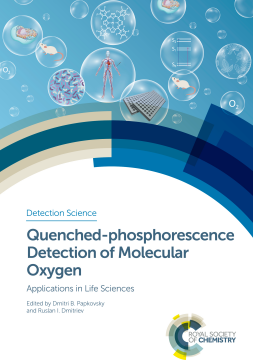
BOOK
Quenched-phosphorescence Detection of Molecular Oxygen
Dmitri B Papkovsky | Ruslan I Dmitriev
(2018)
Additional Information
Book Details
Abstract
Significant progress has been made in recent years in quenched-phosphorescence oxygen sensing, particularly in the materials and applications of this detection technology that are open to commercialization, like uses in brain imaging and food packaging. Prompted by this, the editors have delivered a dedicated book that brings together these developments, provides a comprehensive overview of the different detection methodologies, and representative examples and applications.
This book is intended to attract new researchers from various disciplines such as chemistry, physics, biology and medicine, stimulate further progress in the field and assist in developing new applications. Providing a concise summary at the cutting edge, this practical guide for current experts and new potential users will increase awareness of this versatile sensing technology.
Table of Contents
Section Title | Page | Action | Price |
---|---|---|---|
Front Cover | Cover | ||
Quenched-phosphorescence Detection of Molecular Oxygen: Applications in Life Sciences | i | ||
Preface | v | ||
Contents | vii | ||
Chapter 1 - Fundamentals of Quenched Phosphorescence O2 Sensing and Rational Design of Sensor Materials | 1 | ||
1.1 Introduction | 1 | ||
1.2 Mechanism of Oxygen Quenching | 2 | ||
1.3 Requirements for Phosphorescent Indicators | 4 | ||
1.4 Brief Overview of the Most Common Indicators | 6 | ||
1.5 Rational Design of Optical Sensing Materials | 9 | ||
1.6 Sensitivity and Dynamic Range of Oxygen Sensors | 11 | ||
1.7 Referenced Oxygen Sensing and Imaging | 13 | ||
1.8 Artefacts in Oxygen Sensing | 14 | ||
1.9 Conclusions and Outlook | 16 | ||
References | 16 | ||
Chapter 2 - New Polymer-based Sensor Materials and Fabrication Technologies for Large-scale Applications | 19 | ||
2.1 Introduction | 19 | ||
2.2 Physical Entrapment of Phosphors Within Inorganic and Organic Matrices | 21 | ||
2.2.1 Casting of Polymer ‘Cocktails’ | 25 | ||
2.2.2 Physical Mixing of Phosphors with Ormosils | 29 | ||
2.2.3 Phosphor Integration in Silicone Rubbers | 30 | ||
2.2.4 Impregnation of Microporous Membranes and Microparticles with Phosphor Molecules | 32 | ||
2.2.5 Solvent Crazing | 33 | ||
2.2.6 Electrospinning | 34 | ||
2.2.7 Electrophoretic Deposition | 35 | ||
2.2.8 Layer-by-layer Deposition (LbL) | 35 | ||
2.3 Covalent and Coordinative Binding of Phosphors to Substrates | 36 | ||
2.4 Conclusions and Outlook | 38 | ||
Abbreviations | 39 | ||
Acknowledgement | 39 | ||
References | 40 | ||
Chapter 3 - Evolution of Cell-penetrating Phosphorescent O2 Probes | 50 | ||
3.1 Evolution of Cell-penetrating Phosphorescent O2 probes | 50 | ||
3.1.1 Introduction | 50 | ||
3.1.2 Why and How to Measure Intracellular O2 | 52 | ||
3.1.3 Different Classes of Cell-penetrating O2 Probes and Their Evolution | 55 | ||
3.1.3.1 Small Molecule Probes and Other Conjugates | 55 | ||
3.1.3.2 Nanoparticles of First Generation | 59 | ||
3.1.3.3 Nanoparticles of Second Generation | 60 | ||
3.1.3.4 Nanoparticles of Third Generation | 62 | ||
3.2 Conclusions and Future Outlook | 63 | ||
Acknowledgement | 64 | ||
References | 64 | ||
Chapter 4 - Hydrophilic Ir(iii) Complexes for In vitro and In vivo Oxygen Imaging | 71 | ||
4.1 Introduction | 71 | ||
4.2 Experimental | 73 | ||
4.2.1 Synthesis of BTP-PEGn (n = 12, 24, 48) and PPY-PEG24 | 73 | ||
4.2.2 Photophysical Properties in Aqueous Solutions | 76 | ||
4.2.3 Octanol/Water Partition Coefficients (logPO/W) | 76 | ||
4.2.4 Fluorescence and Phosphorescence Lifetime Imaging Microscopy | 77 | ||
4.2.5 Cell Culture and Imaging | 77 | ||
4.2.6 In vivo Imaging | 77 | ||
4.3 Results and Discussion | 78 | ||
4.3.1 Electronic Structures of the Ir(iii) Complexes | 78 | ||
4.3.2 Hydrophilicity and Photophysical Properties in Water | 79 | ||
4.3.3 Phosphorescence Quenching by Molecular Oxygen in Solution | 81 | ||
4.3.4 Temperature Effects on Phosphorescence Quenching by O2 | 83 | ||
4.3.5 Cellular Uptake and Oxygen Response | 84 | ||
4.3.6 In vivo O2 Imaging by PLIM Measurements | 86 | ||
4.4 Conclusion | 89 | ||
Acknowledgement | 89 | ||
References | 89 | ||
Chapter 5 - Protection of Triplet Excited State Materials from Oxygen Quenching and Photooxidation in Optical Sensing Applications | 91 | ||
5.1 Introduction | 91 | ||
5.2 Phosphorescent Probes with Appended Protective Groups | 93 | ||
5.2.1 Phosphorescent Dendrimers | 93 | ||
5.2.2 “Self-healing” Phosphorescent Complexes | 97 | ||
5.3 Host-guest Complexes and Aggregates | 99 | ||
5.3.1 Tryptophan Phosphorescence in Proteins | 99 | ||
5.3.2 Phosphorescence of Cyclodextrin Complexes in the Presence of Oxygen | 100 | ||
5.3.3 Steroids as Protective Matrixes | 103 | ||
5.3.4 Gel Matrixes | 104 | ||
5.4 Application of Oxygen Scavengers | 105 | ||
5.4.1 Inorganic Oxygen Scavengers | 105 | ||
5.4.2 Application of Natural Antioxidants | 106 | ||
5.4.3 Scavengers of Singlet Oxygen | 107 | ||
5.5 Encapsulation of the Phosphorescent Molecules into Polymers | 108 | ||
5.6 Inorganic Matrix Materials | 110 | ||
5.7 Conclusions and Perspectives | 111 | ||
Acknowledgements | 112 | ||
References | 112 | ||
Chapter 6 - Progress in Phosphorescence Lifetime Measurement Instrumentation for Oxygen Sensing | 117 | ||
6.1 Introduction | 117 | ||
6.2 The Phosphorescence Emission as a Linear System | 120 | ||
6.2.1 First Order Phosphorescent Systems | 120 | ||
6.2.2 Multi-exponential Phosphorescent Systems | 121 | ||
6.2.3 Lifetime Derived from Modulation Factor and Phase-shift | 122 | ||
6.2.4 Modelling and Calibration of Phosphorescent Systems | 123 | ||
6.3 Architecture for Measuring the Frequency Response | 123 | ||
6.3.1 Modular Architecture of the Phosphorescence Instrument | 124 | ||
6.3.2 Estimation of the Frequency Response | 125 | ||
6.4 Multifrequency Measurements and Applications | 127 | ||
6.4.1 Characterization of the Sensing Phase | 128 | ||
6.4.2 Selecting the Optimal Single Modulation Frequency for Analyte Determination | 130 | ||
6.4.3 Using Multi-frequency Information for Oxygen Determination | 131 | ||
6.5 Noise Analysis and Applications | 134 | ||
6.5.1 Application to Uncertainty Estimation | 136 | ||
6.5.2 Application to Optimal Combination of Harmonics | 137 | ||
6.6 Instrument Development | 137 | ||
6.7 Conclusions | 141 | ||
Acknowledgement | 142 | ||
References | 142 | ||
Chapter 7 - Optical O2 Sensing in Aquatic Systems and Organisms | 145 | ||
7.1 Introduction | 145 | ||
7.2 Optical O2 Sensing Platforms | 146 | ||
7.2.1 Fiber-optic O2 Opt(r)odes | 147 | ||
7.2.2 Planar Opt(r)odes for O2 Imaging and Remote Read-out | 148 | ||
7.2.3 Particle-based Optical O2 Sensors | 150 | ||
7.3 Challenges Related to Optical O2 Measurements in Aquatic Systems | 151 | ||
7.3.1 Large O2 Dynamics | 151 | ||
7.3.2 Extreme Environments Require Special Designs | 152 | ||
7.3.3 Sensor Stability – from Mechanical Stress to Biofouling | 154 | ||
7.4 Applications of Optical O2 Sensors | 155 | ||
7.4.1 Application of Micro-opt(r)odes | 155 | ||
7.4.1.1 Profiling | 156 | ||
7.4.1.2 Eddy Correlation Spectroscopy | 156 | ||
7.4.2 Applications of Bulk Sensors and Sensor Patches | 157 | ||
7.4.2.1 Respirometry | 158 | ||
7.4.2.2 Environmental Monitoring | 159 | ||
7.4.3 Chemical Imaging | 159 | ||
7.4.3.1 Microscopic O2 Imaging | 161 | ||
7.4.3.2 O2 Imaging in Sediments | 163 | ||
7.4.3.3 O2 Imaging on Structurally Complex Samples | 165 | ||
7.5 Future Challenges | 167 | ||
7.5.1 Multi-analyte Measurements | 167 | ||
7.5.2 Special O2 Microniches and Their Impact on Other Processes | 168 | ||
7.5.3 Low Cost Instrumentation for Long-term Monitoring | 168 | ||
7.6 Conclusions | 168 | ||
References | 169 | ||
Chapter 8 - Monitoring of Extracellular and Intracellular O2 on a Time-resolved Fluorescence Plate Reader | 175 | ||
8.1 Introduction | 175 | ||
8.2 Measuring Oxygen | 176 | ||
8.2.1 Clark Electrodes | 176 | ||
8.2.2 Phosphorescent Porphyrin-based Probes | 176 | ||
8.2.2.1 Seahorse XF Analyser | 177 | ||
8.2.3 Oxygen Sensing Plate Based Assays Using Standard Tissue Culture Plates | 179 | ||
8.2.3.1 MitoXpress® Oxygen Probes | 180 | ||
8.2.3.2 MitoXpress-Xtra | 181 | ||
8.3 Measurement of Intracellular Oxygen | 183 | ||
8.3.1 MitoXpress-Intra Probe | 184 | ||
8.3.2 Application of the MitoXpress-Intra Probe | 187 | ||
8.4 Future Applications of Plate-based Oxygen Monitoring Systems | 188 | ||
Acknowledgements | 190 | ||
References | 190 | ||
Chapter 9 - Monitoring Parameters of Oxygen Transport to Cells in the Microcirculation | 193 | ||
9.1 Introduction | 193 | ||
References | 203 | ||
Chapter 10 - Photoacoustic Imaging of Oxygen | 205 | ||
10.1 Introduction | 205 | ||
10.2 Photoacoustic Oxygen Monitoring Using Biological Chromophores (i.e. Hemoglobin) | 207 | ||
10.3 Photoacoustic Lifetime Measurements for Oxygen Sensing (PALT) | 209 | ||
10.4 Applying Nanotechnology to PALT | 213 | ||
10.5 Summary and Discussion | 215 | ||
Acknowledgements | 217 | ||
References | 217 | ||
Chapter 11 - Imaging of Tissue Oxygen Ex vivo | 220 | ||
11.1 Introduction | 220 | ||
11.2 Experimental | 222 | ||
11.2.1 Materials | 222 | ||
11.2.2 Animals and Tissue Staining | 222 | ||
11.2.3 Live Tissue Imaging by Confocal FLIM/PLIM Microscopy | 223 | ||
11.2.4 Analysis of Oxygen Consumption Rate (OCR) in Colonic Mucosa Samples | 223 | ||
11.2.5 Statistical Analysis and Data Presentation | 224 | ||
11.3 Results and Discussion | 224 | ||
11.3.1 Oxygen Imaging in the Colon Tissue | 224 | ||
11.3.1.1 Staining of Colonic Tissue with O2 Sensing Probes | 225 | ||
11.3.1.2 Imaging of O2 in Colonic Tissue | 225 | ||
11.3.1.3 Effect of DSS-induced Colitis on O2 Levels and OCR in Mouse Colonocytes | 228 | ||
11.3.1.4 Effect of NOX Deficiency on O2 Levels in Mouse Colonic Epithelium | 228 | ||
11.3.1.5 O2 Imaging in Rat and Human Colonic Epithelium | 229 | ||
11.3.1.6 Main Findings and Conclusions | 230 | ||
11.3.2 Ex vivo O2 Imaging in the Urinary Bladder | 232 | ||
11.3.2.1 Ex vivo and in vivo Staining of the Urinary Bladder Epithelium with Pt-Glc and Other Probes | 233 | ||
11.3.2.2 Ex vivo Imaging of Intracellular O2 Gradients in Umbrella Cells | 234 | ||
11.3.2.3 Ex vivo Imaging of O2 in Other Tissue Types of the Urinary Bladder | 237 | ||
11.3.2.4 Main Findings and Conclusions | 237 | ||
11.3.3 Ex vivo O2 Imaging in Carotid Arteries | 238 | ||
11.4 Concluding Remarks | 240 | ||
Acknowledgements | 241 | ||
References | 241 | ||
Chapter 12 - Tracking of Hypoxia and Cancer Metastasis with Iridium(iii)-based O2 Probes | 244 | ||
12.1 Introduction | 244 | ||
12.2 Hypoxia Imaging with Poly(N-Vinylpyrrolidone) (PVP)-conjugated Ir(iii) Complex Probe (Ir-PVP) | 245 | ||
12.2.1 Design of the Ir-PVP Probe | 245 | ||
12.2.2 Ratiometric Imaging of Hypoxia in Solid Tumours with Ir-PVP Probe | 247 | ||
12.2.3 Monitoring the Proliferation of Cancer Cells in Live Mice with Ir-PVP Probe | 247 | ||
12.3 Tracking Cancer Metastasis with Ir(iii)-based Oxygen Nanosensor | 249 | ||
12.3.1 Design of the Ir(iii)-based Oxygen Nanosensor (Ir-CM) | 249 | ||
12.3.2 Tracking Lung Cancer Metastasis with Ir-CM Nanosensor | 249 | ||
12.3.3 Tracking Cancer Metastasis in the Lymph Node with Ir-CM Nanosensor | 249 | ||
12.4 Ir(iii)-based Successively Activating Phosphorescent Probe for Ultrasensitive Hypoxia Imaging | 253 | ||
12.4.1 Design of the Successively Activating Phosphorescent Probe (Ir-Im-PEG) | 253 | ||
12.4.2 Tracking Liver Cancer Metastasis with the Ir-Im-PEG Probe | 254 | ||
12.5 Conclusion and Perspective | 256 | ||
Acknowledgement | 256 | ||
References | 257 | ||
Chapter 13 - Probing Tissue Oxygenation by Delayed Fluorescence of Protoporphyrin IX | 259 | ||
13.1 Introduction | 259 | ||
13.2 Background | 260 | ||
13.2.1 Oxygen | 260 | ||
13.2.2 Porphyrins | 261 | ||
13.2.3 Protoporphyrin IX | 261 | ||
13.2.4 Delayed Fluorescence Quenching | 262 | ||
13.2.5 Quenching Constants | 264 | ||
13.3 Measuring Mitochondrial PO2 | 265 | ||
13.3.1 Aminolevulinic Acid | 265 | ||
13.3.2 Laboratory Setup | 266 | ||
13.3.2.1 Excitation Source | 266 | ||
13.3.2.2 Gated Detector | 266 | ||
13.3.2.3 Data-acquisition System | 267 | ||
13.3.2.4 Signal Analysis | 267 | ||
13.3.2.5 Multi Compartment Measurements | 269 | ||
13.4 In vivo Cellular Respirometry | 270 | ||
13.5 Clinical Implementation | 271 | ||
13.5.1 The Skin as a “Canary” of the Body | 271 | ||
13.5.2 Priming of the Skin | 272 | ||
13.5.3 The COMET Measuring System | 272 | ||
13.5.4 Example Measurements | 273 | ||
13.6 Conclusion | 275 | ||
Conflict of Interest Statement | 275 | ||
References | 276 | ||
Chapter 14 - Microfluidic Systems and Optical Oxygen Sensors: A Perfect Match for Advancing Bioprocessing and Microbiology | 278 | ||
14.1 Introduction | 278 | ||
14.2 Challenges | 282 | ||
14.3 Sensor Formats | 284 | ||
14.3.1 Water-soluble/Macromolecular Probes | 285 | ||
14.3.2 Sensor Layers | 285 | ||
14.3.3 Micro/Nanoparticles | 285 | ||
14.4 Sensor Layer Integration and Fabrication | 286 | ||
14.5 Detection Principles | 289 | ||
14.6 Applications | 291 | ||
14.7 Conclusion | 293 | ||
References | 294 | ||
Chapter 15 - pO2 Measurements in Biological Tissues by Luminescence Lifetime Spectroscopy: Strategies to Exploit or Minimize Phototoxic Effects in Tumors | 298 | ||
15.1 Introduction | 298 | ||
15.2 Assessment of the Tumor Oxygenation with the Phosphorescence Quenching-based Approach | 300 | ||
15.3 Photodynamic Therapy | 301 | ||
15.4 Oxygen Consumption During Photodynamic Therapy | 305 | ||
15.5 Strategies to Minimize Tissue Damage While Measuring the pO2 | 308 | ||
15.5.1 Impact of the Biodistribution and Subcellular Localization of Oxygen-sensitive Molecules on Their Phototoxicity | 308 | ||
15.5.2 Correlation Between the Production of ROS and the Phototoxicity While Measuring the pO2 | 310 | ||
15.5.3 Alteration of pO2 Measurements by the Luminescence of Oxygen Molecular Probes Photoproducts | 311 | ||
15.6 Conclusion | 313 | ||
Acknowledgement | 314 | ||
References | 314 | ||
Chapter 16 - In vivo Brain Functional Imaging Using Oxygenation-related Optical Signal | 319 | ||
16.1 Introduction | 319 | ||
16.2 Intrinsic Optical Signal (IOS) | 321 | ||
16.3 Functional Magnetic Resonance Imaging (fMRI) | 322 | ||
16.4 Functional Near-Infrared Spectroscopy (fNIRS) | 323 | ||
16.5 Diffuse Optical Tomography (DOT) | 324 | ||
16.6 Photoacoustic Imaging (PAI) | 324 | ||
16.7 Phosphorescence Quenching Techniques | 326 | ||
16.7.1 Soluble Phosphorescent Oxygen-sensitive Probes | 326 | ||
16.7.2 Phosphorescent Microparticle-based Probes | 329 | ||
16.7.3 Planar Phosphorescent Oxygen Sensors | 329 | ||
16.8 Conclusions | 330 | ||
References | 331 | ||
Chapter 17 - Applications of Phosphorescent O2 Sensors in Food and Beverage Packaging Systems | 335 | ||
17.1 Introduction | 335 | ||
17.2 Monitoring O2 Content in Modified Atmosphere Packaging of Foodstuffs | 340 | ||
17.2.1 Selection of Oxygen Sensors for Food Packaging Applications | 342 | ||
17.2.2 Characterisation of O2 Sensors | 342 | ||
17.2.3 Instruments Used to Monitor O2 on Food Packaging Integrated with O2 Sensors | 343 | ||
17.2.4 Applications of O2 Sensors for Monitoring O2 Content in Packaged Food Products | 344 | ||
17.3 Conclusions | 357 | ||
Acknowledgements | 357 | ||
References | 357 | ||
Subject Index | 361 |