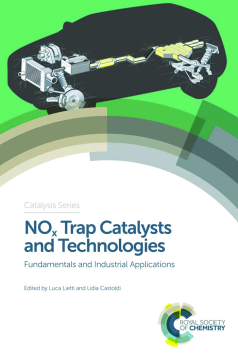
Additional Information
Book Details
Abstract
Vehicle exhaust emissions, particularly from diesel cars, are considered to be a significant problem for the environment and human health. Lean NOx Trap (LNT) or NOx Storage/Reduction (NSR) technology is one of the current techniques used in the abatement of NOx from lean exhausts. Researchers are constantly searching for new inexpensive catalysts with high efficiency at low temperatures and negligible fuel penalties, to meet the challenges of this field.
This book will be the first to comprehensively present the current research on this important area. Covering the technology used, from its development in the early 1990s up to the current state-of-the-art technologies and new legislation. Beginning with the fundamental aspects of the process, the discussion will cover the real application standard through to the detailed modelling of full scale catalysts.
Scientists, academic and industrial researchers, engineers working in the automotive sector and technicians working on emission control will find this book an invaluable resource.
Table of Contents
Section Title | Page | Action | Price |
---|---|---|---|
Cover | Cover | ||
Preface | vii | ||
Contents | ix | ||
Chapter 1 Review of deNOx Technology for Mobile Applications | 1 | ||
1.1 Introduction | 1 | ||
1.2 Regulatory Overview | 2 | ||
1.2.1 Light-duty Regulations | 2 | ||
1.2.2 Heavy-duty Truck Regulations | 3 | ||
1.3 Emission Control Technology for Diesel and Gasoline Engines | 4 | ||
1.3.1 Selective Catalytic Reduction (SCR) | 5 | ||
1.3.2 NOx Trap Catalysts | 10 | ||
1.3.3 NTC + SCR Combination Systems | 13 | ||
1.3.4 Diesel Particulate Filters | 16 | ||
1.3.5 Gasoline Emission Control | 17 | ||
1.4 Future Perspectives | 29 | ||
1.5 Conclusions | 29 | ||
References | 31 | ||
Chapter 2 NSR Technology | 36 | ||
2.1 Introduction | 36 | ||
2.2 General Overview on the NSR Technology | 38 | ||
2.2.1 NSR Concept | 38 | ||
2.2.2 Chemistry of NSR | 40 | ||
2.2.3 Efficiency and Selectivity of NSR Catalyst | 43 | ||
2.3 NOx Abatement in Light and Heavy Duty Engines | 47 | ||
2.4 Integration of Systems with NOx Technology for Light Duty Vehicles | 50 | ||
2.4.1 Combined Particulate and NOx Aftertreatment Systems | 50 | ||
2.4.2 Coupling of NOx Storage and Reduction and Selective Catalytic Reduction Technologies | 53 | ||
2.5 Futures Perspectives | 58 | ||
2.6 Concluding Remarks | 60 | ||
Acknowledgements | 61 | ||
References | 61 | ||
Chapter 3 NSR Catalytic Materials | 67 | ||
3.1 Introduction | 67 | ||
3.2 Requirements for NSR Catalytic Materials | 69 | ||
3.3 PGM-based Catalytic Materials | 70 | ||
3.3.1 Effect of Preparations or Activation Procedures of Pt–Ba–Al2O3 Catalysts | 70 | ||
3.3.2 Effect of Support on the Performance of Pt Catalysts | 72 | ||
3.3.3 Potassium-doped NSR Catalysts | 74 | ||
3.3.4 Cerium-doped NSR Catalysts | 77 | ||
3.3.5 Platinum Catalysts with Other NOx Storage Materials | 80 | ||
3.3.6 NSR Materials with Other Noble Metals | 83 | ||
3.4 Noble Metal-free Catalytic Materials | 85 | ||
3.4.1 Perovskite-based NSR Catalysts | 86 | ||
3.4.2 Non-perovskitic NSR Catalysts Based on Transition Metal Oxides | 88 | ||
3.5 Durability and Deactivation of Catalytic Materials | 89 | ||
3.5.1 Durability. Effect of Thermal Deactivation | 89 | ||
3.5.2 Sulfur Poisoning | 91 | ||
3.5.3 Improvement of Sulfur Resistance | 91 | ||
3.6 Conclusions and Perspectives | 95 | ||
References | 96 | ||
Chapter 4 Lean NOx Trap Performance Degradation – Reversible Sulfur Poisoning and Irreversible Thermally-induced Sintering | 104 | ||
4.1 Thermal Degradation | 105 | ||
4.2 Impact on Precious Metal | 105 | ||
4.3 Impact on the Trapping Component | 108 | ||
4.4 Sulfur Poisoning | 111 | ||
4.5 Desulfation | 116 | ||
4.6 Catalyst Formulation Studies and Sulfur Poisoning Resistance | 119 | ||
References | 122 | ||
Chapter 5 Mechanism and Kinetics of NOx Storage | 127 | ||
5.1 Introduction | 127 | ||
5.2 Mechanisms of NOx Storage | 128 | ||
5.2.1 NO Oxidation | 128 | ||
5.2.2 NOx Adsorption | 131 | ||
5.3 Microkinetic Models for the Storage of Nox | 133 | ||
5.3.1 Detailed Kinetic Model for NO Oxidation | 135 | ||
5.3.2 Detailed Kinetic Model for NOx Storage and Desorption | 138 | ||
5.4 Effect of CO2, H2O and SO2 on the Storage of Nox | 142 | ||
5.4.1 Effect of H2O and CO2 on the Storage of Nox | 142 | ||
5.4.2 Effect of SO2 on the Storage of Nox | 145 | ||
5.5 Conclusions | 153 | ||
Acknowledgements | 154 | ||
References | 154 | ||
Chapter 6 Active Sites for NOx Uptake, and the Nature of Stored NOx Species | 157 | ||
6.1 Introduction | 157 | ||
6.2 Storage Phase Morphology, Active SitesInvolved in NOx Storage, and the Nature of Stored Nox | 159 | ||
6.2.1 Formation of the NSR Catalyst During Synthesis | 159 | ||
6.2.2 Morphology of the BaO Storage Material During NOx Uptake and Release | 165 | ||
6.2.3 Nature of Adsorbed and Absorbed Nox | 165 | ||
6.3 Effect of H2O, CO2 and SO2 on the Active Sites and the NOx Uptake/Release Processes in NSR Catalysts | 169 | ||
6.3.1 Effects of H2O | 170 | ||
6.3.2 Effects of Other Acid Gases, CO2 and SO2 | 174 | ||
References | 185 | ||
Chapter 7 Mechanistic Aspects of the Reduction of the Stored NOx by H2 Investigated by Isotopic Labelling Experiments and FTIR Spectroscopy | 187 | ||
7.1 Introduction | 187 | ||
7.2 Nature of the Adsorbed NOx Species | 188 | ||
7.3 Thermal Stability of the Stored NOx Species | 191 | ||
7.4 Release of Nox | 193 | ||
7.5 Reduction Pathways for N2 Formation | 195 | ||
7.5.1 Reactivity of Gaseous NO | 195 | ||
7.5.2 Reactivity of Stored Nox | 200 | ||
7.5.3 Reaction Pathway for the Reduction of the Stored Nox | 203 | ||
7.6 N2O Formation During Operation of LNTs | 204 | ||
7.7 Effect of CO2/H2O Presence | 206 | ||
7.8 Conclusions | 209 | ||
References | 210 | ||
Chapter 8 NOx Storage and Reduction: Effects of Pt Dispersion, Reductant Type, and Cycle Timing | 213 | ||
8.1 Introduction | 213 | ||
8.2 Precious Group Metal Dispersion, Stored NOx Diffusion, and Byproduct Formation | 215 | ||
8.3 Impact of Cycle Time and Non-isothermal Effects | 222 | ||
8.4 NSR with CO and Hydrocarbons: Chemical Pathways and Spatio-temporal Effects | 229 | ||
8.5 HC–NOx Reduction Pathways. Comparison of Reductants: H2 and C3H6 | 233 | ||
8.6 Summary | 241 | ||
Nomenclature | 242 | ||
Acknowledgements | 243 | ||
References | 243 | ||
Chapter 9 Reduction of Stored NOx with CO/H2 and Hydrocarbons: A Spatial Resolution Analysis | 245 | ||
9.1 Introduction | 245 | ||
9.2 Pathways Involved in the Reduction of Stored NOx Investigated by Spatially Resolved Analysis | 248 | ||
9.2.1 Reactivity of Different Reductantsand Impact on Spatial NOx Distribution | 248 | ||
9.2.2 Evolution of Reductants Along the Length of the Catalyst | 256 | ||
9.2.3 Product Selectivity During Regeneration: Effects of Local Composition | 258 | ||
9.2.4 Role of Spatial Distributions of Reactions in Determining Product Selectivity | 260 | ||
9.2.5 Impact of Spatial Distribution of NOx Storage on Product Selectivity | 266 | ||
9.2.6 Impact of Sulfur Poisoning on Regeneration Product Selectivity Explained Through Changes in Spatial Reaction Distributions | 267 | ||
9.3 Regeneration Strategies to Minimize Byproduct Formation | 270 | ||
9.3.1 Controlling Temperature, Reductant Dosage, and Cycling Timing | 271 | ||
9.3.2 Insertion of Stoichiometric Purge Between Rich and Lean Phases | 273 | ||
9.4 Conclusions | 275 | ||
Acknowledgements | 275 | ||
References | 276 | ||
Chapter 10 Global Kinetic Modelling of the NSR Catalysts | 279 | ||
10.1 Global Kinetic Model Purpose and Requirements | 279 | ||
10.2 Modelling of Mass and Heat Transport Effects | 280 | ||
10.2.1 Monolith Channel Scale | 281 | ||
10.2.2 Washcoat Scale | 283 | ||
10.2.3 Storage Particles Scale | 284 | ||
10.3 Global Reaction Kinetics | 286 | ||
10.3.1 Kinetic Parameters Evaluation | 287 | ||
10.3.2 Oxidation and Three-way Reactions | 292 | ||
10.3.3 NOx Storage | 297 | ||
10.3.4 NOx Reduction During Rich Phase | 300 | ||
10.4 Driving Cycle Simulations | 307 | ||
10.4.1 Model Validation | 307 | ||
10.4.2 Simulation Studies | 309 | ||
Nomenclature | 317 | ||
References | 318 | ||
Chapter 11 Combined LNT–SCR Catalysts for NOx Reduction from Lean Exhaust Gas | 321 | ||
11.1 Introduction | 321 | ||
11.2 Ammonia Formation Over LNT Catalysts | 322 | ||
11.3 Ammonia Storage and NOx Reduction Over SCR Catalysts | 328 | ||
11.4 Coupling LNT and SCR Catalysts | 331 | ||
11.4.1 Fundamental Studies of Combined LNT + SCR System Under Lean–Rich Cycling Conditions | 331 | ||
11.4.2 Performances of Combined LNT–SCR Systems Under Realistic Conditions | 339 | ||
11.5 Conclusion | 347 | ||
References | 348 | ||
Chapter 12 LNT Catalysts for the Simultaneous Removal of NOx and Soot: The DPNR Concept | 353 | ||
12.1 Introduction | 353 | ||
12.2 Soot Removal over LNT Catalysts | 357 | ||
12.3 Simultaneous Removal of NOx and Soot Oxidation over LNT Catalysts | 367 | ||
12.4 Novel Pt-free LNT Formulations | 374 | ||
12.5 Other Systems for the Simultaneous Removal of NOx and Soot | 377 | ||
12.6 Conclusions | 378 | ||
References | 379 | ||
Chapter 13 Non-thermal Plasma NOx Storage-reduction | 384 | ||
13.1 Introduction | 384 | ||
13.2 Reactivity of NSR Catalysts under Non-thermal Plasma Conditions | 385 | ||
13.2.1 Effect of Non-thermal Plasma on Catalyst Properties | 385 | ||
13.2.2 System Architecture for Combining NTP with NSR Catalysts | 386 | ||
13.2.3 NOx Storage under NTP Conditions | 389 | ||
13.2.4 NOx Reduction on NSR Catalysts under NTP Conditions | 391 | ||
13.3 Pathways Involved in the Removal of NOx in the Presence of Non-thermal Plasma | 398 | ||
13.3.1 Effect of Non-thermal Plasma on NOx Adsorption | 398 | ||
13.3.2 Effect of Non-thermal Plasma on NOx Reduction | 400 | ||
13.4 Conclusions and Outlook | 403 | ||
Acknowledgements | 404 | ||
References | 404 | ||
Chapter 14 New deNOx Concept by Fast Reductants Injection Pulse Upstream NSR | 407 | ||
14.1 Introduction | 407 | ||
14.2 New deNOx Concept by Fast Reductants Injection Pulse Upstream NSR | 408 | ||
14.2.1 Background and Basic Concept | 408 | ||
14.2.2 System Layout and deNOx Performance | 408 | ||
14.3 Pathways and Mechanisms Involved in NOx Reduction with the New Concept | 410 | ||
14.3.1 deNOx Performance with Injection Pulse of Different Reductants | 410 | ||
14.3.2 Analysis of Gaseous Species with Fast Time Resolution at the Moment of HC Injection Pulse | 413 | ||
14.3.3 Observation of Metallization Period of PGM by DXAFS | 415 | ||
14.3.4 Investigation of Adsorbed Species by FTIR | 417 | ||
14.3.5 Observation of N2 Behavior during Rich to Lean Condition by Labelled 15NO | 421 | ||
14.3.6 Proposed Reaction Mechanism | 423 | ||
14.4 Application of the New Concept System into Vehicles | 423 | ||
14.4.1 Investigation of the Operation Range | 423 | ||
14.4.2 Performance Study in Transient Cycles | 425 | ||
14.5 Concluding Remarks and Perspectives | 428 | ||
References | 428 | ||
Chapter 15 Development of Combined NSR DeNOx and DeSOx Model and Application for Control Strategy Pre-calibration of a Modern Diesel Engine | 430 | ||
15.1 Introduction | 430 | ||
15.2 DeNOx and DeSOx Modelling | 432 | ||
15.2.1 Mathematical Model Formulation | 432 | ||
15.2.2 Testing for Reaction Model Calibration | 435 | ||
15.2.3 NOx Storage and Reduction | 436 | ||
15.2.4 Sulfur Storage (Sulfation) | 441 | ||
15.2.5 Sulfur Removal (Desulfation) | 445 | ||
15.3 DeNOx and DeSOx of Real Conditions in Driving Cycles | 453 | ||
15.3.1 Engine Bench Experiment | 453 | ||
15.3.2 Driving Conditions Simulation | 455 | ||
15.4 Application | 458 | ||
15.4.1 Implementation of the Exhaust Aftertreatment Model in the Virtual Diesel Engine Platform | 458 | ||
15.4.2 Estimation of Engine Out Emission, Including Rich Pulse | 459 | ||
15.4.3 Validation of the Proposed Method During a Rich Engine Operating Event | 459 | ||
15.4.4 Estimation of Rich Pulse Timing Impacton DeNOx Performance and CO2 Emission Under Real Driving Emission (RDE) Conditions | 461 | ||
15.5 Conclusions | 463 | ||
Nomenclature | 463 | ||
Acknowledgements | 464 | ||
References | 465 | ||
Chapter 16 LNT Catalysis at Ford Motor Company – A Case History | 467 | ||
16.1 Introduction | 467 | ||
16.2 General Considerations for LNT Use on Vehicles | 468 | ||
16.3 LNT for Lean Burn Gasoline Vehicles | 469 | ||
16.3.1 Gasoline LNT Composition | 469 | ||
16.3.2 Effect of PGM Loading | 472 | ||
16.3.3 Effect of Temperature on NOx Conversion | 473 | ||
16.3.4 Gasoline LNT Thermal Stability | 474 | ||
16.3.5 Sulfur Effects on LNT and Desulfation | 475 | ||
16.3.6 LNT Ammonia Generation and Addition of SCR | 478 | ||
16.3.7 Ford Lean Burn Gasoline Vehicle Programs | 479 | ||
16.4 LNT for Diesel Vehicles | 481 | ||
16.4.1 Diesel LNT Composition, Thermal Aging, and Desulfation | 481 | ||
16.4.2 Ford Diesel LNT Programs | 483 | ||
16.4.3 Diesel Outlook | 485 | ||
16.5 Summary | 487 | ||
Acknowledgements | 487 | ||
References | 487 | ||
Subject Index | 490 |