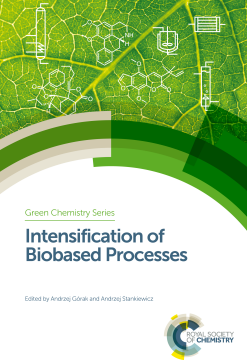
Additional Information
Book Details
Abstract
In recent years bioprocessing has increased in popularity and importance, however, bioprocessing still poses various important techno-economic and environmental challenges, such as product yields, excessive energy consumption for separations in highly watery systems, batch operation or the downstream processing bottlenecks in the production of biopharmaceutical products. Many of those challenges can be addressed by application of different process intensification technologies discussed in the present book.
The first book dedicated entirely to this area, Intensification of Biobased Processes provides a comprehensive overview of modern process intensification technologies used in bioprocessing. The book focusses on four different categories of biobased products: bio-fuels and platform chemicals; cosmeceuticals; food products; and polymers and advanced materials. It will cover various intensification aspects of the processes concerned, including (bio)reactor intensification; intensification of separation, recovery and formulation operations; and process integration. This is an invaluable source of information for researchers and industrialists working in chemical engineering, biotechnology and process engineering.
Prof. Andrzej Górak is a Professor at Lodz University of Technology, Poland and an Emeritus Professor of TU Dortmund University. He is an Editor of Chemical Engineering and Processing: Process Intensification and a Board Member of the European Process Intensification Centre (EUROPIC).
His scientific interests are reactive and bioreactive separation processes, process intensification, computer aided process engineering. His research is also focused on experimental investigations of reactive and hybrid separation processes such as reactive distillation and membrane separations.
Andrzej Stankiewicz is Full Professor at Delft University of Technology (Chair of Intensified Reaction and Separation Systems) and Director of TU Delft Process Technology Institute. He is author of numerous publications on chemical reaction engineering, industrial catalysis and process intensification, including the world’s first book on Process Intensification: Re-Engineering the Chemical Processing Plant.
Prof. Stankiewicz is an Editor of Chemical Engineering and Processing: Process Intensification and a Series Editor of the Green Chemistry Books Series. He was the founder and first Chairman of the Working Party on Process Intensification at the European Federation of Chemical Engineering, and currently chairs the Board of the European Process Intensification Centre (EUROPIC).
His current research interests are control of molecular interactions and intensification of chemical processes using electric and electromagnetic fields. And he has been awarded the prestigious Advanced Investigator Grant by the European Research Council (with co-workers) and a grant by the Bill and Melinda Gates Foundation.
Table of Contents
Section Title | Page | Action | Price |
---|---|---|---|
Cover | Cover | ||
Intensification of Biobased Processes | i | ||
Preface | vii | ||
Dedication | xi | ||
Contents | xiii | ||
Chapter 1 - Intensified Fermentation Processes and Equipment | 1 | ||
1.1 Introduction | 1 | ||
1.2 Fermentation | 3 | ||
1.2.1 Fermentation Processes | 3 | ||
1.2.2 Fermentation Equipment | 4 | ||
1.3 Fermentation Fundamentals and Rate-limiting Factors | 6 | ||
1.3.1 Stoichiometry/Kinetics | 6 | ||
1.3.2 Operation Modes: Use of the Invested Utilities to the Max | 7 | ||
1.3.3 Transport Limitations | 9 | ||
1.3.4 Scale Dependency of Flow Regimes and Transport | 9 | ||
1.3.5 Biomass Retention | 9 | ||
1.4 Hydrodynamics | 10 | ||
1.4.1 Hydrodynamics in Non-stirred Fermenters | 10 | ||
1.4.1.1 Power Input | 10 | ||
1.4.1.2 Fluid Flow Regimes | 11 | ||
1.4.1.3 Liquid Flow and Velocity | 12 | ||
1.4.2 Hydrodynamics in Stirred Fermenters | 13 | ||
1.4.2.1 Power Input | 13 | ||
1.4.2.2 Flow Regimes | 14 | ||
1.4.3 Mass Transfer | 15 | ||
1.4.4 Heat Transfer | 18 | ||
1.4.5 Liquid Mixing | 20 | ||
1.5 Fermentation Intensification | 22 | ||
1.6 Examples | 24 | ||
1.6.1 Bioethanol Production with Saccharomyces cerevisiae: Smart Integration (FI Principle 4) | 24 | ||
1.6.2 Biopharmaceuticals Production with Mammalian Cells: Jump via Cell Retention (FI Principles 2 and 4) | 25 | ||
1.6.3 Bakers’ Yeast Production: Debottlenecking Oxygen Transfer (FI Principle 3) | 26 | ||
1.6.4 Synergistic Production of Complex and Non-natural Compounds via Fermentation (FI Principles 1 and 4) | 28 | ||
1.7 Fermentation Intensification Limits | 28 | ||
1.7.1 Bakers’ Yeast Case | 31 | ||
1.7.2 BDO Case | 34 | ||
1.8 Conclusions and Outlook | 35 | ||
Abbreviations | 38 | ||
Acknowledgements | 38 | ||
References | 38 | ||
Chapter 2 - Rotating Bioreactors: Concept, Designs and Applications | 42 | ||
2.1 Introduction | 42 | ||
2.2 Background | 43 | ||
2.2.1 Bioreactor Design Requirements for Microbial Transformations | 43 | ||
2.2.2 Rotating Reactor: Design Concept, Characteristics and Processing Benefits | 45 | ||
2.3 Rotating Reactors for Microbial Fermentation and Biotransformation Applications | 46 | ||
2.3.1 Rotating Fluidised Bed | 46 | ||
2.3.2 Rotating Bed Bioreactors | 49 | ||
2.3.3 Rotating Biofilm Contactors | 54 | ||
2.3.4 Rotating Membrane Bioreactors | 57 | ||
2.4 Summary and Outlook | 58 | ||
References | 59 | ||
Chapter 3 - Intensified Downstream Processing in Biofuels Production | 62 | ||
3.1 Introduction | 62 | ||
3.2 Types of Biofuels | 63 | ||
3.3 Biodiesel | 65 | ||
3.3.1 Dividing-wall Column (DWC) Technology | 66 | ||
3.3.2 Reactive Distillation | 67 | ||
3.3.3 Reactive Dividing-wall Column | 69 | ||
3.3.4 Other PI Techniques | 70 | ||
3.4 Bioethanol | 71 | ||
3.4.1 Extractive Dividing-wall Column | 72 | ||
3.4.2 Heat Pump Assisted Extractive Distillation | 72 | ||
3.5 Biobutanol | 76 | ||
3.6 Dimethyl Ether | 77 | ||
3.6.1 DWC Ternary Separation | 79 | ||
3.6.2 Reactive DWC | 80 | ||
3.6.3 Reactive Distillation | 81 | ||
3.6.4 Catalytic Cyclic Distillation | 82 | ||
3.7 Concluding Remarks | 83 | ||
References | 84 | ||
Chapter 4 - Improving Fermentation by Product Removal | 86 | ||
4.1 Introduction | 86 | ||
4.2 The Roles of ISPR | 88 | ||
4.2.1 Improving the Product Formation Rate and Yield of Product on Substrate | 88 | ||
4.2.2 Avoiding Product Degradation | 91 | ||
4.2.3 Improving Downstream Processing | 91 | ||
4.3 Process Design Aspects | 92 | ||
4.3.1 Configurations | 92 | ||
4.3.2 Batch Versus Continuous Operating Mode | 93 | ||
4.3.3 The Importance of Cell Reuse in ISPR | 95 | ||
4.3.4 Recycles | 96 | ||
4.3.5 Modelling and Simulation | 96 | ||
4.3.6 Calculating Generic Conditions at Which ISPR is Appropriate | 98 | ||
4.4 ISPR for Phase Separating Compounds | 98 | ||
4.4.1 General Considerations | 98 | ||
4.4.2 Example: Development of ISPR for 2-butanol Production | 101 | ||
4.4.3 Example: Development of ISPR for Sesquiterpene Production | 102 | ||
4.5 Concluding Remarks | 104 | ||
Appendix. Comparison of Performance of Base Case and ISPR Case | 105 | ||
References | 106 | ||
Chapter 5 - Liquid–Liquid Extraction in Processing of Bioproducts | 109 | ||
5.1 Introduction to Liquid–Liquid Extraction | 109 | ||
5.2 Process Intensification in Liquid–Liquid Extraction | 110 | ||
5.2.1 Intensification Using New Materials in Extraction Systems | 111 | ||
5.2.1.1 Water-based and Renewable Solvents | 112 | ||
5.2.1.2 Ionic Liquids (ILs) and Deep Eutectic Solvents (DES) | 113 | ||
5.2.1.3 Surfactants, Microemulsions and Micelles | 114 | ||
5.2.2 Intensification by Novel Equipment and External Force | 114 | ||
5.2.2.1 Intensification in Equipment for Liquid–Liquid Extraction | 114 | ||
5.2.2.2 External Force Assisted Extraction | 115 | ||
5.2.3 Intensification by Integrated and Hybrid Extraction Methods | 117 | ||
5.2.3.1 Integrated Solvent Extraction Strategies | 117 | ||
5.2.3.2 Intensification by Hybrid Separation Methods | 117 | ||
5.3 Application of Liquid–Liquid Extraction to Bioproducts | 118 | ||
5.3.1 Recovery of Fermentation-derived Products | 119 | ||
5.3.1.1 Recovery of Antibiotics | 119 | ||
5.3.1.2 Recovery of Carboxylic Acids and Organic Acids | 120 | ||
5.3.1.3 Other Fermentation-derived Products | 120 | ||
5.3.1.4 Extraction of Biopolymers and Cellular Components | 121 | ||
5.3.2 Biofuels, Platform Chemicals and Biomass Hydrolysate Products | 122 | ||
5.3.2.1 Biofuels | 122 | ||
5.3.2.2 Platform Chemicals | 123 | ||
5.3.2.3 Biomass Hydrolysate Components and Impurities | 123 | ||
5.3.3 Microalgal Bio-based Products | 123 | ||
5.4 Conclusions and Outlook | 124 | ||
References | 125 | ||
Chapter 6 - Organic Solvent Nanofiltration for an Intensified Processing of Renewable Raw Materials | 132 | ||
6.1 Introduction | 132 | ||
6.1.1 Oils and Fats | 133 | ||
6.1.2 Current Processes and Challenges | 133 | ||
6.2 Organic Solvent Nanofiltration | 134 | ||
6.2.1 Fundamentals, Benefits and Constraints | 134 | ||
6.2.2 Membrane Modules, Cascades and Membrane-assisted Processes | 135 | ||
6.3 Applications | 137 | ||
6.3.1 Solvent Recovery | 138 | ||
6.3.2 Deacidification | 139 | ||
6.4 Conclusion | 141 | ||
References | 142 | ||
Chapter 7 - Green Fuels and Fuel Additives Production in Simulated Moving Bed Reactors | 145 | ||
7.1 Simulated Moving Bed Reactor Technology – What is it | 145 | ||
7.2 Design and Modelling of Simulated Moving Bed Reactor Processes | 148 | ||
7.3 Simulated Moving Bed Reactor in the Context of Bio-based Industries: Production of Fuels and Fuel Additives | 152 | ||
7.3.1 Production of Acetals from Bioethanol | 154 | ||
7.3.2 Valorization of Bioglycerol | 158 | ||
7.4 Conclusions | 161 | ||
Nomenclature | 162 | ||
Greek Letters | 162 | ||
Superscripts and Subscripts | 162 | ||
Abbreviations | 163 | ||
Acknowledgements | 163 | ||
References | 163 | ||
Chapter 8 - Intensification of Enzymatic Hydrolysis of Cellulose Using High Frequency Ultrasound | 166 | ||
8.1 Introduction | 166 | ||
8.2 Theoretical Background | 169 | ||
8.2.1 Fundamental Aspects of Ultrasound and Cavitation | 169 | ||
8.2.2 Overview of Cavitation-assisted Enzymatic Hydrolysis | 171 | ||
8.2.3 Overview of the Effects of Ultrasound on Enzymes | 173 | ||
8.3 Experimental Section | 174 | ||
8.3.1 Materials | 174 | ||
8.3.2 Procedures and Sample Preparations | 175 | ||
8.3.3 Brunauer–Emmett–Teller (BET) and X-ray Diffraction (XRD) Surface Analysis | 176 | ||
8.4 Results and Discussion | 177 | ||
8.4.1 Enzymatic Hydrolysis of Cellulose in the Presence and Absence of Ultrasound | 177 | ||
8.4.2 Effects of the Frequency of Ultrasound on Enzymatic Hydrolysis | 179 | ||
8.4.3 BET and XRD Studies | 180 | ||
8.5 Energy, Cost, and Scale-up Considerations | 182 | ||
8.6 Conclusions | 184 | ||
Acknowledgements | 184 | ||
References | 185 | ||
Chapter 9 - Process Intensification for Hydroprocessing of Vegetable Oil | 188 | ||
9.1 Introduction | 188 | ||
9.2 Experimental | 191 | ||
9.2.1 Catalyst Coatings for Microchannel Reactors | 191 | ||
9.2.2 Microchannel Plate Pretreatment and Catalyst Coating | 192 | ||
9.2.3 Experimental Setup | 192 | ||
9.2.4 Catalyst Activity Test and Jatropha Properties | 194 | ||
9.3 Results and Discussion | 195 | ||
9.3.1 Physicochemical Properties of the Catalyst | 195 | ||
9.3.2 |
195 | ||
9.3.3 C9–C14 Hydrocarbons Yield | 196 | ||
9.3.4 C15–C18 Hydrocarbons Yield | 197 | ||
9.3.5 >C18 Oligomerized Products Yield | 198 | ||
9.3.6 Influence of Pressure on Product Yield in the Microchannel Reactor | 198 | ||
9.3.7 Kinetics and Thermodynamics of Vegetable Oil Hydroprocessing | 200 | ||
9.3.8 Computational Dynamics Studies | 200 | ||
9.3.8.1 Molar Flow Rate Variation of Reactant and Products in COMSOL | 200 | ||
9.3.8.2 Concentration, Temperature and Velocity Profile for Triglyceride Hydroprocessing in the Microchannel Reactor | 202 | ||
9.3.8.3 Concentration Profile of Products | 204 | ||
9.4 Conclusion | 205 | ||
Acknowledgements | 207 | ||
References | 207 | ||
Chapter 10 - Enzymatic Reactive Absorption and Distillation | 210 | ||
10.1 Introduction | 210 | ||
10.1.1 Reactive Absorption (RA) and Distillation (RD) | 211 | ||
10.1.2 Enzymatic Reactive Absorption (ERA) and Distillation (ERD) | 212 | ||
10.2 Technical Realization | 215 | ||
10.2.1 Equipment for ERA and ERD Processes | 215 | ||
10.2.1.1 Columns | 215 | ||
10.2.1.2 Rotating Packed Beds | 216 | ||
10.2.1.3 Membrane Contactors | 218 | ||
10.2.1.4 Criteria for Equipment Selection | 219 | ||
10.2.2 Application of Enzymes | 219 | ||
10.2.2.1 Homogeneous Application and Recovery | 220 | ||
10.2.2.2 Heterogeneous Enzyme Application | 222 | ||
10.3 Modeling and Design | 225 | ||
10.3.1 Modeling of Continuous ERA and ERD | 226 | ||
10.3.2 Reaction Kinetics for Enzyme Reactions | 228 | ||
10.4 Application Examples | 229 | ||
10.4.1 Application of ERA for CO2 Capture | 230 | ||
10.4.1.1 Challenges in Conventional CO2-capture Processes | 230 | ||
10.4.1.2 Carbonic Anhydrase in CO2-capture Processes | 231 | ||
10.4.1.3 Performance of Enzyme-enhanced Absorption Processes | 232 | ||
10.4.2 Application of ERD | 234 | ||
10.4.2.1 Transesterification to Butyl Butyrate | 235 | ||
10.4.2.2 Resolution of Secondary Alcohols | 236 | ||
10.4.2.3 The Potential of ERD | 237 | ||
10.5 Conclusions | 238 | ||
Acknowledgements | 240 | ||
References | 240 | ||
Chapter 11 - Process Intensification of Immobilized Enzyme Reactors | 249 | ||
11.1 Introduction | 249 | ||
11.2 Enzymes as Catalysts | 250 | ||
11.3 Enzymatic Reactors: Conventional Versus Process Intensified Reactors | 251 | ||
11.3.1 Conventional Reactors and Enzyme Catalysis | 251 | ||
11.3.2 Process Intensified Enzymatic Reactors (PI-ER) | 252 | ||
11.3.2.1 Enzymatic Membrane Reactor (EMR) | 252 | ||
11.3.2.2 Enzymatic Microreactors | 255 | ||
11.3.2.3 Immobilized Monolithic Enzyme Reactors (IMERs) | 258 | ||
11.3.2.4 Rotating Packed Bed Reactors (RPBs) | 260 | ||
11.3.2.5 Spinning Mesh Disc Reactor (SMDR) | 261 | ||
11.4 Conclusion | 263 | ||
References | 263 | ||
Chapter 12 - Process Intensification of Enzymatic Biotransformation Processes | 268 | ||
12.1 Introduction | 268 | ||
12.1.1 Enzymatic Biotransformation | 268 | ||
12.1.2 Process Intensification | 271 | ||
12.2 Process Intensification and Biotransformation | 272 | ||
12.3 Case Study 1 – Extractive Biotransformation of Naphthalene | 277 | ||
12.4 Case Study 2 – Intensification of Enzymatic Hydrolyis of Triglyceride Ester | 279 | ||
12.5 Conclusions | 284 | ||
References | 285 | ||
Chapter 13 - Microalgae: From Bio-based Curiosity Towards a Bulk Feedstock | 289 | ||
13.1 Microalgae and Their Potential for Bulk-commodities and Biofuels | 289 | ||
13.2 Cultivation of Microalgae: Current State and Emerging Trends | 290 | ||
13.2.1 Current Phototrophic Cultivation | 290 | ||
13.2.2 Novel Reactor Designs | 293 | ||
13.2.2.1 Panel Photobioreactors | 293 | ||
13.2.2.2 Tubular Photobioreactors with Wavy Two-phase Flow | 293 | ||
13.2.2.3 Thin-layer Systems – Sloped Shallow Ponds | 293 | ||
13.2.2.4 Biofilm Systems | 294 | ||
13.3 From Single to Multiple Products | 294 | ||
13.3.1 Necessity for Low Cost- and Mild Technologies | 294 | ||
13.3.2 Harvesting Microalgae | 295 | ||
13.3.3 Cell Disruption | 296 | ||
13.3.4 Extraction | 297 | ||
13.4 Concluding Remarks | 298 | ||
13.4.1 State-of-development of Microalgae for Bulk Products | 298 | ||
13.4.2 Further Steps Towards Feasible Bulk-commodity Production | 299 | ||
References | 299 | ||
Chapter 14 - Process Intensification in Glutamic Acid Production | 303 | ||
14.1 Introduction | 303 | ||
14.2 Current Production Process and the Challenges in Sustainability | 304 | ||
14.3 Process Intensification Approaches Towards Sustainability | 306 | ||
14.4 Process Intensification in Glutamic Acid Production Through a Membrane-integrated Reactor System | 307 | ||
14.5 The Fermentation Media for the Bioprocess | 311 | ||
14.6 Fermentation | 311 | ||
14.7 Analytical Determination for Process Monitoring | 312 | ||
14.8 Membrane Fouling and Cleaning | 312 | ||
14.9 Analysis of Process Intensification Through a Membrane-integrated Hybrid Reactor System | 313 | ||
14.9.1 Production Parameters | 313 | ||
14.9.2 Plant Configuration | 315 | ||
14.9.3 Space Intensification | 317 | ||
14.9.4 Flexibility in Capacity and Application | 319 | ||
14.9.5 Energy Intensity | 319 | ||
14.9.6 Labour Intensity | 320 | ||
14.9.7 Eco-friendliness | 321 | ||
14.9.8 Economic Gain | 321 | ||
14.10 Conclusion | 325 | ||
References | 325 | ||
Chapter 15 - Intensified Production of Recombinant Proteins | 327 | ||
15.1 Introduction | 327 | ||
15.1.1 Recombinant Monoclonal Antibodies as Pharmaceuticals | 327 | ||
15.1.2 Development and Production of a Recombinant Monoclonal Antibody | 328 | ||
15.1.3 State-of-the-art Monoclonal Antibody Production | 328 | ||
15.1.3.1 Upstream: Cultivation of Cells | 328 | ||
15.1.3.2 Downstream: Monoclonal Antibody Capture and Polishing | 329 | ||
15.2 Continuous Cultivation of Mammalian Cell Cultures | 331 | ||
15.2.1 Perfusion Culture Systems | 331 | ||
15.2.2 Steady State Operation of Continuous Bioreactors | 332 | ||
15.3 Continuous Countercurrent Chromatography in Monoclonal Antibody Capture | 333 | ||
15.3.1 Principles of Countercurrent Chromatography | 333 | ||
15.3.2 Periodic Countercurrent Chromatography | 335 | ||
15.3.3 Performance Comparison of Capture Processes | 335 | ||
15.4 Continuous mAb Polishing | 337 | ||
15.4.1 Multi-column Countercurrent Solvent Gradient Purification | 337 | ||
15.4.2 Integrated Protein PEGylation | 340 | ||
15.5 Conclusions | 341 | ||
References | 342 | ||
Chapter 16 - Intensification of Aqueous Two-phase Extraction for Protein Purification | 344 | ||
16.1 Introduction | 344 | ||
16.2 Thermodynamic Background | 345 | ||
16.2.1 ATPS Phase Equilibria | 345 | ||
16.2.2 Protein Partitioning | 346 | ||
16.2.2.1 Measurement of the Second Osmotic Virial Coefficient | 348 | ||
16.2.2.2 Measurement of Osmotic Cross Virial Coefficient | 349 | ||
16.2.3 Results–Estimating Partition Coefficients in ATPE | 350 | ||
16.3 Purification of Enzymes in a Mixer–Settler Apparatus | 352 | ||
16.3.1 Single Stage Experiments | 353 | ||
16.3.2 Multistage Experiments | 355 | ||
16.4 Centrifugal Partition Chromatography | 356 | ||
16.4.1 Experimental | 358 | ||
16.4.2 Results | 358 | ||
16.4.2.1 Hydrodynamic Behavior | 358 | ||
16.4.2.2 Activity Balance for Laccase Separation Using the Combination of a CPC and ATPE | 359 | ||
16.5 Conclusion | 361 | ||
References | 361 | ||
Chapter 17 - Intensification of Functional Foods Production | 365 | ||
17.1 Functional Foods | 365 | ||
17.2 Food Processes Intensification | 367 | ||
17.3 Process Intensification of Functional Foods Based on Nanodispersion Production | 368 | ||
17.3.1 Formation of Nanodispersions | 369 | ||
17.3.2 Classification of the Techniques for Production of Nanodispersions Based on Nature of Solvent Used | 370 | ||
17.3.2.1 Emulsification-evaporation Technique | 370 | ||
17.3.2.2 Emulsification–Diffusion Technique | 371 | ||
17.3.2.3 Solvent-displacement Technique | 371 | ||
17.3.3 Process Intensification | 372 | ||
17.4 Preparation of Functional Nano-sized Lycopene from Tomato Processing Wastes | 373 | ||
17.4.1 Materials and Methods | 373 | ||
17.4.2 Results and Discussions | 374 | ||
17.4.3 Conclusions | 377 | ||
17.5 Concluding Remarks | 377 | ||
Acknowledgements | 378 | ||
References | 378 | ||
Chapter 18 - Microwave-enhanced Extraction of Natural and Food Products: from Academia to Innovative and Large-scale Applications | 381 | ||
18.1 Introduction | 381 | ||
18.2 Microwave Heat Transfer | 382 | ||
18.3 Microwave-assisted Extraction | 383 | ||
18.3.1 Microwave Combined with Solvent Extraction | 384 | ||
18.3.1.1 Microwave-assisted Solvent Extraction (MASE) | 384 | ||
18.3.1.2 Vacuum Microwave-assisted Extraction (VMASE) | 384 | ||
18.3.1.3 Microwave Integrated Soxhlet Extraction (MIS) | 384 | ||
18.3.2 Microwave Extraction with Water Distillation | 385 | ||
18.3.2.1 Compressed Air Microwave Distillation (CAMD) | 385 | ||
18.3.2.2 Microwave Hydrodistillation (MWHD) | 385 | ||
18.3.2.3 Vacuum Microwave Hydrodistillation (VMHD) | 385 | ||
18.3.2.4 Microwave Accelerated Steam Distillation (MASD) | 385 | ||
18.3.3 Microwave Combined with Solvent-free Distillation | 386 | ||
18.3.3.1 Solvent-free Microwave-assisted Extraction (SFME) | 386 | ||
18.3.3.2 Vacuum Solvent-free Microwave-assisted Extraction (VSFME) | 386 | ||
18.3.4 Microwave-assisted Hydrodiffusion | 386 | ||
18.3.4.1 Microwave Hydrodiffusion and Gravity (MHG) | 386 | ||
18.3.4.2 Vacuum Microwave Hydrodiffusion and Gravity (VMHG) | 387 | ||
18.3.5 Microwave Steam Diffusion (MSDF) | 388 | ||
18.3.6 Industrial Application of Microwave-assisted Extraction | 389 | ||
18.4 Applications | 389 | ||
18.4.1 Antioxidants | 389 | ||
18.4.2 Flavors and Fragrances | 390 | ||
18.4.3 Natural Colors | 391 | ||
18.4.4 Fats and Oils | 392 | ||
18.5 Applications of Microwave Extraction in Industry | 394 | ||
Acknowledgements | 394 | ||
References | 394 | ||
Chapter 19 - Intensified Food Processing Through Membrane Operations | 397 | ||
19.1 Introduction | 397 | ||
19.2 Fundamentals of Membrane Operations | 398 | ||
19.2.1 Pressure-driven Membrane Operations | 398 | ||
19.2.2 Membrane Contactors | 402 | ||
19.2.3 Pervaporation | 405 | ||
19.2.4 Electrodialysis | 406 | ||
19.3 Integrated Membrane Operations in Food Processing | 407 | ||
19.3.1 Fruit Juice Processing | 407 | ||
19.3.2 Must and Wine Processing | 416 | ||
19.3.3 Milk and Whey Processing | 420 | ||
19.4 Conclusions | 424 | ||
References | 425 | ||
Chapter 20 - Intensified Brewing Systems | 430 | ||
20.1 Introduction | 430 | ||
20.2 Short Overview on Brewing | 431 | ||
20.2.1 Malting | 431 | ||
20.2.2 Mashing | 432 | ||
20.2.2.1 Traditional Equipment | 433 | ||
20.2.3 Wort Boiling | 434 | ||
20.2.3.1 Traditional Technology | 435 | ||
20.2.4 Fermentation and Maturation | 436 | ||
20.3 Bottlenecks to Process Intensification | 437 | ||
20.4 Intensification of Brewing Processes | 439 | ||
20.4.1 High Gravity Brewing | 439 | ||
20.4.2 Intensifying Enzymatic Activity in Mashing | 440 | ||
20.4.3 Intensification in Wort Treatment | 445 | ||
20.4.3.1 Pre-treatment of Hops | 445 | ||
20.4.3.2 Rectification Systems for Minimum Evaporation | 445 | ||
20.4.3.3 Specific Technologies for Volatile Removal | 446 | ||
20.4.4 Fermentation and Maturation | 447 | ||
20.4.4.1 Continuous Fermentation and Maturation | 448 | ||
20.4.4.2 Yeast Modification | 448 | ||
20.4.4.3 Additives to Maturation | 449 | ||
20.4.5 Pasteurization | 449 | ||
20.4.6 Deaeration of Water | 449 | ||
20.4.7 Dealcoholization | 450 | ||
20.4.8 From Batch to Continuous | 451 | ||
20.4.9 Synergy in Process Steps | 452 | ||
20.5 New Energy Concepts Based on Intensified Brewing Systems | 452 | ||
20.5.1 Low Temperature Heat Supply and Heat Integration | 452 | ||
20.5.2 Hydrodynamic Cavitation in Brewing | 457 | ||
References | 457 | ||
Chapter 21 - Novel Processing Concepts for Making Fibrous Food Products | 462 | ||
21.1 Introduction | 462 | ||
21.2 Current Processes for Making Meat Analogs | 464 | ||
21.2.1 Mixing Proteins with Hydrocolloids | 464 | ||
21.2.2 Extrusion | 465 | ||
21.2.3 Mycoprotein | 466 | ||
21.3 Novel Processing Concepts | 466 | ||
21.3.1 Spinning | 466 | ||
21.3.2 Cultured Meat | 468 | ||
21.3.3 Structuring with Simple Shear Flow | 468 | ||
21.4 Comparing Structuring Methods | 470 | ||
21.5 Ingredients for Meat Analogs | 471 | ||
21.5.1 Plant Proteins | 472 | ||
21.5.2 Solidification Properties | 472 | ||
21.5.3 Minor Components for Taste and Color | 473 | ||
21.5.4 Fat or Oil | 474 | ||
21.6 Future Outlook | 474 | ||
References | 475 | ||
Chapter 22 - The Usefulness of Direct Digital Manufacturing for Biomedical Applications | 478 | ||
22.1 Introduction | 478 | ||
22.2 General Overview | 480 | ||
22.3 DDM for Biomedical Applications | 481 | ||
22.3.1 DDM for Pharmaceutics | 481 | ||
22.3.2 DDM for Surgical Planning and Training | 482 | ||
22.3.3 DDM for Tissue Engineering | 483 | ||
22.4 Conclusion | 485 | ||
Acknowledgements | 485 | ||
References | 485 | ||
Chapter 23 - Alternative Energy Forms in Manufacturing, Processing and Applications of Biopolymers and Biomaterials | 488 | ||
23.1 Introduction | 488 | ||
23.2 Electric Fields | 491 | ||
23.3 Magnetic Fields | 492 | ||
23.4 Microwave Fields | 494 | ||
23.5 Plasma | 497 | ||
23.6 Acoustic Fields | 497 | ||
23.7 Energy of Light | 500 | ||
23.8 Concluding Remarks – From Lab-scale Research to Commercial-scale Manufacturing | 501 | ||
References | 502 | ||
Subject Index | 507 |