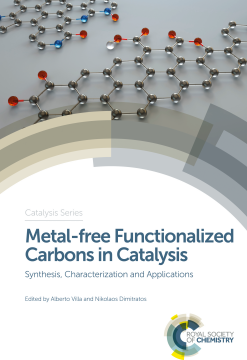
Additional Information
Book Details
Abstract
Metal-free carbons have recently shown great efficiency in several catalytic processes, including oxidative dehydrogenation (ODH) of ethylbenzene and alkenes, hydrogen evolution, liquid Brønsted and Lewis acid catalysis and electrochemical reactions. The catalytic activities of carbon materials are intimately related to their defects, structures, and surface chemistry. In particular, nitrogen functionalized carbons present different surface functional groups, and they can be used as multifunctional catalysts, either through their electronic or nucleophilic properties, or their ability to form additional H bonds with substrates.
This book provides an overview of the preparation, characterization and application of metal-free functionalized carbons, including carbon nanotubes, graphene, carbon nitride and covalent organic frameworks (COFs). It is ideal for researchers and industrialists working in catalysis, gas sensing and carbon dioxide storage.
Table of Contents
Section Title | Page | Action | Price |
---|---|---|---|
Front Cover | Cover | ||
Metal-free Functionalized Carbons in Catalysis: Synthesis, Characterization and Applications | i | ||
Preface | vii | ||
Contents | ix | ||
Section I - Synthesis | 1 | ||
Chapter 1 - Covalent Methods for Functional Carbons’ Synthesis | 3 | ||
1.1 Towards a Molecular Understanding of the Reactivity of Carbon Surfaces | 3 | ||
1.1.1 Role of Curvature and Topological Defects on Surface Reactivity | 3 | ||
1.1.2 Surface Modification through Heteroatom Insertion | 4 | ||
1.2 Strategies for the Synthesis of Functional Carbon Catalysts | 6 | ||
1.2.1 Catalytic Chemical Vapor Deposition | 6 | ||
1.2.2 Post-synthetic Functionalization | 7 | ||
1.2.3 Tethering Discrete Active Sites Through Nanomaterials’ Surface Functionalization | 11 | ||
1.3 Oxidized Carbon Nanomaterials in Catalysis | 11 | ||
1.3.1 Oxidation Reactions | 12 | ||
1.3.2 Reduction Reactions | 14 | ||
1.3.3 C–C and C–N Bond Forming Reactions | 15 | ||
1.3.4 Cycloaddition and Polymerization Reactions | 17 | ||
1.4 Tuning Chemical and Electronic Surface Properties of Carbon Nanomaterials in Catalysis via Tailored Chemical Functionalizatio... | 18 | ||
1.4.1 Acid- and Base-catalyzed Reactions with Functionalized Nanocarbons | 18 | ||
1.4.2 Chemically Decorated Nanocarbons with Tailored Functional Groups for the Electrochemical Oxygen Reduction Reaction (ORR) | 21 | ||
1.5 Conclusion | 21 | ||
Acknowledgements | 22 | ||
References | 23 | ||
Chapter 2 - Non-covalent Methodologies for the Preparation of Metal-free Nanocarbons for Catalysis | 29 | ||
2.1 Introduction | 29 | ||
2.2 Graphene | 31 | ||
2.2.1 Functionalization via Physisorption | 31 | ||
2.2.1.1 Small Molecules | 31 | ||
2.2.1.2 Polymers | 33 | ||
2.2.2 Graphene/Polymer Assemblies by In situ Polymerization | 36 | ||
2.2.3 Preparation of Porous Supramolecular Architectures | 38 | ||
2.2.3.1 Soft Templates | 38 | ||
2.2.3.2 Hard Templates | 40 | ||
2.2.4 Other Approaches | 40 | ||
2.3 Fullerenes | 43 | ||
2.4 Carbon Nanotubes | 45 | ||
2.4.1 Solubilization of CNTs and Physisorption of Small Molecules | 45 | ||
2.4.2 Functionalization with Polymers | 46 | ||
2.4.3 Encapsulation of Molecules (Peapods) | 47 | ||
2.4.4 Functionalization with Other Graphitic Carbons | 48 | ||
2.4.5 Doping of CNTs | 49 | ||
2.5 Carbon Nanohorns | 51 | ||
2.6 Nanodiamonds | 52 | ||
2.7 Carbon Dots | 54 | ||
2.7.1 Functionalization via Physisorption | 54 | ||
2.7.2 Functionalization with Graphitic Nanostructures | 55 | ||
2.8 Conclusions | 59 | ||
References | 60 | ||
Chapter 3 - Carbon Nitrides (g-C3N4) and Covalent Triazine Frameworks (CTFs) | 67 | ||
3.1 Introduction | 67 | ||
3.2 Methods of Synthesis of g-C3N4 | 70 | ||
3.2.1 Bulk g-C3N4 | 70 | ||
3.2.2 Template-assisted Method | 72 | ||
3.2.2.1 Hard Template Approach | 72 | ||
3.2.2.1.1\rMesoporous g-C3N4.Initial reports on mesoporous graphitic carbon nitride (mpg-C3N4) showed that it was synthesized by nanocastin... | 72 | ||
3.2.2.1.2\rg-C3N4 Nanosheets and Thin Films.A novel, size-controllable synthesis of graphitic carbon nitride nanosheets (g-CNNSs) using sme... | 74 | ||
3.2.2.1.3\rOne-dimensional g-C3N4 Nanowires/Nanorods/Nanotubes.One-dimensional nanostructured g-C3N4 has its own place in nanoscience and t... | 75 | ||
3.2.2.2 Soft Template Approach | 76 | ||
3.2.2.3 Supramolecular Preorganization Method | 76 | ||
3.2.3 Ionothermal Approach | 79 | ||
3.2.4 Bottom-up and Top-down Strategies | 79 | ||
3.3 Methods of Synthesis of Covalent Triazine Frameworks (CTFs) | 81 | ||
3.3.1 Ionothermal Synthesis | 81 | ||
3.3.2 Low-temperature and Microwave-assisted Synthesis | 83 | ||
3.3.3 Solid-state Synthetic Method | 85 | ||
3.4 Miscellaneous | 86 | ||
3.4.1 Heteroatom Doping | 87 | ||
3.4.1.1 Non-metal Doping | 87 | ||
3.4.1.1.1\rNitrogen Doping.Polycondensation of precursors such as melamine with a nitrogen-rich additive of hydrazine hydrate provides nitr... | 87 | ||
3.4.1.1.2\rOxygen Doping.Mei et al. described a polycondensation reaction combined with a solution mixing pathway using melamine and cyanur... | 87 | ||
3.4.1.1.3 Sulfur Doping.Wang et al. demonstrated that sulfur-doped g-C3N4 was fabricated using thiourea by a simple calcination at 520 °C.... | 88 | ||
3.4.1.1.4\rPhosphorus Doping.The introduction of phosphorus into the structural framework of g-C3N4 would lead to customized electronic pro... | 88 | ||
3.4.1.1.5\rHalogen-modified g-C3N4.Iodine-modified g-C3N4 and iodinated g-C3N4 nanosheets have been synthesized by following co-condensatio... | 89 | ||
3.4.1.2 Metal Doping and Heterostructure Formation | 89 | ||
3.5 Remarks and Future Prospects | 90 | ||
References | 91 | ||
Section II - Characterization | 103 | ||
Chapter 4 - Raman, IR and INS Characterization of Functionalized Carbon Materials | 105 | ||
4.1 Raman Spectroscopy Applied to Carbons | 105 | ||
4.1.1 Raman Spectroscopy of Perfect and Defective Graphite | 106 | ||
4.1.2 Raman Spectroscopy of Activated Carbons | 107 | ||
4.1.3 Raman Spectroscopy of Graphene, Graphene Oxide and Reduced Graphene Oxide | 108 | ||
4.1.4 Raman Spectroscopy of Carbon Nanotubes (CNTs) | 109 | ||
4.1.5 Raman Spectroscopy of Other C-containing Materials | 110 | ||
4.2 IR Spectroscopy Applied to Carbons: Principles, Problems and Solutions | 111 | ||
4.2.1 FT-IR Spectroscopy of Activated Carbons | 114 | ||
4.2.2 FT-IR Spectroscopy of GO, RGO, and Doped GO | 117 | ||
4.2.3 FT-IR Spectroscopy of CNTs | 119 | ||
4.2.4 FT-IR Spectroscopy of Fullerenes | 120 | ||
4.2.5 FT-IR Spectroscopy of Other Carbon-based Materials | 121 | ||
4.3 Neutron Properties, and Inelastic Neutron Scattering Applied to Carbons | 125 | ||
4.3.1 INS Spectra of Activated Carbons | 126 | ||
4.3.2 INS Spectroscopy of Other Carbonaceous Materials | 129 | ||
4.4 Conclusions and Future Perspectives | 131 | ||
References | 131 | ||
Chapter 5 - XPS Characterization of Metal-free Functionalized Carbons | 138 | ||
5.1 Introduction | 138 | ||
5.1.1 Physical Principles and Practical Aspects of XPS | 139 | ||
5.1.2 XPS Instrumentation | 142 | ||
5.1.3 XPS Peak Characteristics, Chemical Shift and Quantification | 143 | ||
5.2 Analysis of the C 1s Spectra of Carbonaceous Materials | 146 | ||
5.2.1 Peak Fitting or Peak Deconvolution Procedure | 148 | ||
5.2.2 Use of a Difference C 1s Spectra Peak Fitting Procedure | 150 | ||
5.3 XPS Studies of Pristine Carbon Materials | 150 | ||
5.4 XPS Characterization of Defects on Carbon Materials | 154 | ||
5.5 XPS Characterization of Carbon Materials with Oxygen Functional Groups | 156 | ||
5.6 XPS Characterization of Carbon Materials with Heteroatom Dopants | 158 | ||
5.6.1 XPS Characterization of Carbon Materials Doped with Nitrogen | 159 | ||
5.6.2 XPS Characterization of Carbon Materials with Other Heteroatoms | 162 | ||
5.7 Conclusions | 166 | ||
References | 166 | ||
Section III - Applications | 177 | ||
Chapter 6 - Metal-free Functionalized Carbon in Liquid Phase Reactions | 179 | ||
6.1 Introduction | 179 | ||
6.2 Hydrocarbon Oxidation | 180 | ||
6.2.1 Cyclohexane Oxidation | 181 | ||
6.2.2 Ethylbenzene Oxidation | 182 | ||
6.2.3 Selective Oxidation of Toluene | 183 | ||
6.3 Alcohol Oxidation | 184 | ||
6.4 Acid or Base Catalysis | 186 | ||
6.4.1 Knoevenagel Condensation Reaction | 186 | ||
6.4.2 Transesterification | 187 | ||
6.4.3 Hydrolysis Reactions | 188 | ||
6.5 Coupling Reactions | 189 | ||
6.6 Reduction Reactions | 190 | ||
6.7 Discussion | 191 | ||
References | 193 | ||
Chapter 7 - Carbon-based Metal-free Catalysts for Dehydrogenation of Hydrocarbons | 196 | ||
7.1 Introduction | 196 | ||
7.2 Oxidative Dehydrogenation of Ethylbenzene | 198 | ||
7.3 Catalytic Oxidation of Alkenes and Alkanes | 212 | ||
7.4 Direct Dehydrogenation | 219 | ||
7.5 Conclusion | 222 | ||
Acknowledgements | 222 | ||
References | 223 | ||
Chapter 8 - Electrochemistry Reactions Catalyzed by Metal-free Functionalized Carbons | 228 | ||
8.1 Introduction | 228 | ||
8.2 Oxygen Electrochemistry Reactions | 230 | ||
8.3 Metal-free Functionalized Carbons for ORR Catalysis | 231 | ||
8.3.1 Heteroatom or Molecular Doping | 232 | ||
8.3.1.1 Nitrogen-doped Nanocarbon | 232 | ||
8.3.1.2 Activity Descriptor | 234 | ||
8.3.1.3 Molecular Doping | 236 | ||
8.3.2 Edge Effects or Edge Sites | 236 | ||
8.3.3 Intrinsic Topological Defects | 239 | ||
8.3.3.1 Theoretical Investigation of the Role of Defects | 240 | ||
8.3.3.2 Defective Nanocarbons as Superior ORR Catalysts | 241 | ||
8.3.3.3 Correlation Among Dopants, Edges, and Defects | 244 | ||
8.4 Metal-free Functionalized Carbons for OER Catalysis | 247 | ||
8.4.1 N-doped Carbons for OER Catalysis | 247 | ||
8.4.2 Oxidized Carbons for OER Catalysis | 249 | ||
8.4.3 Dual-doped Carbons for OER Catalysis | 250 | ||
8.5 Multifunctional Metal-free Carbon Catalysts | 253 | ||
8.5.1 ORR/OER Bifunctional Catalysts | 253 | ||
8.5.1.1 N-doped Carbons | 253 | ||
8.5.1.2 Dual-doped Carbons | 255 | ||
8.5.2 HER-containing Multifunctional Catalysts | 257 | ||
8.6 Conclusions | 259 | ||
Acknowledgements | 260 | ||
References | 260 | ||
Chapter 9 - Metal-free Functionalised Carbons in Photocatalysis | 266 | ||
9.1 Overview, Basic Concepts, and Key Terminologies | 266 | ||
9.1.1 Overview | 266 | ||
9.1.2 Key Terminologies | 269 | ||
9.2 Organic Polymer Photocatalysts | 270 | ||
9.2.1 Linear Structured Polymers | 270 | ||
9.2.2 Polymeric Networks | 273 | ||
9.2.3 Covalent Organic Frameworks | 277 | ||
9.3 Graphitic Carbon Nitrides (g-C3N4) | 279 | ||
9.3.1 Geometric and Electronic Structures | 280 | ||
9.3.2 Pristine Bulk g-C3N4 | 282 | ||
9.3.3 Modified g-C3N4 and Carbon Nitride Derivatives | 286 | ||
9.3.3.1 Porous Structure | 286 | ||
9.3.3.2 Shape Engineering of g-C3N4 | 288 | ||
9.3.3.3 Doping and Surface Engineering | 291 | ||
9.3.3.4 Carbon Nitride Derivatives | 296 | ||
9.3.4 Other Applications Beyond Photocatalytic HER | 298 | ||
9.4 Concluding Remarks and Perspectives | 300 | ||
Acknowledgements | 300 | ||
References | 300 | ||
Chapter 10 - Metal-free Doped Carbons for Electroanalytical Sensors | 304 | ||
10.1 Introduction | 304 | ||
10.2 Modified Electrode Fabrication | 306 | ||
10.3 Carbon Materials for the Preparation of Modified Electrodes | 310 | ||
10.3.1 Carbon Paste and Ordered Carbons | 310 | ||
10.3.2 Glassy Carbon (GC), Boron-doped Diamond (BDD) and Tetrahedral Amorphous Carbon (ta-C) | 311 | ||
10.3.3 Carbon Nanotubes (CNTs) | 313 | ||
10.3.3.1 Metal-free Functionalized Carbon Nanotubes | 313 | ||
10.3.3.2 Oxygen-doped CNTs | 314 | ||
10.3.3.3 Nitrogen-doped CNTs | 315 | ||
10.3.3.4 Boron-doped CNTs | 315 | ||
10.3.3.5 Phosphorus-doped CNTs | 316 | ||
10.3.4 Graphene | 316 | ||
10.3.4.1 Advantages of the Use of Graphene | 316 | ||
10.3.4.2 Metal-free Graphene-based Materials | 316 | ||
10.3.4.3 Boron-doped Graphene | 317 | ||
10.3.4.4 Nitrogen-doped Graphene | 317 | ||
10.3.4.5 Oxygen-doped Graphene | 319 | ||
10.3.4.6 Phosphorus-doped Graphene | 319 | ||
10.3.4.7 Sulfur-doped Graphene | 320 | ||
10.3.4.8 Halogen (F, Cl, Br and I)-doped Graphene | 320 | ||
References | 320 | ||
Subject Index | 326 |