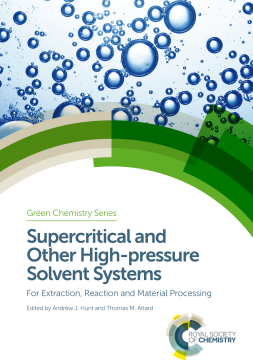
Additional Information
Book Details
Abstract
Exploring the range and utility of high-pressure solvent systems across a variety of different chemical applications, this book brings together recent advances in supercritical technology and other pressurised-solvent systems. It provides an in-depth overview of the latest advances and developments and discusses the limitations and drawbacks that need to be addressed. Wherever possible, the greenness and economic viability of the different solvent systems is highlighted. This book is ideal for researchers and industrialists working in environmental science, green chemistry and biorefineries.
Table of Contents
Section Title | Page | Action | Price |
---|---|---|---|
Cover | Cover | ||
Supercritical and Other High-pressure Solvent Systems: For Extraction, Reaction and Material Processing | i | ||
Preface | vii | ||
Contents | ix | ||
Chapter 1 - Introduction to High-pressure Solvent Systems | 1 | ||
1.1 Green Chemistry | 1 | ||
1.2 Supercritical Fluids | 3 | ||
1.2.1 Supercritical Fluids: Physical Properties | 6 | ||
1.2.2 Supercritical Carbon Dioxide | 7 | ||
1.2.3 Subcritical and Supercritical Water | 8 | ||
1.2.4 Pressurised Solvent Systems | 9 | ||
1.3 Conclusions | 11 | ||
References | 11 | ||
Chapter 2 - Understanding Entrainer Effects in Supercritical CO2 | 14 | ||
2.1 Introduction | 14 | ||
2.1.1 Increasing Solubility | 14 | ||
2.2 The Key Ideas | 16 | ||
2.2.1 Too Many (Non-)Explanations | 16 | ||
2.2.2 The Right Explanation | 17 | ||
2.2.3 Getting the Numbers | 18 | ||
2.2.4 How Not to Do It | 19 | ||
2.3 The Difficulty of Prediction | 20 | ||
2.3.1 What Gives Large Gu2 Values | 21 | ||
2.3.2 Modelling the Baseline Solubility in Pure scCO2 via the Chrastil Formula | 21 | ||
2.3.3 Linear Enhancement Factor per Entrainer Concentration (the Sovová kav Factor) | 24 | ||
2.3.4 Database of Linear Solubility Enhancement Factors | 26 | ||
2.3.5 From the Linearised Sovová Enhancement Factor Model to KBIs | 26 | ||
2.3.6 Entrainer Effect Viewed from kav | 31 | ||
2.3.7 As Good as It Gets | 32 | ||
2.4 A Plausible Theory | 33 | ||
2.5 Conclusions | 36 | ||
References | 37 | ||
Chapter 3 - Supercritical Carbon Dioxide Extraction of Lipophilic Molecules | 40 | ||
3.1 Introduction | 40 | ||
3.2 Solvent Extraction Problems | 41 | ||
3.3 Supercritical Fluid Extraction | 45 | ||
3.3.1 Choosing the Appropriate Operating Conditions for Extraction | 45 | ||
3.3.2 Pressure and Temperature | 46 | ||
3.3.3 Density and Solvent Power | 47 | ||
3.3.4 Use of Modifiers/Co-solvents | 48 | ||
3.3.5 Flow Rate | 48 | ||
3.3.6 Particle Size | 48 | ||
3.3.7 Optimisation of scCO2 Extraction Processes: Experimental Design | 49 | ||
3.3.8 Screening Experimental Designs | 50 | ||
3.3.9 Two-level Factorial Design | 51 | ||
3.3.10 Two-level Full Factorial Design | 51 | ||
3.3.11 Two-level Fractional Factorial Design | 52 | ||
3.3.12 Two-level Plackett–Burman Design | 53 | ||
3.3.13 Optimisation Design | 53 | ||
3.3.14 Taguchi Design | 54 | ||
3.3.15 Star Design | 55 | ||
3.3.16 Central Composite Design | 55 | ||
3.3.17 Box–Behnken Design (BBD) | 55 | ||
3.4 Supercritical Extraction of Waxes and Bioactive Compounds | 57 | ||
3.4.1 Bioactive Compounds | 62 | ||
3.5 scCO2 Fractionation | 65 | ||
3.6 scCO2 Extraction of Lipids as Part of a Holistic Biorefinery | 67 | ||
3.7 Supercritical Extraction in Industry: Recent Advances in the Past Few Years | 68 | ||
3.8 Conclusion | 70 | ||
References | 70 | ||
Chapter 4 - Subcritical Water: Current Status, Advances, and Applications for Extractions, Reactions and Separations | 77 | ||
4.1 Introduction | 77 | ||
4.2 Subcritical Water | 78 | ||
4.3 Use of Subcritical Water: Current Status | 80 | ||
4.4 Water for Extractions, Reactions and Separations | 83 | ||
4.4.1 Extractions | 83 | ||
4.4.1.1 Phenolic Compounds | 83 | ||
4.4.1.2 Carbohydrates | 85 | ||
4.4.1.3 Other Compounds | 88 | ||
4.4.2 Mass Transfer During Extraction | 90 | ||
4.4.2.1 Main Extraction Parameters | 92 | ||
4.4.2.1.1\rSolubility (Thermodynamic State).The high solubility of a solute in a solvent indicates that less amount of solvent is needed to... | 92 | ||
4.4.2.1.2\rSolvent Flow Rate.The solvent flow rate parameter is directly associated with the mass transfer mode when the flow is large enou... | 93 | ||
4.4.2.1.3\rProperties of Plant Matrices.The knowledge of the raw material characteristics and the solute is crucial for efficient extractio... | 93 | ||
4.4.3 Separations and Decompositions | 94 | ||
4.4.4 Reactions | 95 | ||
4.5 Economic Issues of Subcritical Water Processes | 97 | ||
4.6 Patents Survey | 99 | ||
4.7 Conclusions and Future Perspectives | 99 | ||
Acknowledgements | 101 | ||
References | 101 | ||
Chapter 5 - Supercritical Fluid Chromatography (SFC) | 106 | ||
5.1 Introduction | 106 | ||
5.2 Early Packed Columns | 107 | ||
5.3 SFC Instrumentation | 108 | ||
5.4 Mobile Phase | 108 | ||
5.5 Additives | 109 | ||
5.6 Solid Phase | 110 | ||
5.7 Detectors | 112 | ||
5.7.1 Detectors: Mobile Phase | 112 | ||
5.7.1.1 Mobile Phase – No Modifier | 112 | ||
5.7.1.2 Mobile Phase – Modifier | 112 | ||
5.7.1.3 SFC-MS | 114 | ||
5.7.2 Detectors: Type of Extract | 115 | ||
5.8 Two-dimensional SFC (2D SFC) | 115 | ||
5.9 Polymer Separations | 117 | ||
5.9.1 Polystyrene | 117 | ||
5.9.2 Polyethylene Glycol (PEG) | 119 | ||
5.9.3 Polyprenol | 119 | ||
5.10 Natural Product Separations | 120 | ||
5.10.1 Complex Lipid Systems | 120 | ||
5.10.2 Triacylglycerols (TAGs) | 121 | ||
5.10.3 Carotenoids | 122 | ||
5.10.4 Terpenes | 122 | ||
5.10.5 Alkaloids | 124 | ||
5.10.6 Phenolic Compounds | 125 | ||
5.10.6.1 Coumarins | 126 | ||
5.10.6.2 Cannabinoids | 126 | ||
5.10.6.3 Anthraquinones | 126 | ||
5.10.6.4 Flavonoids | 127 | ||
5.11 Green Credentials | 128 | ||
5.12 Conclusion | 128 | ||
References | 128 | ||
Chapter 6 - Heterogeneous Catalysis in Supercritical Carbon Dioxide | 132 | ||
6.1 Introduction | 132 | ||
6.2 Reactions over Heterogeneous Catalysts | 137 | ||
6.2.1 Hydrogenation | 137 | ||
6.2.1.1 The –C=O Bond of α,β-unsaturated Aldehydes | 138 | ||
6.2.1.2 Conjugated and Isolated –C=C Bonds | 147 | ||
6.2.1.3 Nitrile to Amine | 151 | ||
6.2.1.4 Nitroaromatics | 153 | ||
6.2.1.5 Aromatic Ring Hydrogenation | 156 | ||
6.2.2 Oxidation | 159 | ||
6.2.2.1 Alcohol Oxidation | 160 | ||
6.2.2.2 Other Oxidising Agents | 165 | ||
6.2.3 Hydrogenolysis | 168 | ||
6.2.3.1 Hydrogenolysis of the Ethereal C–O Bond | 168 | ||
6.2.3.2 Hydrogenolysis of the Carbonyl C–O Bond | 173 | ||
6.2.3.3 Hydrogenolysis of the Carbonyl C–O Bond of Tetrahydrofurfuryl Alcohol | 174 | ||
6.2.4 Dehydrogenation of Alcohols | 176 | ||
6.2.5 Current Status of Some Industrially Important Reactions | 178 | ||
6.2.5.1 Alkylation | 178 | ||
6.2.5.2 Hydroformylation | 181 | ||
6.2.5.3 Reductive Amination | 182 | ||
6.3 Conclusion | 183 | ||
References | 184 | ||
Chapter 7 - Biocatalysis in Supercritical and Liquid Carbon Dioxide and Carbon Dioxide-expanded Liquids | 191 | ||
7.1 Introduction | 191 | ||
7.1.1 Supercritical and Liquid CO2 | 192 | ||
7.1.2 CO2-expanded Liquids | 193 | ||
7.2 Biocatalyst Behaviour in High-pressure CO2-related Solvents | 194 | ||
7.2.1 Nature of Enzymes | 195 | ||
7.2.2 Effect of Water Content | 195 | ||
7.2.3 Solubility of Enzymes and Coenzymes | 197 | ||
7.2.4 Carbamate and Carbonic Acid Formation | 198 | ||
7.2.5 Thermodynamic Equilibrium of Reactions | 199 | ||
7.2.6 Effect of Temperature and Pressure | 200 | ||
7.2.7 Effect of Changes in the Solvent Properties Caused by Tuning the Pressure and Temperature | 201 | ||
7.3 Biocatalytic Reactions in Liquid and Supercritical CO2 | 203 | ||
7.3.1 Lipase-catalysed (Trans)Esterification, Hydrolysis and Polymerisation | 203 | ||
7.3.2 Galactosidase-catalysed Transgalactosylation | 206 | ||
7.3.3 Alcohol Dehydrogenase-catalysed Reduction | 207 | ||
7.3.4 Carboxylase-catalysed Carboxylation | 207 | ||
7.4 Biocatalytic Reactions in CO2-expanded Liquids | 208 | ||
7.4.1 Conventional Organic Liquids as the Solvent Component | 208 | ||
7.4.2 Green Liquids as the Solvent Component: Bio-based Liquids | 210 | ||
7.4.3 Green Liquids as the Solvent Component: Water | 212 | ||
7.4.4 Green Liquids as the Solvent Component: Ionic Liquids | 215 | ||
7.5 Conclusions | 215 | ||
References | 216 | ||
Chapter 8 - Selective Hydrogenation in Carbon Dioxide-dissolved Expanded Liquid Phases | 221 | ||
8.1 Introduction | 221 | ||
8.2 Hydrogenation of α,β-unsaturated Carbonyl Compounds | 223 | ||
8.2.1 Cinnamaldehyde | 223 | ||
8.2.2 Citral | 230 | ||
8.2.3 Furfural and Furfuryl Alcohol Analogues | 231 | ||
8.2.4 Isophorone Analogues | 234 | ||
8.2.5 Maleic Anhydride | 236 | ||
8.3 Hydrogenation of Nitro Compounds | 237 | ||
8.3.1 Hydrogenation in CO2-dissolved Expanded Substrates | 238 | ||
8.3.2 Hydrogenation in CO2-dissolved Expanded Liquid Solvents | 242 | ||
8.4 Hydrogenation of Nitrile Compounds | 245 | ||
8.5 Hydrogenation of Aromatic Rings, Alkenes, Alkynes and Other Compounds | 250 | ||
8.5.1 Hydrogenation of Phenol | 250 | ||
8.5.2 Hydrogenation of Biphenyl, Naphthalene and Tetralin | 252 | ||
8.5.3 Hydrogenation of Alkenes and Alkynes | 253 | ||
8.5.4 Hydrogenation of Other Compounds | 255 | ||
8.6 Conclusion | 255 | ||
Acknowledgements | 256 | ||
References | 256 | ||
Chapter 9 - Electrochemistry in Supercritical Fluids | 262 | ||
9.1 Introduction | 262 | ||
9.2 A Brief Historical Review of Electrochemistry in Supercritical Fluids | 263 | ||
9.3 Supercritical Fluids | 264 | ||
9.3.1 Polar Supercritical Fluids | 270 | ||
9.3.2 Supercritical CO2 | 270 | ||
9.3.3 Supercritical Hydrofluorocarbons | 271 | ||
9.4 Electrolytes | 272 | ||
9.4.1 Phase Behaviour | 272 | ||
9.4.2 Conductivity of SCF Electrolytes | 272 | ||
9.4.3 Viscosity | 280 | ||
9.5 Electrodes | 280 | ||
9.6 High Pressure and Temperature Systems for Supercritical Fluid Electrochemistry | 282 | ||
9.7 Electrochemistry | 291 | ||
9.7.1 The Double Layer in SCF Electrolytes | 291 | ||
9.7.2 Electrode Kinetics and Mass Transport | 292 | ||
9.8 Applications of Supercritical Fluid Electrochemistry | 295 | ||
9.8.1 Electrodeposition | 295 | ||
9.8.2 Electrosynthesis | 297 | ||
9.9 Conclusions | 297 | ||
Acknowledgements | 298 | ||
References | 298 | ||
Chapter 10 - Materials Processing and Recycling with Near- and Supercritical CO2-based Solvents | 304 | ||
10.1 Introduction | 304 | ||
10.2 Thermodynamics and Phase Behaviour Data for CO2-based Solvents | 306 | ||
10.2.1 Equation of State for the Estimation of the Vapour–Liquid Equilibrium and Thermodynamic Properties | 308 | ||
10.2.2 Solubility of Reagents | 310 | ||
10.3 Organic Materials Processing Using CO2-based Systems | 311 | ||
10.3.1 Required Equipment | 312 | ||
10.3.1.1 Pumps | 312 | ||
10.3.1.2 Contactor | 313 | ||
10.3.1.3 Precipitation Vessel | 313 | ||
10.3.1.4 Back-pressure Regulator | 313 | ||
10.3.1.5 Particle Separator | 314 | ||
10.3.1.6 Fluid Separator | 314 | ||
10.3.2 Principles of Processes Using CO2 for Organic Material Precipitation | 314 | ||
10.3.2.1 CO2 as a Solvent | 314 | ||
10.3.2.2 CO2 as an Antisolvent | 316 | ||
10.3.2.3 CO2 as a Solute | 318 | ||
10.4 Inorganic Materials Processing Using CO2-based Systems | 320 | ||
10.4.1 Continuous Synthesis of Inorganic Nanostructures in CO2-based Solvents | 321 | ||
10.4.2 Deposition of Films with a Supercritical Fluid Chemical Deposition Process in a Cold Wall Reactor | 323 | ||
10.4.3 Surface Engineering of Materials in CO2-based Solvents | 326 | ||
10.5 Materials Recycling with Supercritical CO2 | 327 | ||
10.5.1 LIB Electrolyte Recovery | 328 | ||
10.5.2 Heavy Metal Extraction | 330 | ||
10.5.3 Polymer Recycling, Case Study of PS | 331 | ||
10.5.4 New Recycling Procedures based on the Use of scCO2 | 331 | ||
10.5.4.1 Supercritical CO2 Leaching | 331 | ||
10.5.4.2 Supercritical CO2 Delamination | 332 | ||
10.6 Conclusions | 333 | ||
Acknowledgements | 334 | ||
References | 334 | ||
Chapter 11 - Solubility and Synthesis of Polymers Using Supercritical Carbon Dioxide | 340 | ||
11.1 Introduction | 340 | ||
11.2 Solubility of Polymers in scCO2 | 341 | ||
11.2.1 Predicting the CO2 Solubility in Polymers: Theoretical and Experimental Approaches | 342 | ||
11.2.1.1 Henry's Law | 343 | ||
11.2.1.2 Flory–Huggins Cell Model | 343 | ||
11.2.1.3 Sanchez–Lacombe Model | 344 | ||
11.2.1.4 Statistical Association Fluid Theory (SAFT) | 344 | ||
11.2.2 Experimental Techniques to Measure Solubility | 344 | ||
11.2.2.1 Gravimetric Method | 344 | ||
11.2.2.2 Manometric Method | 345 | ||
11.2.2.3 Piezoelectric Method | 345 | ||
11.2.2.4 Gas Chromatography (GC) | 345 | ||
11.2.2.5 Spectroscopic Method | 346 | ||
11.3 Plasticising Effect of CO2 | 346 | ||
11.4 Overview of the Synthesis of Polymers in scCO2 | 348 | ||
11.4.1 Step-growth Polymerisation | 348 | ||
11.4.2 Chain-growth Polymerisation in scCO2 | 349 | ||
11.4.2.1 Homogeneous Radical Polymerisation in scCO2 | 350 | ||
11.4.2.2 Heterogeneous Polymerisation in scCO2 | 352 | ||
11.4.2.2.1\rPrecipitation Polymerisation.In precipitation polymerisation, the monomers and other components start off completely soluble in ... | 352 | ||
11.4.2.2.2\rDispersion Polymerisation.This is similar to precipitation polymerisation, whereby the monomer and the initiator are completely ... | 354 | ||
11.4.2.2.3\rSuspension Polymerisation.Contrary to precipitation polymerisation and dispersion polymerisation, both the monomers and resultin... | 356 | ||
11.4.2.2.4\rEmulsion Polymerisation.Emulsion polymerisations typically involve a biphasic mixture (that is stirred) of water–organic solvent... | 357 | ||
CO2-in-H2O Emulsion Polymerisation: Examples. As stated previously, CO2-in-H2O emulsion polymerisation leads to the formation of... | 359 | ||
H2O-in-CO2 Emulsion Polymerisation: Examples. Inverse emulsion polymerisation was first reported by Beckman1397969777s group in ... | 360 | ||
11.4.2.2.5\rCLRP Polymerisation in Heterogeneous Systems Involving scCO2.scCO2 is ideal for conducting heterogeneous CLRP since the monomer,... | 360 | ||
RAFT. There is significant work reported in the literature covering RAFT-mediated dispersion polymerisation in scCO2, which was ... | 360 | ||
NMP. Initially, NMP polymerisation in scCO2 was solely reported using styrene as the monomer in dispersion (use of a stabiliser)... | 363 | ||
ATRP. Both precipitation and dispersion ATRP polymerisations have been carried out in scCO2, but in the case of the former, ther... | 364 | ||
CLRP Mini-emulsion Polymerisation in scCO2. Cheng et al. performed the RAFT mini-emulsion polymerisation of styrene with trithio... | 364 | ||
Other CLRP in scCO2. A dispersion reversible chain transfer catalysed polymerisation (dispersion RTCP) of MMA in scCO2 was condu... | 365 | ||
11.5 Conclusion | 365 | ||
References | 366 | ||
Chapter 12 - Incorporation of Drugs and Metals into Aerogels Using Supercritical Fluids | 374 | ||
12.1 Aerogels | 374 | ||
12.2 Supercritical Impregnation | 376 | ||
12.2.1 Silica Aerogels | 378 | ||
12.2.2 Polysaccharide and Protein Aerogels | 383 | ||
12.3 Supercritical Fluid Deposition | 386 | ||
12.4 Conclusion | 389 | ||
References | 390 | ||
Chapter 13 - Mathematical Modelling and Computation for Rapid Expansion of Supercritical Solutions | 395 | ||
13.1 Introduction | 395 | ||
13.2 Ideal-gas Flow Model | 396 | ||
13.2.1 Compressible Navier–Stokes Equations | 396 | ||
13.2.2 Preconditioned Equations | 398 | ||
13.2.3 Numerical Methods | 398 | ||
13.3 Thermophysical Flow Model | 399 | ||
13.3.1 Modification of Preconditioned Equations | 399 | ||
13.3.2 Thermophysical Model | 399 | ||
13.4 Condensation Model | 401 | ||
13.4.1 General Dynamic Equation | 401 | ||
13.4.2 Method of Moments | 401 | ||
13.4.3 Nucleation Model | 403 | ||
13.4.4 Growth Rate Model | 406 | ||
13.4.5 Coagulation Model | 407 | ||
13.5 RESS Simulation | 409 | ||
13.6 Concluding Remarks | 414 | ||
References | 415 | ||
Chapter 14 - The Engineering of Continuous Hydrothermal/Solvothermal Synthesis of Nanomaterials | 416 | ||
14.1 Introduction | 416 | ||
14.2 Hydrothermal Synthesis | 417 | ||
14.2.1 Nucleation and Growth Mechanisms during Hydrothermal Synthesis | 418 | ||
14.3 The Case for Continuous Flow Methods for the Synthesis of Nanomaterials | 419 | ||
14.3.1 The Road from Batch to Continuous | 420 | ||
14.4 Reactor Modelling | 423 | ||
14.4.1 Pseudo-fluid Modelling | 423 | ||
14.4.2 Computational Fluid Dynamics (CFD) Modelling | 426 | ||
14.4.3 Neutron and X-ray Measurements | 428 | ||
14.4.4 Reactor Design Principles | 430 | ||
14.4.4.1 Essential Features | 430 | ||
14.4.4.2 Important Features | 432 | ||
14.4.4.3 Desirable Features | 434 | ||
14.5 Beyond T-piece Reactors | 436 | ||
14.5.1 Y-piece Reactors | 436 | ||
14.5.2 Tangential Swirl Reactors | 436 | ||
14.5.3 Pipe-in-pipe Reactors | 438 | ||
14.5.4 Mechanical Hybrid Designs | 439 | ||
14.6 Scale Up | 439 | ||
14.7 Economics and Life Cycle Assessment (LCA) | 442 | ||
14.7.1 Production Benefits and Opportunities for CHS | 443 | ||
14.8 Conclusions and Future Outlook | 444 | ||
References | 445 | ||
Chapter 15 - The Chemistry of Continuous Hydrothermal/Solvothermal Synthesis of Nanomaterials | 449 | ||
15.1 Introduction | 449 | ||
15.2 Metal Oxides | 450 | ||
15.3 Metal Sulphides and Chalcogenides | 455 | ||
15.4 Phosphates | 460 | ||
15.5 Metals | 461 | ||
15.6 Zeolites and Metal Organic Frameworks | 464 | ||
15.7 Layered Double Hydroxides | 467 | ||
15.8 Other Materials | 468 | ||
15.9 Conclusions | 468 | ||
References | 469 | ||
Chapter 16 - Supercritical Water Oxidation: The Next Generation for Abatement of Unrecyclable Waste of Hazardous Nature and Complex Composition | 476 | ||
16.1 Background | 476 | ||
16.2 The Chemistry of Supercritical Water | 479 | ||
16.3 Features and Design Considerations for the Supercritical Water Oxidation Process | 480 | ||
16.3.1 Feedstock | 481 | ||
16.3.2 Reactor Design and Configuration | 482 | ||
16.3.3 Energy Recovery and Integration | 483 | ||
16.4 Operational Problems Associated with Supercritical Water Oxidation | 486 | ||
16.4.1 Salt Precipitation | 486 | ||
16.4.1.1 Pressure Manipulation | 487 | ||
16.4.1.2 Reactor Design Solutions | 488 | ||
16.4.2 Corrosion | 489 | ||
16.5 Enhancement of the SCWO Process | 492 | ||
16.5.1 Multi-port Oxidant Injection | 492 | ||
16.5.2 Co-fuel Assisted SCWO System | 494 | ||
16.5.3 Effects of Process Enhancements on SCWO Kinetics | 499 | ||
16.5.3.1 Case Study 1: Supercritical Water Oxidation of DBU in an IPA-added System | 500 | ||
16.5.3.2 Case Study 2: Supercritical Water Oxidation of NH4+ in an IPA-added System | 501 | ||
16.6 Performance of the SCWO System for the Treatment of Complex Waste | 506 | ||
16.6.1 Case Study: Use of SCWO for Treatment of Complex Wastewater | 506 | ||
16.7 Conclusions | 508 | ||
References | 508 | ||
Chapter 17 - Gas Expanded-liquids | 512 | ||
17.1 Introduction | 512 | ||
17.2 Carbon Dioxide-expanded Liquids (CXLs) | 515 | ||
17.2.1 Properties | 515 | ||
17.2.2 Instrumentation | 518 | ||
17.3 Applications | 519 | ||
17.3.1 Extraction and Biorefinery | 520 | ||
17.3.2 Particle Formation | 521 | ||
17.3.2.1 CO2 Acting as the Solute: Particles from a Gas-saturated Solution (PGSS) | 522 | ||
17.3.2.2 CO2 Acting as an Antisolvent | 523 | ||
17.3.2.2.1\rGas Antisolvent (GAS) Technique.The simplest process that uses CO2 as an antisolvent is the GAS process.9 It is particularly use... | 523 | ||
17.3.2.2.2\rPrecipitation with Compressed Antisolvent (PCA) and Aerosol Solvent Extraction System (ASES).The PCA process is the reverse of t... | 524 | ||
17.3.2.3 CO2 Acting as a Co-solvent: Depressurisation of an Expanded Liquid Organic Solution (DELOS) | 526 | ||
17.3.2.4 Enhanced Oil Recovery | 527 | ||
17.3.2.5 Other Applications | 528 | ||
17.4 Conclusions and Future Trends | 528 | ||
Acknowledgements | 529 | ||
References | 529 | ||
Chapter 18 - Use of Water and Supercritical Carbon Dioxide in Novel Methodologies for Biomass Processing | 532 | ||
18.1 Introduction | 532 | ||
18.2 Biomass Hydrolysis | 536 | ||
18.2.1 Effect of Pre-treatment on Hemicellulose Hydrolysis | 536 | ||
18.2.2 Influence of Pre-treatment on Cellulose Hydrolysis | 538 | ||
18.2.3 Effect of Pre-treatment on Starchy Materials | 538 | ||
18.3 Conversion of Biomass-related Monosaccharides into Value-added Products | 539 | ||
18.3.1 Hexose-derived Products | 540 | ||
18.3.2 Pentose-derived Products | 543 | ||
18.3.3 Lignin Extraction with scCO2 | 547 | ||
18.4 Effect of Pre-treatment on Enzymatic Hydrolysis | 548 | ||
18.5 Conclusions | 554 | ||
Acknowledgements | 554 | ||
References | 555 | ||
Chapter 19 - Hydrothermal Events Occurring During Gasification in Supercritical Water | 560 | ||
19.1 Introduction | 560 | ||
19.2 Thermo-physical Properties of Supercritical Water | 563 | ||
19.3 Supercritical Water Gasification | 566 | ||
19.4 Degradation of Model Compounds in Hydrothermal Gasification | 570 | ||
19.4.1 Carbohydrates | 570 | ||
19.4.2 Lignin | 572 | ||
19.4.3 Proteins | 573 | ||
19.4.4 Lipids and Fatty Acids | 575 | ||
19.4.5 Alcohols | 576 | ||
19.4.6 Sewage Sludge | 578 | ||
19.5 Conclusions | 581 | ||
Acknowledgements | 581 | ||
References | 581 | ||
Chapter 20 - Sub- and Supercritical Water Hydrolysis Applied to Agri-food Residues | 588 | ||
20.1 Introduction | 588 | ||
20.2 Sub/supercritical Water Hydrolysis Process | 590 | ||
20.3 Novel Developments in Sub/supercritical Water Hydrolysis Applied to Agri-food Residues | 597 | ||
20.4 Future Perspectives | 614 | ||
20.5 Conclusions | 615 | ||
References | 616 | ||
Chapter 21 - Applications of Fluids at Supercritical Pressure in the Area of Power Generation – Movements Towards Economically-viable Clean Energy | 620 | ||
21.1 The Properties of Fluids at Supercritical Pressure | 620 | ||
21.2 Supercritical Pressure Fluids in Power Generation Plants | 622 | ||
21.2.1 How it all Began – Supercritical Pressure ‘Steam’ Generators | 622 | ||
21.2.2 Power Stations Operating at Supercritical Pressure – The Benson Boiler | 622 | ||
21.3 Early Research on Heat Transfer with Fluids at Supercritical Pressure | 623 | ||
21.4 A Unique Study of Heat Transfer to Carbon Dioxide at Supercritical Pressure | 624 | ||
21.4.1 Diffusion of Heat with Strong Non-uniformity of Fluid Properties but in the Absence of any Advection of Heat | 624 | ||
21.4.2 The Initial Experiments | 626 | ||
21.4.3 Further Experiments | 626 | ||
21.4.4 Experiments with the Cooled Surface Uppermost | 629 | ||
21.4.5 Direct Numerical Simulation of the Experiments | 629 | ||
21.5 Localised Deterioration of Heat Transfer in Vertical Heated Tubes | 629 | ||
21.5.1 An Early Experiment Using Supercritical Water | 629 | ||
21.5.2 Experiments with Supercritical Pressure Carbon Dioxide | 631 | ||
21.5.3 Examples of Localised Deterioration of Heat Transfer with Heated Tubes of Smaller Diameter | 633 | ||
21.5.4 Experiments at Pressures Just Below the Critical Value | 633 | ||
21.6 A Simple Model of Buoyancy-induced Partial Laminarisation | 634 | ||
21.6.1 Introduction | 634 | ||
21.6.2 Effect of Buoyancy in Modifying the Distribution of Shear Stress | 635 | ||
21.6.3 Effect of Shear Stress Modification on the Turbulent Diffusion Heat | 636 | ||
21.6.4 Explanation of the Localised Nature of the Heat Transfer Deterioration | 637 | ||
21.6.5 A Simple Criterion for Partial Laminarisation | 637 | ||
21.7 Turbulent Forced Convection Heat Transfer with Fluids at Supercritical Pressure | 638 | ||
21.7.1 Introduction | 638 | ||
21.7.2 Empirical Equations for Turbulent Forced Convection Heat Transfer to Fluids at Supercritical Pressure | 639 | ||
21.7.3 An Evaluation of Some Early Equations | 639 | ||
21.7.4 Similarity and Scaling in the Case of Forced Convection Heat Transfer to Fluids at Supercritical Pressure | 641 | ||
21.7.5 Correlating Buoyancy-influenced and Acceleration-influenced Heat Transfer Data | 641 | ||
21.8 Development of Physically Based Semi-empirical Models of Heat Transfer to Supercritical Pressure Fluids | 644 | ||
21.8.1 A Revival of Interest after the Millennium in Heat Transfer to Fluids at Supercritical Pressure | 644 | ||
21.8.2 A Physically Based, Semi-empirical Model of Buoyancy-influenced Turbulent Flow and Heat Transfer in Supercritical Pressure... | 644 | ||
21.8.3 A Physically Based Semi-empirical Model of Impairment of Heat Transfer due to Thermally Induced Bulk Flow Acceleration | 646 | ||
21.8.4 Recent Work on the Development of Extended Semi-empirical Models | 648 | ||
21.9 Supercritical Transformational Electric Power Cycles | 648 | ||
21.9.1 Background | 648 | ||
21.9.2 Advantages of Power Conversion Cycles using Supercritical Pressure Carbon Dioxide | 649 | ||
21.9.3 The Supercritical CO2 Re-compression Cycle | 649 | ||
21.9.4 Other Applications of scCO2 Power Cycles | 650 | ||
21.9.5 Ongoing Studies of Applications Involving scCO2 Cycles | 650 | ||
21.9.6 Ongoing Work | 651 | ||
21.9.7 Where are we Heading Clean Energy Production Using scCO2 Power Cycles | 652 | ||
Nomenclature | 652 | ||
Greek symbols | 653 | ||
Subscripts | 653 | ||
Superscripts | 654 | ||
Appendix: Steady Turbulent Flow and Heat Transfer in Tubes | 654 | ||
1 Introduction | 654 | ||
2 Historical Background – Analogy Between Friction and Heat Transfer | 654 | ||
3 Reynolds’s Ground Breaking Experimental Study of Turbulent Flow in Tubes | 657 | ||
4 The Basis of our Present Day Approach to Turbulent Flow –Reynolds’s Dynamical Theory of Incompressible Viscous Fluids | 660 | ||
5 Turbulent Flow and Heat Transfer in Tubes – the Present Picture | 662 | ||
References | 665 | ||
Subject Index | 667 |