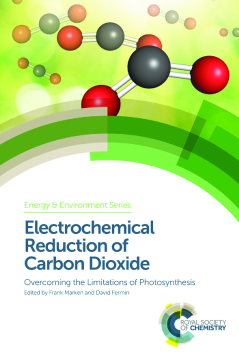
Additional Information
Book Details
Abstract
One of the crucial challenges in the energy sector is the efficient capture and utilisation of CO2 generated from fossil fuels. Carbon capture and storage technologies can provide viable alternatives for energy intensive processes, although implementation of large-scale demonstrators remains challenging. Therefore, innovative technologies are needed that are capable of processing CO2 emission from a wide range of sources, ideally without additional fossil energy demand (e.g. solar driven or overcoming the limits of photosynthesis). This book covers the most recent developments in the field of electrochemical reduction of CO2, from first-principle mechanistic studies to technological perspectives. An introduction to basic concepts in electrochemistry and electrocatalysis is included to provide a background for newcomers to this field. This book provides a comprehensive overview for researchers and industrial chemists working in environmental science, electrochemistry and chemical engineering.
David Fermin is a Professor in the School of Chemistry at the University of Bristol, UK. He has over 15 years’ experience in dynamic electrochemistry and photoelectrochemistry.
Frank Marken is a Professor in the Department of Chemistry at the University of Bath, UK. His research interests lie in both the fundamental and applied aspects of electrochemistry.
Table of Contents
Section Title | Page | Action | Price |
---|---|---|---|
Cover | Cover | ||
Contents | v | ||
Chapter 1 Introduction to the Eletrochemical and Photo-electrochemical Reduction of CO2 | 1 | ||
1.2 New Catalysts for the (Photo-)Electrochemical Reduction of CO2 | 7 | ||
1.3 Combining Heterogeneous and Homogeneous Approaches for the (Photo-)Electrochemical Reduction of CO2 | 10 | ||
1.4 Summary and Chapter Overview | 10 | ||
References | 12 | ||
Chapter 2 Bio-inspired and Bio-electrochemical Approaches in CO2 Reduction Catalysis | 17 | ||
2.1 CO2 Reduction: The Biological Example | 17 | ||
2.2 Bio-electrocatalysis | 19 | ||
2.2.1 Bio-electrochemical Cells and Product Detection | 19 | ||
2.2.2 Performance Metrics | 20 | ||
2.2.3 Substrate | 20 | ||
2.2.4 NADH Regeneration | 21 | ||
2.3 Enzymatic CO2 Electro-reduction | 22 | ||
2.3.1 Carbon Monoxide Generation | 23 | ||
2.3.2 Formate Generation | 28 | ||
2.3.3 Multiple Enzyme Cascades | 35 | ||
2.3.4 Summary | 37 | ||
2.4 Microbial CO2 Reduction | 38 | ||
2.4.1 Microbes in Nature | 38 | ||
2.4.2 Microbial Bio-electrochemical Systems for CO2 Reduction | 39 | ||
2.4.3 Stability | 41 | ||
2.4.4 Summary | 42 | ||
2.5 Bio-inspired Photo-electrochemical CO2 Reduction | 2 | ||
2.5.1 Photo-electrochemical CO2 Reduction in Nature | 42 | ||
2.5.2 Class 1. Sacrificial Chromophore Regeneration | 44 | ||
2.5.3 Class 2. Bio-inspired: Photo-electrochemical Cells | 48 | ||
2.5.4 Class 3. Bio-inspired: Photovoltaic Coupled to Electrochemical Cell (PV-EC) | 50 | ||
2.5.5 Summary | 51 | ||
2.6 Outlook and Future Perspective | 52 | ||
References | 54 | ||
Chapter 3 Copper Catalysts for the Electrochemical Reduction of Carbon Dioxide | 63 | ||
3.1 Introduction | 63 | ||
3.2 Reactivity of Copper | 64 | ||
3.3 Types of Copper Catalysts | 68 | ||
3.3.1 Cu Nanoparticles | 69 | ||
3.3.2 Oxide-derived Copper | 71 | ||
3.3.3 Copper Composites | 81 | ||
3.4 Summary and Future Work | 82 | ||
Acknowledgements | 85 | ||
References | 85 | ||
Chapter 4 Single-crystal Surfaces as Model Electrocatalysts for CO2 Reduction | 88 | ||
4.1 Introduction and General Principles | 88 | ||
4.2 Role of the Surface Structure on the Reduction of CO2 on Pt-group Single-crystal Electrodes | 89 | ||
4.3 Reduction of CO2 on Ag and Au Single-crystal Electrodes | 94 | ||
4.4 Reactivity and Selectivity of Cu Single-crystal Electrodes in the CO2RR | 95 | ||
4.5 Computational Studies | 101 | ||
4.6 Relevant Considerations and Future Challenges | 105 | ||
4.7 Conclusions | 106 | ||
References | 107 | ||
Chapter 5 Homogeneous M(bpy)(CO)3X and Aromatic N-heterocycle Catalysts for CO2 Reduction | 111 | ||
5.1 Introduction | 111 | ||
5.2 CO2 Reduction with Re and Mn Complexes | 114 | ||
5.2.1 Background | 114 | ||
5.2.2 Re Complex Reaction Pathways | 115 | ||
5.2.3 Mn Complex Pathways | 117 | ||
5.2.4 Subsequent Studies | 122 | ||
5.3 Aromatic N-heterocycle Promoted Processes | 124 | ||
5.3.1 Background | 124 | ||
5.3.2 Theoretical Studies of Homogeneous Mechanisms | 125 | ||
5.3.3 ANH Reactions on Surfaces | 128 | ||
5.4 Conclusions | 130 | ||
Acknowledgements | 131 | ||
References | 131 | ||
Chapter 6 DFT Modelling Tools in CO2 Conversion: Reaction Mechanism Screening and Analysis | 136 | ||
6.1 Introduction | 136 | ||
6.2 Insights into the Electrochemical CO2 Conversion Reaction Mechanisms | 140 | ||
6.3 Thermochemistry and Chemical Kinetics in Electrochemical Reactions | 145 | ||
6.4 In Practice | 149 | ||
Acknowledgements | 157 | ||
References | 157 | ||
Chapter 7 Electrocarboxylation in Ionic Liquids | 160 | ||
7.1 Introduction | 160 | ||
7.2 Electrocarboxylation in Ionic Liquids | 163 | ||
7.2.1 Electrocarboxylation of Organic Halide Compounds in Ionic Liquids | 164 | ||
7.2.2 Electrocarboxylation of Aromatic Ketones in Ionic Liquids | 165 | ||
7.2.3 Electrocarboxylation of Other Substrates in Ionic Liquids | 168 | ||
7.3 The Role of the Proton in Ionic Liquids in Determining the Reaction Pathway Accompanying Electroreduction of Aromatic Ketones under a CO2 Atmosphere | 169 | ||
7.3.1 The Influence of C2–H from Imidazolium | 170 | ||
7.3.2 The Influence of Adventitious Water | 173 | ||
7.4 Conclusions and Prospects | 176 | ||
Abbreviations | 177 | ||
References | 177 | ||
Chapter 8 IR Spectro-electrochemistry and Group-6 α-diimine Catalysts of CO2 Reduction | 182 | ||
8.1 Introduction | 182 | ||
8.1.1 Carbon Dioxide Reduction and Homogeneous Catalysis | 182 | ||
8.2 Electroanalytical Techniques and Electrochemical Mechanisms | 183 | ||
8.2.1 Cyclic Voltammetry | 184 | ||
8.2.2 IR Spectro-electrochemistry | 186 | ||
8.3 Group-6 Carbonyl Complexes Bearing Redox Non-innocent Ligands | 189 | ||
8.3.1 Cathodic Behaviour and CO2 Catalysis Pertaining to 2,20-bipyridine Complexes | 191 | ||
8.3.2 Electronic Structure | 193 | ||
8.3.3 Ligand-based Reactivity of Pyridyl-2-carbaldimine Complexes | 201 | ||
8.4 Summary and Outlook | 207 | ||
References | 207 | ||
Chapter 9 Probing CO2 Reduction Intermediates Employing in situ Spectroscopy and Spectrometry | 212 | ||
9.1 Introduction | 212 | ||
9.2 Differential Electrochemical Mass Spectrometry (DEMS) | 214 | ||
9.3 In Situ Spectroscopies | 223 | ||
9.3.1 In Situ Fourier Transform Infrared Spectroscopy (FTIRS) | 224 | ||
9.3.2 In Situ Raman Spectroscopy | 231 | ||
9.3.3 In Situ UV–vis Spectroscopy | 235 | ||
9.4 Summary | 237 | ||
References | 238 | ||
Chapter 10 Surface-selective and Time-resolved Spectro-electrochemical Studies of CO2 Reduction Mechanisms | 244 | ||
10.1 Introduction to Detecting Short-lived Intermediates at Surfaces and in Solution | 244 | ||
10.2 Surface Enhanced Raman Spectroscopy | 245 | ||
10.3 Surface Enhanced Infrared Absorption Spectroscopy (SEIRAS) | 247 | ||
10.4 Sum-frequency Generation Spectroscopy | 251 | ||
10.5 Pulse Radiolysis and Time-resolved Spectroscopy | 256 | ||
10.6 Outlook and Summary | 259 | ||
References | 259 | ||
Subject Index | 264 |