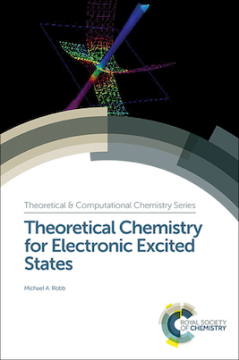
Additional Information
Book Details
Abstract
Over the past few decades, experimental excited state chemistry has moved into the femtochemistry era, where time resolution is short enough to resolve nuclear dynamics. Recently, the time resolution has moved into the attosecond domain, where electronic motion can be resolved as well. Theoretical chemistry is becoming an essential partner in such experimental investigations; not only for the interpretation of the results, but also to suggest new experiments.
This book provides an integrated approach. The three main facets of excited-state theoretical chemistry; namely, mechanism, which focuses on the shape of the potential surface along the reaction path, multi-state electronic structure methods, and non-adiabatic dynamics, have been brought together into one volume. Theoretical Chemistry for Electronic Excited States is aimed at both theorists and experimentalists, involved in theoretical chemistry, in electronic structure computations and in molecular dynamics. The book is intended to provide both with the knowledge and understanding to discover ways to work together more closely through its unified approach.
Table of Contents
Section Title | Page | Action | Price |
---|---|---|---|
Cover | Cover | ||
Preface | v | ||
Contents | xi | ||
Chapter 1 Introduction and Motivation | 1 | ||
1.1 The Chemical Nature of Electronic Excited States | 2 | ||
1.2 Chemical Reactivity in Electronic Excited States | 9 | ||
1.3 The Main Mechanism for Excited State Photochemical Transformations | 22 | ||
1.4 The Essential Features of Excited State Computational Procedures | 24 | ||
1.4.1 Electronic Structure Computations Within the Algebraic Approximation | 24 | ||
1.4.2 Gradients, Second Derivatives, Molecular Structure and Dynamics | 27 | ||
1.4.3 Perturbation Theory Within the Algebraic Approximation | 28 | ||
References | 30 | ||
Chapter 2 Conceptual Development Centred on the Shapes and Topological Features of Potential Surfaces | 34 | ||
2.1 Excited States Are VB Isomers of Ground States | 35 | ||
2.2 The Mechanism of Radiationless Decay | 40 | ||
2.3 Theory of Conical Intersections | 44 | ||
2.3.1 The ''Shape'' of Conical Intersections | 44 | ||
2.3.2 Understanding Conical Intersections Using Valence Bond Theory | 56 | ||
2.3.3 What Happens When One Does a Conical Intersection Circuit in the Branching Plane? | 61 | ||
2.3.4 Conical Intersections in n – 1 Directions: For Example Singlet–Triplet Crossings | 71 | ||
2.3.5 More Advanced Treatment of the Extended Seam of a Conical Intersection | 78 | ||
2.4 Summary | 84 | ||
References | 86 | ||
Chapter 3 Electronic Structure Methods for the Computation of Electronic States | 90 | ||
3.1 How Is an Electronic Excited State FormulatedWithin the Orbital-based Methods Used in the Ground State? | 91 | ||
3.2 The Conceptual Aspects of Electron Correlation for Electronic Excited States | 92 | ||
3.2.1 Multi-dimensional Perturbation Theory | 93 | ||
3.2.2 Three Different Correlation Effects in Excited States | 102 | ||
3.2.3 Effective Hamiltonians for Singly IonizedStates and for Single Excitations from a Closed Shell | 103 | ||
3.2.4 Combining Force Field Methods with Electronic Structure Computations | 106 | ||
3.3 Electronic Structure Methods for Excited State Computation | 110 | ||
3.3.1 Methods with max nh = 1, max np = 1: Complete Active Space SCF Method | 110 | ||
3.3.2 Methods with (max nh = 2, max np = 2): CASPT2 and RPA/TD-DFT | 117 | ||
3.3.3 Methods Based on Space of Particle Hole Excitations | 118 | ||
3.3.4 Nuclear Gradients and Hessians | 119 | ||
3.3.5 Designing an Active Space | 121 | ||
3.4 Non-stationary States and Electron Dynamics:Solving the Time-dependent SchrodingerEquation for Electronic Motion (Electron Dynamics) | 127 | ||
3.5 Summary and Conclusions | 131 | ||
References | 132 | ||
Chapter 4 The Dynamics of Nuclear Motion | 134 | ||
4.1 Theoretical and Conceptual Introduction | 134 | ||
4.2 Quantum Dynamics with Moving Gaussians | 139 | ||
4.3 Electron Dynamics Coupled to Nuclear Motion (the Ehrenfest Method and Beyond) | 145 | ||
4.4 Semi-classical Dynamics with Surface Hopping | 148 | ||
4.5 Summary | 151 | ||
References | 151 | ||
Chapter 5 Applications and Case Studies in Nonadiabatic Chemistry | 153 | ||
5.1 Introductory Remarks | 153 | ||
5.2 Photochromism, Photostabilizers and Photochemical Switches | 157 | ||
5.2.1 Ultrafast Internal Conversion of Azulene | 157 | ||
5.2.2 Dihydroazulene (DHA)/Vinylheptafulvene (VHF) Photochromism | 159 | ||
5.2.3 Diarylethene Photochromism | 162 | ||
5.2.4 Excited State Intramolecular Proton Transfer in o-hydroxyphenyl-(1,3,5)-triazine | 167 | ||
5.2.5 Photostability of an Excited Cytosine–Guanine Base Pair in DNA | 170 | ||
5.3 Cis–Trans Isomerization | 174 | ||
5.3.1 Photo-activation of the Photoactive Yellow Protein | 174 | ||
5.4 Vibrational Control of Photochemistry on an Extended Seam | 179 | ||
5.4.1 Fulvene Dynamics on an Extended Seam | 180 | ||
5.4.2 A Model Cyanine Dye | 183 | ||
5.4.3 The Extended Seam Benzene Conical Intersection | 187 | ||
5.5 Photochemistry Involving Lone Pairs (n–π* States) | 189 | ||
5.5.1 Photochemistry of Formaldehyde | 190 | ||
5.6 Energy Transfer (Charge Transfer vs. Charge Migration) | 194 | ||
5.6.1 Charge Transfer in Bis(hydrazine) RadicalCations and in Bis(methylene) Adamantyl Radical Cation (BMA) | 194 | ||
5.6.2 Electron Dynamics (Charge Migration) in BMA[5,5] | 201 | ||
5.7 Mapping the ''Complete'' Conical Intersection Seams in Benzene | 203 | ||
5.8 Summary | 208 | ||
References | 209 | ||
Chapter 6 Conclusion and Future Developments | 215 | ||
References | 220 | ||
Subject Index | 221 |