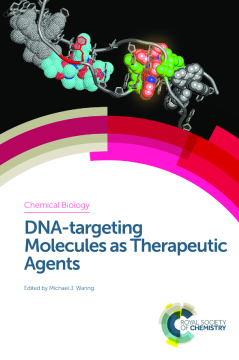
Additional Information
Book Details
Abstract
There have been remarkable advances towards discovering agents that exhibit selectivity and sequence-specificity for DNA, as well as understanding the interactions that underlie its propensity to bind molecules. This progress has important applications in many areas of biotechnology and medicine, notably in cancer treatment as well as in future gene targeting therapies.
The editor and contributing authors are leaders in their fields and provide useful perspectives from diverse and interdisciplinary backgrounds on the current status of this broad area. The role played by chemistry is a unifying theme. Early chapters cover methodologies to evaluate DNA-interactive agents and then the book provides examples of DNA-interactive molecules and technologies in development as therapeutic agents. DNA-binding metal complexes, peptide and polyamide–DNA interactions, and gene targeting tools are some of the most compelling topics treated in depth.
This book will be a valuable resource for postgraduate students and researchers in chemical biology, biochemistry, structural biology and medicinal fields. It will also be of interest to supramolecular chemists and biophysicists.
Table of Contents
Section Title | Page | Action | Price |
---|---|---|---|
Cover | Cover | ||
Preface to Sequence-specific DNA Binding Agents | v | ||
Preface | vii | ||
Contents | ix | ||
Chapter 1 DNA Recognition by Parallel Triplex Formation | 1 | ||
1.1 Why Triplexes? | 1 | ||
1.1.1 Triplets and Triplex Motifs | 2 | ||
1.1.2 Base, Sugar and/or Phosphate Modifications | 3 | ||
1.2 Stabilising Triplexes | 5 | ||
1.2.1 Enhancing Stacking and Hydrophobic Interactions | 5 | ||
1.2.2 Locking the Sugar Pucker | 6 | ||
1.2.3 Adding Positive Charge(s) | 8 | ||
1.2.4 Removing Negative Charge(s) | 11 | ||
1.2.5 Triplex-binding and Cross-linking Agents | 12 | ||
1.3 Decreasing pH Dependence | 13 | ||
1.3.1 Pyrimidine Analogues | 13 | ||
1.3.2 Purine Analogues | 15 | ||
1.4 Recognising Pyrimidine–Purine Base Pairs | 16 | ||
1.4.1 Null Bases and Abasic Linkers | 17 | ||
1.4.2 Natural Bases | 17 | ||
1.4.3 Analogues for CG Recognition | 18 | ||
1.4.4 Analogues for TA Recognition | 21 | ||
1.4.5 Other Approaches | 23 | ||
1.5 Towards Mixed Sequence Recognition at Neutral pH | 23 | ||
1.6 Outlook | 24 | ||
Acknowledgements | 25 | ||
References | 25 | ||
Chapter 2 Interfacial Inhibitors | 33 | ||
2.1 Introduction | 33 | ||
2.2 Case Studies | 34 | ||
2.2.1 Topoisomerase Inhibitors | 34 | ||
2.2.2 HIV Integrase Strand Transfer Inhibitors | 39 | ||
2.2.3 STING Inhibitors | 39 | ||
2.2.4 Arp2–3 Inhibitors | 39 | ||
2.3 Prospects | 42 | ||
Acknowledgements | 43 | ||
References | 43 | ||
Chapter 3 Slow DNA Binding | 45 | ||
3.1 Introduction—Kinetics vs. Thermodynamics of DNA Binding | 45 | ||
3.2 Different DNA Binding Modes—Different DNA Binding Kinetics | 48 | ||
3.2.1 External Electrostatic Binding | 48 | ||
3.2.2 Groove Binding | 48 | ||
3.2.3 Intercalation | 52 | ||
3.2.4 Threading Intercalation | 53 | ||
3.3 Common Slow DNA Binders | 54 | ||
3.3.1 Actinomycin D | 55 | ||
3.3.2 Nogalamycin | 57 | ||
3.4 Ruthenium Complexes Exhibiting Slow DNA-binding Kinetics | 59 | ||
3.4.1 Bis-intercalating Ru-dimer [μ-c4(cpdppz)2(phen) 4Ru2]4 | 60 | ||
3.4.2 Semirigid Ru-dimer [μ-(11,11'-bidppz)(x)4Ru2]4+ (x=phen or bipy) | 64 | ||
3.5 Addendum to Second Edition | 68 | ||
References | 69 | ||
Chapter 4 Thermal Denaturation of Drug–DNA Complexes | 74 | ||
4.1 Introduction | 74 | ||
4.2 Thermal Denaturation Tools | 75 | ||
4.2.1 Analysis of Tm shifts in the Presence of Drug | 75 | ||
4.2.2 Obtaining Binding Enthalpy Values by DSC | 79 | ||
4.2.3 Modeling Melting Curves by McGhee's Algorithm | 80 | ||
4.2.4 Case Studies: Bisintercalating Anthracyclines and Echinomycin | 82 | ||
4.2.5 Summary: Advantages and Pitfalls | 85 | ||
4.3 High-throughput Thermal Denaturation Approaches | 87 | ||
4.3.1 Differential Scanning Fluorimetry | 87 | ||
4.3.2 DSC Compared with DSF: Slow and Expensive but Definitive | 88 | ||
4.3.3 Illustrations of Differential Scanning Fluorimetry Data and Utility | 89 | ||
4.3.4 Advantages and Prospects | 93 | ||
4.4 Summary | 93 | ||
Acknowledgements | 93 | ||
References | 93 | ||
Chapter 5 Computer Simulations of Drug–DNA Interactions: A Personal Journey | 96 | ||
5.1 Introduction | 96 | ||
5.2 Minor Groove DNA Binders | 99 | ||
5.3 Natural Bifunctional Intercalators and Hoogsteen Base Pairing | 103 | ||
5.4 Bis-intercalation of Echinomycin and Related Bifunctional Agents in Relation to Binding Sequence Preferences | 106 | ||
5.5 Binding Preferences of Synthetic Pyridocarbazole Bis-intercalators | 112 | ||
5.6 Sequence Selectivity of Actinomycin D | 113 | ||
5.7 Binding of the Potent Antitumor Agent Trabectedin to DNA | 116 | ||
5.8 Other Examples of DNA Minor-groove-bonding Tetrahydroisoquinoline Antibiotics | 121 | ||
5.9 Melting DNA on the Computer | 123 | ||
5.10 Mitomycin Bis-adduct Formation as a Test Case for QM/MM Methods | 124 | ||
5.11 Lamellarins as Topoisomerase I Poisons | 128 | ||
5.12 Concluding Remarks | 131 | ||
Acknowledgements | 131 | ||
References | 132 | ||
Chapter 6 Binding of Small Molecules to Trinucleotide DNA Repeats Associated with Neurodegenerative Diseases | 144 | ||
6.1 Introduction | 144 | ||
6.1.1 Trinucleotide Repeat DNA | 145 | ||
6.1.2 Diseases Associated with Expansion of Repetitive DNA | 147 | ||
6.1.3 Molecular Mechanism of TNR Expansion | 147 | ||
6.2 Interaction of DNA-binding Drugs with Triplet Repeats Connected with Neurological Diseases | 148 | ||
6.2.1 Actinomycin D | 150 | ||
6.2.2 Aureolic Acid-type Metallo-ligands | 154 | ||
6.2.3 Pyrene-functionalized Pyrrole–Imidazole Polyamides | 156 | ||
6.2.4 Naphthyridine and Its Analogues | 158 | ||
6.2.5 Bulge-binding Agents | 163 | ||
6.2.6 Triptycene- and Acridine-based Ligands | 166 | ||
6.3 Conclusion | 167 | ||
References | 169 | ||
Chapter 7 Parsing the Enthalpy–Entropy Compensation Phenomenon of General DNA–Ligand Interactions by a 'Gradient Determinant' Approach | 175 | ||
7.1 Introduction | 175 | ||
7.1.1 Footprinting Analysis of DNA–Peptide Sequence-selective Interactions | 179 | ||
7.1.2 Circular Dichroism Analysis of DNA–Peptide Interactions | 182 | ||
7.1.3 Investigations of Enthalpy–EntropyCompensation Phenomena in General DNA–Ligand Interactions | 183 | ||
7.2 Conclusions Regarding the EEC Phenomenon of General DNA–Ligand Interactions | 195 | ||
Acknowledgements | 195 | ||
References | 196 | ||
Chapter 8 Structural Studies of DNA-binding Metal Complexes of Therapeutic Importance | 198 | ||
8.1 Introduction–Ruthenium Complexes as DNA Probes and DNA Damage Agents | 198 | ||
8.2 The Versatility of Ruthenium Polypyridyl Complexes | 199 | ||
8.2.1 Early Spectroscopic Studies | 200 | ||
8.3 PACT and PDT | 202 | ||
8.3.1 Therapeutic Relevance | 204 | ||
8.4 Intercalation by Ruthenium–dppz Complexes | 205 | ||
8.4.1 B-DNA Duplexes—Intercalation Geometries and Sequence Specificities | 207 | ||
8.4.2 Lambda Enantiomer | 208 | ||
8.4.3 Semi-intercalation | 210 | ||
8.4.4 Symmetrical Intercalation | 211 | ||
8.4.5 Delta Enantiomer | 212 | ||
8.4.6 Racemic Binding | 214 | ||
8.5 Binding of Ru-polypyridyl Complexes to DNA G-quadruplexes | 216 | ||
8.5.1 Quadruplex Binding—Mononuclear Complexes | 218 | ||
8.5.2 Quadruplex Binding–Binuclear Complexes | 220 | ||
8.6 Summary and Future Outlook | 223 | ||
Acknowledgements | 224 | ||
References | 224 | ||
Chapter 9 Therapeutic Potential of DNA Gene Targeting using Peptide Nucleic Acid (PNA) | 228 | ||
9.1 Introduction | 228 | ||
9.2 Duplex DNA Recognition In Vitro | 229 | ||
9.3 PNA Conjugates | 233 | ||
9.4 Effect of PNA Binding on DNA Structure | 234 | ||
9.5 Cellular Delivery and Tissue Bioavailability In Vivo | 234 | ||
9.6 Cellular Gene Targeting | 235 | ||
9.7 Activation of Gene Transcription | 236 | ||
9.8 Gene-targeted Repair | 236 | ||
9.9 In Vivo Gene Targeting and Repair by PNA Oligomers | 237 | ||
9.10 Therapeutic Prospects | 238 | ||
References | 239 | ||
Chapter 10 Sequence-selective Interactions of Actinomycin D with DNA: Discovery of a Thermodynamic Switch | 246 | ||
10.1 Summary | 246 | ||
10.2 Introduction | 247 | ||
10.3 DNA Sequence Dictates Binding Energetics | 250 | ||
10.3.1 The Energetic Mechanism Is Sequence-dependent | 250 | ||
10.3.2 The Mode of Binding is Intercalation | 250 | ||
10.4 DNA Sequence Effects on Kinetics | 252 | ||
10.4.1 Dissociation Kinetics Properties | 252 | ||
10.4.2 Association Kinetics Controlled by DNA Sequence | 255 | ||
10.4.3 Linkage of Energetics and Kinetics to the Shuffling Model | 255 | ||
10.5 Discussion | 257 | ||
10.6 Summary | 260 | ||
References | 261 | ||
Chapter 11 Molecular Modelling Approaches for Assessing Quadruplex–Small Molecule Interactions | 265 | ||
11.1 Introduction | 265 | ||
11.1.1 A Brief Overview of Quadruplexes | 266 | ||
11.2 G-quadruplex Stabilising Ligands | 269 | ||
11.3 Some Basic Molecular Modelling Approaches | 272 | ||
11.3.1 Molecular Docking Procedures | 272 | ||
11.3.2 Classical Molecular Dynamics Simulations | 273 | ||
11.4 Force Fields for Quadruplexes | 273 | ||
11.4.1 Long-range Electrostatic Interactions | 274 | ||
11.4.2 Base Stacking and Backbone Descriptions | 275 | ||
11.4.3 Molecular Docking and DynamicSimulations of DNA and RNA Quadruplex–Ligand Complexes—Some Examples | 275 | ||
11.5 Enhanced Sampling Methods | 279 | ||
11.5.1 Simulated Annealing Algorithms | 279 | ||
11.5.2 Principal Component Analysis | 280 | ||
11.5.3 Free-energy Calculations | 281 | ||
11.5.4 Umbrella Sampling | 282 | ||
11.5.5 Markov State Models | 283 | ||
11.6 Conclusions | 284 | ||
Acknowledgements | 285 | ||
References | 285 | ||
Chapter 12 Molecular Recognition of DNA by Py–Im Polyamides: From Discovery to Oncology | 298 | ||
12.1 Introduction—DNA-targeted Therapeutics | 298 | ||
12.2 Pairing Rules in the Minor Groove | 299 | ||
12.3 The Hairpin Structure | 300 | ||
12.4 Binding Site Size: β–β, Im–β and Py–β Pairs | 301 | ||
12.5 The γ-Hairpin Turn and Orientation Preference | 303 | ||
12.6 The C-terminus of the Hairpin | 303 | ||
12.7 Second Generation Heterocycles for DNA Recognition | 305 | ||
12.8 Synthetic Methods | 306 | ||
12.9 Disruption of Transcription Factor–DNA Interface | 307 | ||
12.10 Inhibition of RNA Polymerase II Elongation | 307 | ||
12.11 Cell Permeation and Nuclear Localisation | 312 | ||
12.12 Gene Regulation in Cell Culture | 313 | ||
12.13 Global Sequence Analysis of Sequence Specificity | 315 | ||
12.14 Animal Studies: Pharmacokinetics and Toxicity | 318 | ||
12.15 Xenograft Cancer Models | 321 | ||
12.16 Formulation | 324 | ||
Acknowledgements | 325 | ||
References | 325 | ||
Chapter 13 Synthetic Peptides for DNA Recognition Inspired by Transcription Factors | 332 | ||
13.1 Transcription Factors as Source of Inspiration for the Design of DNA-binding Peptides | 332 | ||
13.1.1 Interaction Between dsDNA and Proteins: A Key Factor Regulating Transcription | 332 | ||
13.1.2 Families of Transcription Factors | 334 | ||
13.1.3 Detailed Analysis of the Primary and Secondary Structures of the GCN4 TF Bound to Its Target DNA as a Basis for the Design of Synthetic DNA-binding Mimics | 337 | ||
13.2 Design and Synthesis of TF Mimics as DNA Binding Peptides | 341 | ||
13.2.1 Design of TF Mimics: Replacement of the Dimerization Domain by Non-peptide Scaffolds | 341 | ||
13.2.2 Moving Away From Dimer-based Major Groove Binding: Miscellaneous Peptide Conjugates for Combined Major and Minor Groove Recognition | 351 | ||
13.2.3 Further Structure Minimisation: Monomeric Stapled Peptides as GCN4 TF Mimics | 354 | ||
13.2.4 Increasing the Therapeutic Potential: Cell-uptake Studies and Enhanced Proteolytic Stability | 358 | ||
13.3 Conclusions and Considerations for Future Design | 359 | ||
Acknowledgements | 360 | ||
References | 360 | ||
Chapter 14 Targeting DNA Mismatches with Coordination Complexes | 367 | ||
14.1 Introduction—Transition Metal Complexes as Non-covalent Probes for Nucleic Acids | 367 | ||
14.2 Rhodium Metalloinsertors: Probes for DNA Mismatches | 371 | ||
14.3 Rhodium Metalloinsertors in the Cell | 373 | ||
14.4 Luminescent Ruthenium Complexes as Probes for DNA Mismatches | 379 | ||
14.5 Conclusions and Future Directions | 386 | ||
Acknowledgements | 387 | ||
References | 387 | ||
Chapter 15 CRISPR Highlights and Transition of Cas9 into a Genome Editing Tool | 391 | ||
15.1 Introduction | 391 | ||
15.2 The Discovery of CRISPR | 392 | ||
15.3 CRISPRs Contain Foreign DNA Elements, Suggesting a Role in Immunity | 392 | ||
15.4 Functional Demonstration of CRISPR-dependent Acquired Immunity | 394 | ||
15.5 The Target for CRISPR Interference | 394 | ||
15.6 Cas9, crRNA and tracrRNA: Discovery and Significance | 396 | ||
15.7 Biochemistry of type II CRISPR–Cas-mediated DNA Cleavage | 396 | ||
15.8 First Human Cell Genome Editing Using CRISPR–Cas9 | 397 | ||
15.9 DNA Target Specificity of Cas9 | 399 | ||
15.10 High-fidelity CRISPRs | 400 | ||
15.11 DSB Repair Pathway Recruitment | 402 | ||
15.12 Therapeutics | 402 | ||
References | 404 | ||
Subject Index | 408 |