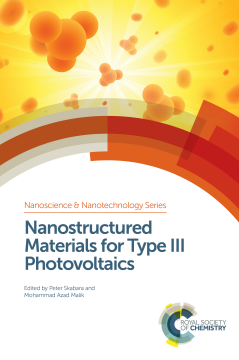
Additional Information
Book Details
Abstract
Materials for type III solar cells have branched into a series of generic groups. These include organic ‘small molecule’ and polymer conjugated structures, fullerenes, quantum dots, copper indium gallium selenide nanocrystal films, dyes/TiO2 for Grätzel cells, hybrid organic/inorganic composites and perovskites. Whilst the power conversion efficiencies of organic solar cells are modest compared to other type III photovoltaic materials, plastic semiconductors provide a cheap route to manufacture through solution processing and offer flexible devices. However, other types of materials are proving to be compatible with this type of processing whilst providing higher device efficiencies. As a result, the field is experiencing healthy competition between technologies that is pushing progress at a fast rate. In particular, perovskite solar cells have emerged very recently as a highly disruptive technology with power conversion efficiencies now over 20%. Perovskite cells, however, still have to address stability and environmental issues. With such a diverse range of materials, it is timely to capture the different technologies into a single volume of work. This book will give a collective insight into the different roles that nanostructured materials play in type III solar cells. This will be an essential text for those working with any of the devices highlighted above, providing a fundamental understanding and appreciation of the potential and challenges associated with each of these technologies.
Table of Contents
Section Title | Page | Action | Price |
---|---|---|---|
Cover | Cover | ||
Nanostructured Materials for Type III Photovoltaics | i | ||
Preface | vii | ||
Dedication | ix | ||
Contents | xi | ||
Chapter 1 - Reliably Measuring the Performance of Emerging Photovoltaic Solar Cells | 1 | ||
1.1 Introduction | 1 | ||
1.1.1 Lamp Spectrum | 5 | ||
1.1.2 Spectral Quality | 6 | ||
1.1.3 Spatial Uniformity | 8 | ||
1.1.4 Temporal Uniformity | 9 | ||
1.2 Reference Diodes and Mismatch Factors | 10 | ||
1.3 Area Measurements | 14 | ||
1.4 External Quantum Efficiency | 19 | ||
1.5 Making Measurements | 20 | ||
1.6 Example of Two Emerging Photovoltaic Systems | 23 | ||
1.7 Summary | 30 | ||
Acknowledgements | 31 | ||
References | 31 | ||
Chapter 2 - Bulk Heterojunction Organic Solar Cells: Working Principles and Power Conversion Efficiencies | 33 | ||
2.1 Introduction | 33 | ||
2.2 History of Organic Photovoltaic Cells | 35 | ||
2.3 Working Principle and Device Design | 39 | ||
2.3.1 Importance of the Absorber Morphology | 41 | ||
2.3.1.1 Post-treatment Exposure56 | 42 | ||
2.3.1.2 Solvents and Solvent Mixtures | 42 | ||
2.3.1.3 Processing Additives | 43 | ||
2.3.1.4 Chemical Design58 | 43 | ||
2.3.1.5 Planar Heterostructures or Graded Junction Solar Cells | 43 | ||
2.3.2 Materials for OPV Cells | 44 | ||
2.4 Efficiency of OSCs | 46 | ||
2.4.1 CTCs and Detailed Balance Limit for OPV Cells | 49 | ||
2.4.2 Empirical Approaches | 52 | ||
2.4.3 Improving Single-junction Devices | 55 | ||
2.4.4 Third-generation Photovoltaics and OSCs | 57 | ||
2.5 Summary: Promising Approaches for Higher Efficiencies | 60 | ||
References | 61 | ||
Chapter 3 - High-performance Organic Photovoltaic Donor Polymers | 69 | ||
3.1 Introduction | 69 | ||
3.1.1 Photophysics in OPV Devices | 70 | ||
3.1.1.1 Organic Semiconductors and Light Absorption Properties | 70 | ||
3.1.1.2 Frenkel Excitons and the Photovoltaic Process | 71 | ||
3.1.1.3 Bulk Heterojunctions and Nanoscale Morphology | 72 | ||
3.1.2 Device Architectures | 73 | ||
3.1.3 Organic Photovoltaic Semiconductors | 74 | ||
3.1.3.1 Donor Materials | 75 | ||
3.1.3.2 Acceptor Materials | 76 | ||
3.2 Design Considerations for Donor Polymer Materials | 77 | ||
3.3 High-efficiency Donor Polymers | 79 | ||
3.3.1 Introduction to Push–Pull Copolymers | 79 | ||
3.3.2 Push–Pull Copolymers Based on Thiophenes, Fused Thiophenes and Their Derivatives | 80 | ||
3.3.3 Push–Pull Copolymers Based on Cyclopentadithiophene, Dithienosilole, Dithienogermole and Other Bridged Bithiophene Derivati... | 85 | ||
3.3.4 Push–Pull Copolymers Based on Benzodithiophene and Its Derivatives | 88 | ||
3.3.5 Push–Pull Copolymers Based on Fluorene, Silafluorene, Carbazole and Other Bridged Biphenyl Derivatives | 96 | ||
3.3.6 Push–Pull Copolymers Based on Indacenodithiophene and Its Derivatives | 98 | ||
3.4 Conclusions and Outlook | 101 | ||
References | 102 | ||
Chapter 4 - p-Type Molecular Materials for Organic Solar Cells | 109 | ||
4.1 Introduction | 109 | ||
4.1.1 Operating Principles | 110 | ||
4.1.2 Device Architecture | 112 | ||
4.2 Designing OPV Materials | 112 | ||
4.2.1 Bandgap Engineering | 113 | ||
4.2.2 Power Conversion Efficiency | 117 | ||
4.2.3 Morphology | 120 | ||
4.3 Thiophene-based Materials | 120 | ||
4.4 Diketopyrrolopyrrole-based Materials | 125 | ||
4.5 Oligoacene-based Materials | 130 | ||
4.6 Triphenyl Amine-based Materials | 133 | ||
4.7 Dyes and Other Miscellaneous Materials | 135 | ||
4.7.1 Squaraine Dye Materials | 135 | ||
4.7.2 BODIPY-based Materials | 139 | ||
4.7.3 Miscellaneous Materials | 142 | ||
4.8 Future Prospects and Outlook | 145 | ||
References | 147 | ||
Chapter 5 - Fullerenes and New Acceptors for Organic Solar Cells | 154 | ||
5.1 Introduction | 154 | ||
5.2 Fullerenes and Derivatives | 158 | ||
5.2.1 C60 and C70 | 159 | ||
5.2.2 Aryl-fullerene-butyric Acid Methyl Ester | 160 | ||
5.2.2.1 PC61BM, PC71BM and PC85BM | 160 | ||
5.2.2.2 PC61BM and PC71BM Multi-adduct | 161 | ||
5.2.2.3 Aryl-substituted PCBM | 161 | ||
5.2.3 Indene-fullerene Adduct | 161 | ||
5.2.4 Other Fullerene Derivatives | 162 | ||
5.3 New Acceptors | 163 | ||
5.3.1 Subphthalocyanine Derivatives | 163 | ||
5.3.2 Amide/imide-functionalized Materials | 164 | ||
5.3.2.1 Perylene Diimide and Related Acceptors | 164 | ||
5.3.2.1.1\rPDI Polymers.Figure 5.4 and Table 5.4 illustrate the chemical structures and performance data, respectively, of PDI polymer acce... | 164 | ||
5.3.2.1.2\rPDI and Related Small Molecules.PDI small molecules are the earliest and one of the most common non-fullerene acceptors used in ... | 167 | ||
5.3.2.2 Naphthalene Diimide Acceptors | 170 | ||
5.3.2.3 Other Amide/imide-functionalized Acceptors | 172 | ||
5.3.3 Fused-ring Electron Acceptors | 174 | ||
5.4 Summary and Outlook | 176 | ||
References | 176 | ||
Chapter 6 - Structure/Property/Processing Relationships for Organic Solar Cells | 182 | ||
6.1 Introduction | 182 | ||
6.1.1 Microscopic Considerations in Organic Photovoltaics | 183 | ||
6.1.2 Macroscopic Considerations in OPV Devices | 186 | ||
6.2 Chemical Design Criteria for Polymers Targeted for OPV Applications | 187 | ||
6.2.1 Manipulation of Optoelectronic Properties Based on Chemical Structure | 187 | ||
6.2.2 Influence of Chemical Structure on Solution Behaviour | 190 | ||
6.2.3 Effects of Solvent(s) | 192 | ||
6.2.4 Controlling Structure Formation in the Solid State Through Chemical Design | 193 | ||
6.2.4.1 Regioregularity and Coupling Defects | 194 | ||
6.2.4.2 Effect of Molecular Weight | 196 | ||
6.2.4.3 Effect of Glass Transition Temperature and Tendency for Vitrification | 197 | ||
6.2.4.4 Molecular Order and Preferred Orientation (Texture) | 198 | ||
6.2.5 Chemical Design of Fullerene Acceptors to Manipulate Device Performance | 199 | ||
6.3 Physicochemical Tools to Manipulate OPV Blends | 203 | ||
6.3.1 Solubility in Solution, Evaporation Rate and Drying Conditions | 203 | ||
6.3.2 Use of Co-solvents and Solvent Additives | 204 | ||
6.3.3 Surface Energy Effects | 206 | ||
6.3.4 Wetting and Film Formation Phenomena | 207 | ||
6.4 Formation of Solid State Structure in OPV Blends | 208 | ||
6.4.1 Solid State Miscibility and the Presence of Mixed Phases | 209 | ||
6.4.2 Use of Phase Diagrams | 211 | ||
6.4.3 Thermal Stability of OPV Blends | 214 | ||
6.5 Conclusions | 216 | ||
References | 217 | ||
Chapter 7 - Charge Generation and Recombination in Organic Solar Cells | 226 | ||
7.1 Introduction | 226 | ||
7.2 From Light Absorption to Exciton Dissociation | 228 | ||
7.2.1 Light Absorption and Exciton Delocalisation | 230 | ||
7.2.2 Role of Phase Morphology | 232 | ||
7.2.3 Hole Transfer | 235 | ||
7.3 From Bound to Separate Charges | 236 | ||
7.3.1 Evidence for Ultrafast Long-range Charge Separation | 236 | ||
7.3.2 Interfacial Charge Transfer States | 238 | ||
7.3.3 Involvement of Hot States | 241 | ||
7.3.4 Impact of Phase Morphology and Interface Structure | 244 | ||
7.3.5 Effect of Transport Properties on Electron–Hole Separation | 249 | ||
7.4 Non-geminate Recombination of Separate Charges | 254 | ||
7.4.1 Bimolecular Langevin Recombination | 255 | ||
7.4.2 Recombination via Triplet States | 257 | ||
7.4.3 Trap-based Recombination | 257 | ||
7.4.4 Auger and Higher Order Recombination | 258 | ||
7.5 Conclusions | 259 | ||
Acknowledgements | 259 | ||
References | 260 | ||
Chapter 8 - Dye-sensitised Solar Cells | 268 | ||
8.1 Introduction | 268 | ||
8.2 Structure and Mechanism of DSSCs | 269 | ||
8.3 Substrates for DSSCs | 271 | ||
8.4 Nanocrystalline Semiconductors | 272 | ||
8.5 Electrolytes | 273 | ||
8.5.1 Liquid Electrolytes | 273 | ||
8.5.2 Ionic Liquid and Quasi-solid State Electrolytes | 273 | ||
8.5.3 Solid Electrolytes | 274 | ||
8.6 Sensitising Dyes | 277 | ||
8.6.1 Ruthenium Complexes | 278 | ||
8.6.2 Porphyrin and Phthalocyanine Dyes | 281 | ||
8.6.3 Metal-free Organic Dyes | 281 | ||
8.6.4 Co-sensitisers | 283 | ||
8.7 Counter Electrodes | 284 | ||
8.8 Scaling-up of DSSCs | 286 | ||
8.8.1 Device Architectures of DSSC Modules | 287 | ||
8.8.1.1 Series Connections | 287 | ||
8.8.1.1.1\rMonolithic Designs.In the monolithic configuration (also called the Kay cell, named after the inventor Andrea Kay, who reported ... | 287 | ||
8.8.1.1.2\rZ-Type Designs.The Z-type configuration is a sandwich-type design requiring two TCO substrates. The adjacent cells are isolated ... | 288 | ||
8.8.1.1.3\rW-Type Designs.The W-type configuration is also a sandwich-type design that requires two TCO substrates. The adjacent cells are ... | 289 | ||
8.8.1.2 Parallel Connections | 289 | ||
8.9 Printing/Coating Techniques | 289 | ||
8.10 Manufacturing of DSSC Modules | 290 | ||
8.11 Conclusions | 291 | ||
References | 292 | ||
Chapter 9 - Hybrid Solar Cells | 298 | ||
9.1 Hybrid Solar Cells | 298 | ||
9.1.1 What Is a Hybrid Solar Cell | 298 | ||
9.1.2 DSSCs | 299 | ||
9.1.2.1 Mechanisms of DSSCs | 299 | ||
9.1.2.2 The Beginning of DSSCs | 300 | ||
9.1.3 Materials and Architecture | 301 | ||
9.1.3.1 Inorganic Layer | 302 | ||
9.1.3.2 TiO2 | 302 | ||
9.1.3.3 Mesoporous TiO2 Nanostructures | 303 | ||
9.1.3.4 TiO2 Nanowires | 304 | ||
9.1.3.5 TiO2 Nanotubes | 305 | ||
9.1.3.6 Other TiO2 Nanostructures | 305 | ||
9.1.3.7 ZnO | 306 | ||
9.1.3.8 Mesoporous ZnO | 307 | ||
9.1.3.9 ZnO Nanowires | 307 | ||
9.1.3.10 ZnO Nanotubes | 308 | ||
9.1.3.11 Other ZnO Nanostructures | 308 | ||
9.1.3.12 Other Inorganic Semiconductors | 308 | ||
9.1.3.13 Sensitisers | 309 | ||
9.1.3.14 Ruthenium Polypyridyl | 310 | ||
9.1.3.15 Metal-free Organic Dyes | 310 | ||
9.1.3.16 Porphyrin Dyes | 311 | ||
9.1.3.17 Electrolytes | 312 | ||
9.1.3.18 Liquid Electrolytes | 312 | ||
9.1.3.19 Solid State Electrolytes | 312 | ||
9.2 Organometal Halide Perovskites | 313 | ||
9.2.1 Structure and Properties | 313 | ||
9.2.1.1 Optical Absorption Properties | 315 | ||
9.2.1.2 Bandgap | 317 | ||
9.2.1.3 Charge Carriers in Halide Perovskites | 318 | ||
9.2.1.4 Hysteresis | 319 | ||
9.2.2 History of Organometal Halides | 321 | ||
9.2.2.1 Halide Perovskites in DSSCs | 321 | ||
9.2.2.2 Arrival of Solid State Cells | 322 | ||
9.2.2.3 Planar Perovskites | 323 | ||
9.2.2.4 Current State-of-the-art Mixed Perovskites | 324 | ||
9.2.3 Stability Issues | 324 | ||
9.2.3.1 Oxygen-induced Degradation | 324 | ||
9.2.3.2 Moisture-induced Degradation | 325 | ||
9.2.3.3 Light-induced Degradation | 325 | ||
9.2.3.4 Thermal Decomposition | 325 | ||
9.2.3.5 Chemical Stability | 326 | ||
Compositional Influences on Stability.Pb-based perovskites mainly suffer from stability issues due to their high sensitivity to ... | 326 | ||
Improving Stability.From the proposed mechanisms, it can be seen that degradation processes require moisture, oxygen and light. ... | 326 | ||
9.2.4 Device Architectures | 327 | ||
9.2.4.1 Mesoporous Scaffold Architectures | 327 | ||
9.2.4.2 Mesoporous Modifications | 327 | ||
9.2.4.3 MSSCs | 329 | ||
9.2.4.4 Planar Heterojunctions | 329 | ||
9.2.4.5 Hole Transporting Materials | 330 | ||
9.2.4.6 Electron Transporting Materials | 332 | ||
9.3 Processing Perovskite Films | 333 | ||
9.3.1 Techniques Based on Solution Processing | 333 | ||
9.3.2 Lead Acetate Perovskites | 333 | ||
9.3.3 Vapour Deposition Techniques | 334 | ||
9.4 Conclusions | 335 | ||
References | 335 | ||
Chapter 10 - Deposition Techniques for Perovskite Solar Cells | 341 | ||
10.1 Introduction | 341 | ||
10.2 Perovskite Solar Cells | 342 | ||
10.3 Deposition Techniques for Perovskite Absorber Layers | 346 | ||
10.3.1 One-step Deposition Techniques | 347 | ||
10.3.2 Two-step Deposition Techniques | 354 | ||
10.3.3 Three-step Deposition Techniques | 362 | ||
10.4 Conclusions | 362 | ||
References | 363 | ||
Chapter 11 - CIGS and CIS Nanomaterials for Solar Cells | 367 | ||
11.1 Introduction | 367 | ||
11.2 Solar Cell Generations | 369 | ||
11.2.1 First Generation Solar Cells | 369 | ||
11.2.1.1 Monocrystalline Silicon Solar Cells | 369 | ||
11.2.1.2 Polycrystalline Silicon Solar Cells | 370 | ||
11.2.2 Second Generation Solar Cells | 370 | ||
11.2.2.1 Amorphous Silicon Thin Film Solar Cells | 370 | ||
11.2.2.2 CdTe Thin Film Solar Cells | 371 | ||
11.2.2.3 CIGS Solar Cells | 371 | ||
11.2.3 Third Generation Solar Cells | 372 | ||
11.2.3.1 Nanocrystal-based Solar Cells | 372 | ||
11.2.3.2 Polymer-based Solar Cells | 372 | ||
11.2.3.3 Dye-sensitised Solar Cells | 373 | ||
11.2.3.4 Concentrator Photovoltaics | 373 | ||
11.2.4 Perovskite-based Solar Cells | 374 | ||
11.3 Third Generation CIGS Solar Cells | 374 | ||
11.3.1 Construction of CIGS Solar Cells | 374 | ||
11.3.2 Crystal Structure of CIGS Tetragonal Unit Cell | 376 | ||
11.3.3 Efficiency of CIGS Solar Cells | 378 | ||
11.3.4 Advantages of CIGS Solar Cells | 378 | ||
11.3.5 Disadvantages of CIGS Solar Cells | 378 | ||
11.3.6 Methods of Preparation of Nanostructured CIGS Solar Cells | 379 | ||
11.3.7 CIGS Materials | 380 | ||
11.4 Third Generation CIS Solar Cells | 381 | ||
11.5 Conclusions | 387 | ||
References | 387 | ||
Chapter 12 - Copper-based Multinary Materials for Solar Cells | 393 | ||
12.1 Introduction | 393 | ||
12.2 CZTS Nanocrystals and Thin Films | 395 | ||
12.3 Cu2ZnSnSe4 Nanocrystals and Thin Films | 415 | ||
12.4 Cu2ZnSn(SSe)4 Nanocrystals and Thin Films | 418 | ||
12.5 Cu2FeSnS4 Nanocrystals and Thin Films | 422 | ||
12.6 Cu2FeSnSe4 Nanocrystals and Thin Films | 425 | ||
12.7 Conclusions | 427 | ||
Acknowledgements | 428 | ||
References | 428 | ||
Chapter 13 - Quantum Dots for Type III Photovoltaics | 436 | ||
13.1 Introduction | 436 | ||
13.2 Historical Background of Photovoltaics | 437 | ||
13.3 Quantum Dots | 438 | ||
13.4 Classification of Photovoltaics | 441 | ||
13.4.1 Dye-sensitised Solar Cells | 443 | ||
13.4.2 Organic Photovoltaics | 444 | ||
13.4.3 Quantum Dot Solar Cells | 447 | ||
13.4.4 Hybrid Solar Cells | 448 | ||
13.5 Synthesis and Characterisation of Quantum Dots | 448 | ||
13.5.1 Synthesis of Binary Semiconducting Quantum Dots | 451 | ||
13.5.1.1 Group I–VI Quantum Dots | 451 | ||
13.5.1.2 Group II–VI Quantum Dots | 452 | ||
13.5.1.3 Group III–VI Quantum Dots | 453 | ||
13.5.1.4 Group IV–VI Quantum Dots | 454 | ||
13.5.2 Ternary and Quaternary Semiconductor Quantum Dots | 455 | ||
13.6 Quantum Dot Based Solar Cells | 456 | ||
13.6.1 Quantum Dot Solar Cells Within the Shockley–Queisser Limit | 457 | ||
13.6.1.1 Quantum Dot Sensitised Solar Cells | 457 | ||
13.6.1.2 Schottky Junction Solar Cells | 458 | ||
13.6.1.3 Heterojunction Quantum Dot Solar Cells | 459 | ||
13.6.1.4 Bulk Heterojunction and Bulk Nanoheterojunction Quantum Dot Solar Cells | 460 | ||
13.6.1.5 Quantum Junction Quantum Dot Solar Cells | 462 | ||
13.6.1.6 Quantum Funnels | 462 | ||
13.6.2 Quantum Dot Solar Cells Beyond the Shockley–Queisser Limit | 462 | ||
13.6.2.1 Multiple Junction or Tandem Quantum Dot Solar Cells | 463 | ||
13.6.2.2 Hot Carrier and Multi-exciton Generation Solar Cells | 463 | ||
13.7 Summary | 464 | ||
References | 464 | ||
Chapter 14 - Charge Dynamics in Colloidal Quantum Dots: Recombination, Trapping and Multiple Exciton Generation | 472 | ||
14.1 Introduction | 472 | ||
14.2 Synthesis of Colloidal Quantum Dots | 475 | ||
14.2.1 Cores | 475 | ||
14.2.1.1 CdX, Where X = S/Te/Se | 475 | ||
14.2.1.2 PbX, Where X = S/Te/Se | 476 | ||
14.2.1.3 InAs, InP and GaAs | 476 | ||
14.2.1.4 CuInSe2 and CuInS2 | 477 | ||
14.2.2 Shelling Techniques | 477 | ||
14.2.3 Passivation and Ligands | 480 | ||
14.3 Experimental Techniques | 481 | ||
14.3.1 Transient Photoluminescence Spectroscopy | 482 | ||
14.3.2 Ultrafast Transient Absorption Spectroscopy | 483 | ||
14.4 Multiple Exciton Generation and Other Cooling Processes | 485 | ||
14.5 Trapping | 489 | ||
14.5.1 Dangling Bonds and Surface States | 489 | ||
14.5.2 Effects of Trap States on Ultrafast Charge Dynamics: Cooling and Recombination | 490 | ||
14.5.3 Effect of Passivation on Trapping Dynamics | 495 | ||
14.5.4 Auger-mediated Trapping Model | 496 | ||
14.6 Recombination Dynamics | 497 | ||
14.7 Summary | 501 | ||
Acknowledgements | 501 | ||
References | 502 | ||
Subject Index | 508 |