance using geometries obtained by X-ray computed tomography | 227 | ||
INTRODUCTION | 227 | ||
MATERIAL AND METHODS | 230 | ||
Spacer types | 230 | ||
Spacer geometry acquisition and processing | 230 | ||
X-ray computed tomography procedure and surface triangulation | 231 | ||
Processing of triangulated surfaces | 233 | ||
Surface fitting and conversion to a solid shape | 234 | ||
Construction of computational domain | 234 | ||
Spacer geometry measurements | 234 | ||
Construction of simplified spacer geometry | 234 | ||
Numerical model | 235 | ||
Model solution and mesh convergence studies | 236 | ||
Experimental methods | 236 | ||
Measures for evaluation of different spacer geometries | 236 | ||
Pressure drop | 236 | ||
Friction factor | 237 | ||
Membrane shear stress generated per power input | 237 | ||
Membrane shear stress efficiency for a constant flow rate | 238 | ||
Strand eccentricity | 238 | ||
RESULTS | 238 | ||
Geometric characteristics of feed channel spacers from CT scans | 238 | ||
Impact of resolution on geometry characteristics | 239 | ||
Hydraulic characterization | 243 | ||
Pressure drop measurements compared to model solutions using CT scans | 243 | ||
Friction from strand shape and orientation | 245 | ||
Shear at the membrane and power input | 246 | ||
Spatial velocity distribution | 248 | ||
Analysis of simplified model geometries | 250 | ||
DISCUSSION | 250 | ||
Feed channel spacer geometry | 250 | ||
Pressure drop measurements compared to model solutions using CT scans | 251 | ||
Friction due to strand shape and orientation | 251 | ||
Local membrane shear distribution | 252 | ||
Power input with respect to friction | 252 | ||
Spatial velocity distribution | 253 | ||
Mechanical deformation and membrane imprinting | 254 | ||
Further studies | 254 | ||
CONCLUSIONS | 255 | ||
Chapter 13: Development and characterization of 3D-printed feed spacers for spiral wound membrane systems | 257 | ||
INTRODUCTION | 257 | ||
MATERIAL AND METHODS | 260 | ||
Numerical modelling | 260 | ||
3D printed feed spacers | 261 | ||
Microscopic observation | 262 | ||
Experimental set up | 263 | ||
Operating conditions | 264 | ||
Sampling and biomass analyses | 264 | ||
RESULTS | 265 | ||
Numerical modeling and 3D printing of feed spacers | 265 | ||
Comparison of feed spacer from practice and 3D printed spacer with same geometry | 265 | ||
Comparison of feed spacer from practice and 3D printed spacer with modified geometry | 266 | ||
DISCUSSION | 270 | ||
3D printing technique to design modified geometry feed spacers | 271 | ||
A novel strategy to modify feed spacer geometry | 272 | ||
Suggestions for future studies | 274 | ||
CONCLUSIONS | 275 | ||
Chapter 14: Strategies for biofouling mitigation in spiral wound membrane systems | 277 | ||
INTRODUCTION | 277 | ||
Biofouling control strategies | 280 | ||
Pre-treatment by water filtration and bacterial inactivation | 280 | ||
Membrane modification | 281 | ||
Feed spacer and hydrodynamics | 282 | ||
Spiral wound membrane modules cleaning strategies | 283 | ||
FUTURE RESEARCH DIRECTIONS | 284 | ||
Biofilm structural characterization | 284 | ||
Biofilm mechanical characterization | 285 | ||
Biofouling mitigation strategies | 287 | ||
Membrane and spacer surface modification | 287 | ||
New spacer designs | 287 | ||
Numerical evaluation | 289 | ||
Membrane cleaning strategies | 289 | ||
CONCLUSIONS | 290 | ||
References | 293 | ||
Index | 321 |