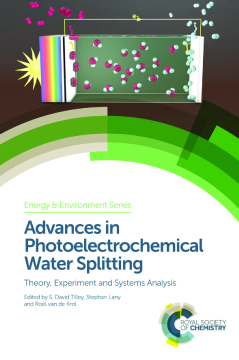
BOOK
Advances in Photoelectrochemical Water Splitting
S David Tilley | Stephan Lany | Roel van de Krol
(2018)
Additional Information
Book Details
Abstract
Tremendous research is taking place to make photoelectrochemical (PEC) water splitting technology a reality. Development of high performance PEC systems requires an understanding of the theory to design novel materials with attractive band gaps and stability. Focusing on theory and systems analysis, Advances in Photoelectrochemical Water Splitting provides an up-to-date review of this exciting research landscape.
The book starts by addressing the challenges of water splitting followed by chapters on the theoretical design of PEC materials and their computational screening. The book then explores advances in identifying reaction intermediates in PEC materials as well as developments in solution processed photoelectrodes, photocatalyst sheets, and bipolar membranes. The last part of the book focuses on systems analysis, which lays out a roadmap of where researchers hope the fundamental research will lead us.
Edited by world experts in the field of solar fuels, the book provides a comprehensive overview of photoelectrochemical water splitting, from theoretical aspects to systems analysis, for the energy research community.
Table of Contents
Section Title | Page | Action | Price |
---|---|---|---|
Cover | Cover | ||
Preface | v | ||
Contents | vii | ||
Chapter 1 The Challenge of Water Splitting in View of Photosynthetic Reality and of Research Trends | 1 | ||
1.1 Introduction | 1 | ||
1.2 The Evolution of Natural Photosynthetic Water Splitting: The Most Remarkable Facts | 4 | ||
1.2.1 The Missing Overpotential in Photosynthesis: What Is the Evidence? | 7 | ||
1.3 How Can Photosynthetic Water Oxidation Be More Efficient Than Technical? | 9 | ||
1.3.1 Thermodynamics of Photo-induced Water Splitting | 10 | ||
1.3.2 How Did Evolution Optimise Photosynthetic Water Oxidation? | 11 | ||
1.3.3 How Could Such a Self-organisation Mechanism Be Experimentally Dealt With? | 13 | ||
1.4 Progress with Artificial Photo-electrochemical Water Splitting | 15 | ||
1.5 Bio-mimetic Approaches Require Progress in Non-equilibrium, Irreversible Thermodynamics | 19 | ||
1.5.1 A Paradigm Change Towards a Fundamental Time Arrow Is Needed | 21 | ||
References | 25 | ||
Chapter 2 Theoretical Design of PEC Materials | 29 | ||
2.1 Introduction | 29 | ||
2.2 Effects of Doping in Photocatalyst | 34 | ||
2.2.1 Chromium Doping in SrTiO3 | 34 | ||
2.2.2 Sulfur and Silicon Doping in Ag3PO4 | 37 | ||
2.3 Band Structure Design of Highly Efficient Photocatalysis by Strain Engineering | 41 | ||
2.3.1 Strain Engineering for Single-layer SnS2 | 41 | ||
2.3.2 Strain Engineering for Layered SnO | 45 | ||
2.4 Exploration of Photofunctional Materials Employing Evolutional Structure Search | 47 | ||
2.4.1 Mixed Valence Tin Oxides as Novel Photocatalysts | 47 | ||
2.4.2 Determination of Crystal Structures of Graphitic Carbon Nitride | 52 | ||
2.5 Conclusions | 57 | ||
Acknowledgements | 58 | ||
References | 58 | ||
Chapter 3 Computational Screening of Light-absorbing Materials for Photoelectrochemical Water Splitting | 62 | ||
3.1 Introduction | 62 | ||
3.2 Density Functional Theory and High-throughput Screening | 65 | ||
3.3 Screening Descriptors and Criteria | 68 | ||
3.3.1 Abundance, Cost and Herfindahl–Hirschman Index | 69 | ||
3.3.2 Toxicity | 70 | ||
3.3.3 Stability | 71 | ||
3.3.4 Electronic Properties | 72 | ||
3.3.5 Direct Calculation of Light Absorption | 76 | ||
3.3.6 Interfaces | 78 | ||
3.4 Materials Investigated | 79 | ||
3.4.1 Perovskites | 81 | ||
3.4.2 Electronic Properties of Existing Materials | 90 | ||
3.4.3 2D Materials | 91 | ||
3.5 Conclusions and Perspectives | 92 | ||
Acknowledgements | 93 | ||
References | 93 | ||
Chapter 4 Unravelling the Charge Transfer Mechanism in Water Splitting Hematite Photoanodes | 100 | ||
4.1 Introduction | 100 | ||
4.2 Photoelectrochemical Methods | 102 | ||
4.2.1 Current Density—Voltage (J–V) Curve Measurements | 102 | ||
4.2.2 Current Transient Measurements | 103 | ||
4.2.3 Cyclic Voltammetry (CV) Surface Measurements | 106 | ||
4.2.4 Electrochemical Impedance Spectroscopy (EIS) | 107 | ||
4.2.5 Intensity Modulated Photocurrent Spectroscopy (IMPS) | 109 | ||
4.3 Mechanism of Water Oxidation | 111 | ||
4.3.1 PEC Water Oxidation on Hematite Photoanode | 111 | ||
4.3.2 Photochemical Water Oxidation on Iron-based Homogeneous Catalysts | 114 | ||
4.3.3 Determination of Water OxidationIntermediates via Operando Infrared Spectroscopy | 115 | ||
4.4 Ternary Metal Oxides for PEC Water Oxidation | 119 | ||
4.4.1 CuWO4 | 119 | ||
4.4.2 BiVO4 | 120 | ||
4.5 Outlook | 121 | ||
4.6 Summary | 122 | ||
Acknowledgements | 122 | ||
References | 122 | ||
Chapter 5 Rate Law Analysis of Water Splitting Photoelectrodes | 128 | ||
5.1 Introduction | 128 | ||
5.1.1 Rate Law Analysis for Solar Fuels Production | 128 | ||
5.1.2 Kinetic Model | 130 | ||
5.1.3 Experimental Set-up | 134 | ||
5.2 Case Studies | 135 | ||
5.2.1 Oxidation Reactions | 136 | ||
5.2.2 Reduction Reactions: Proton Reduction on [Cu2O]/RuOx | 152 | ||
5.3 Conclusions | 159 | ||
Acknowledgements | 159 | ||
References | 159 | ||
Chapter 6 Emerging Semiconductor Oxides for Direct Solar Water Splitting | 163 | ||
6.1 Introduction | 163 | ||
6.2 Bismuth Vanadate | 166 | ||
6.3 Multinary Ferrites | 169 | ||
6.4 Copper-based Oxides | 171 | ||
6.5 Delafossites | 173 | ||
6.6 Strategies for Improving Multinary Oxides | 176 | ||
6.7 Outlook for Multinary Oxides | 177 | ||
References | 178 | ||
Chapter 7 Particulate Photocatalyst Sheets for Efficient and Scalable Water Splitting | 183 | ||
7.1 Introduction | 183 | ||
7.2 Photocatalyst Sheets Based on SrTiO3:La, Rh and BiVO4 | 184 | ||
7.2.1 Preparation and Structure | 184 | ||
7.2.2 Z-Scheme Water Splitting Based on Electron Transfer via an Underlying Conductor | 187 | ||
7.2.3 Comparison with Powder Suspensions and Photoelectrode Systems | 189 | ||
7.2.4 Influence of the Reaction Conditions on the Water Splitting Activity | 190 | ||
7.2.5 Carbon Conductor-based Sheets Operable at Ambient Pressure | 192 | ||
7.2.6 Simulation of Band Diagrams and Carrier Density Distributions | 194 | ||
7.3 Approaches to the Development of Photocatalyst Sheets Based on Narrow Band Gap Photocatalysts | 197 | ||
7.3.1 LaMg1/3Ta2/3O2N as a Hydrogen Evolution Photocatalyst | 197 | ||
7.3.2 Unassisted Photoelectrochemical Water Splitting Using a La5Ti2(Cu,Ag)S5O7 Photocathode and a BaTaO2N Photoanode | 199 | ||
7.4 Summary and Future Prospects | 203 | ||
Acknowledgements | 204 | ||
References | 205 | ||
Chapter 8 Applications of Bipolar Membranes for Electrochemical and Photoelectrochemical Water Splitting | 208 | ||
8.1 Introduction | 208 | ||
8.1.1 Challenges in (Solar Driven) Water Splitting | 209 | ||
8.1.2 Effect of pH | 209 | ||
8.1.3 Membranes in (Solar) Water Splitting | 210 | ||
8.2 Monopolar and Bipolar Ion-exchange Membranes | 210 | ||
8.2.1 Monopolar Membranes | 212 | ||
8.2.2 Bipolar Membranes (BPM) | 217 | ||
8.3 Membrane Performance | 223 | ||
8.3.1 I–V Curves for Monopolar and Bipolar Membranes | 223 | ||
8.3.2 Membrane Pricing | 224 | ||
8.4 Demonstration of BPM's in Water Splitting Systems | 224 | ||
8.4.1 Single Electrolyte | 224 | ||
8.4.2 Extreme pH Gradient Across BPM's | 225 | ||
8.4.3 Intermediate pH Gradient | 227 | ||
8.4.4 Co-ion Transport (at Non-extreme pH) | 227 | ||
8.5 BPM's in Other Electrochemical Systems | 230 | ||
8.5.1 Fuel Cells and Batteries | 230 | ||
8.5.2 CO2 Reduction | 230 | ||
8.6 Outlook/Conclusions | 232 | ||
References | 232 | ||
Chapter 9 Modelling-derived Design Guidelines for Photo-electrochemical Devices | 239 | ||
9.1 Introduction | 239 | ||
9.2 Zero-dimensional Models | 240 | ||
9.2.1 Governing Equations | 240 | ||
9.2.2 Example Results | 241 | ||
9.3 Multi-dimensional Models | 246 | ||
9.3.1 One-dimensional Models | 247 | ||
9.3.2 Two-dimensional Models | 253 | ||
9.4 Conclusion | 260 | ||
Acknowledgements | 261 | ||
References | 261 | ||
Chapter 10 Technoeconomic Analysis of PEC Water Splitting at Various Scales | 266 | ||
10.1 Introduction | 266 | ||
10.2 Basic Considerations and Definitions | 267 | ||
10.2.1 Hydrogen Production and Application Scenarios | 268 | ||
10.2.2 Location | 268 | ||
10.2.3 Specific Collector Size and Number of PEC-PV Units | 268 | ||
10.2.4 Fundamental Economic Constraints under Non-concentrated Sunlight | 270 | ||
10.2.5 Fundamental Economic Constraints under Concentrated Sunlight | 271 | ||
10.3 Plant Design and Components | 273 | ||
10.3.1 Safety | 275 | ||
10.3.2 Cooling and Heating | 275 | ||
10.3.3 Component Sizing | 275 | ||
10.4 Life-cycle Analysis | 276 | ||
10.5 Cost Estimation | 279 | ||
10.6 Benchmarking | 281 | ||
10.7 Conclusions and Outlook | 281 | ||
Acknowledgements | 282 | ||
References | 282 | ||
Subject Index | 285 |