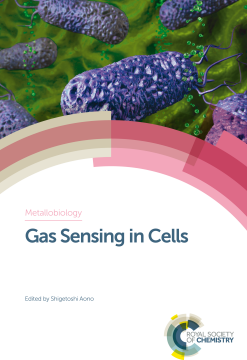
Additional Information
Book Details
Abstract
Gas molecules such as O2, NO, CO and ethylene are present in the environment and are endogenously (enzymatically) produced to act as signalling molecules in biological systems, including the regulation of metabolic networks, chemotaxis, circadian rhythms, mammalian hypoxia responses, and plant ethylene responses by transcriptional, translational, or post translational control. Sensing these gas molecules is the first step in their acting as signalling molecules. When a sensor domain/protein senses an external signal, intra- and inter-molecular signal transductions take place to regulate the biological function of a regulatory domain/protein such as DNA-binding, enzymatic activity, or protein–protein interaction. Interaction between gas molecules and sensor proteins is essential for recognition of gas molecules. Metal-containing prosthetic groups such as haem, iron–sulfur clusters, and non-haem iron centres are widely used. As these metal-containing centres are good spectroscopic probes, detail characterizations have utilized spectroscopic techniques along with X-ray crystallography.
Covering both the signalling and sensing of gaseous molecules, this book provides the first comprehensive overview of gas sensor proteins in both prokaryotic and eukaryotic cells. This book will be particularly interesting to postgraduates and researchers in biochemistry, molecular biology and metallobiology.
Professor Shigatoshi Aono received a Ph.D. from Tokyo Institute of Technology in 1987. After working as a postdoctoral fellow in the University of Georgia, he was an Assistant Professor at the Tokyo Institute of Technology and then an Associate Professor at the Japan Advanced Institute of Science and Technology from 1994. He is now a Professor at the Okazaki Institute for Integrative Bioscience & Institute for Molecular Science, since 2002. His research focuses on the heme-based gas sensor proteins such as CO sensor (CooA) and O2 sensors (HemAT, Aer2, and HemDGC). He has pioneered the establishment of CO as a signaling molecule for bacterial transcriptional regulation.
Dr. Rui Wang has been Vice-President of Research of Laurentian University since January of 2015. From 2004 to 2014, Dr. Wang served as the Vice President of Research firstly, and then as Vice President of Research, Economic Development and Innovation at Lakehead University. Dr. Wang came to Lakehead from the University of Saskatchewan, where he was a Professor of Physiology and leader of both the Cardiovascular Research Group and the Cardiovascular and Respiratory Network. Dr. Wang is an international leader in the study of the metabolism and physiological functions of a group of small molecules of gas, known as gasotransmitters, a category which includes nitric oxide, carbon monoxide, hydrogen sulfide (H2S), and ammonia. Dr. Wang's achievements have been recognized with numerous national and international honors and awards.
Table of Contents
Section Title | Page | Action | Price |
---|---|---|---|
Cover | 1 | ||
Gas Sensing in Cells | 2 | ||
Preface | 6 | ||
Contents | 8 | ||
Chapter 1 - Overview of Gas-sensing Systems | 14 | ||
1.1 Introduction | 14 | ||
1.2 Biological Signal-transduction Systems Including Gas Sensing | 15 | ||
1.2.1 Single-component Systems | 15 | ||
1.2.2 Two-component Systems | 15 | ||
1.2.3 Multicomponent Systems | 19 | ||
1.3 Prosthetic Groups Utilized to Sense Gas Molecules | 20 | ||
1.3.1 Haem | 20 | ||
1.3.2 Iron–Sulfur Clusters | 21 | ||
1.3.3 Nonhaem Iron Centres | 22 | ||
1.3.3.1 Binuclear Nonhaem Iron | 22 | ||
1.3.3.2 Mononuclear Nonhaem Iron | 24 | ||
References | 24 | ||
Chapter 2 - Haem-based Sensors of Nitric Oxide | 28 | ||
2.1 Introduction | 28 | ||
2.2 The Mammalian NO Sensor: Soluble Guanylyl Cyclase (sGC) | 30 | ||
2.3 Bacterial NO-sensing H-NOX Proteins | 32 | ||
2.3.1 Discovery of the H-NOX Family | 32 | ||
2.3.2 Operon Organization of H-NOX Proteins | 33 | ||
2.3.3 Ligand-binding Properties of H-NOX Proteins | 34 | ||
2.3.4 Structural Characterization of H-NOX Proteins | 35 | ||
2.3.5 Hydrogen Bonding Through a Tyrosine Residue in the Distal Pocket Facilitates Ligand Discrimination | 36 | ||
2.3.6 H-NOX Haem Distortion and Its Role in Signal Transduction | 37 | ||
2.3.7 Iron–Histidine Bond Cleavage Upon NO Binding Leads to Haem Relaxation | 39 | ||
2.3.8 Ligand Migration Through the H-NOX Tunnel System | 42 | ||
2.4 The YybT Family of Haem–PAS Domains | 42 | ||
2.4.1 Discovery That YybT is a Haemoprotein Family | 43 | ||
2.4.2 Evidence That YybT is an NO Sensor | 44 | ||
2.5 The E75 Family of Haem-bound Transcription Factors | 45 | ||
2.5.1 Characterization of E75 in Drosophila melanogaster | 46 | ||
2.5.2 Rev-erbs: Mammalian Homologues of E75 | 47 | ||
2.6 Haem and NO Signalling in Regulating Biofilm Formation in Pseudomonas aeruginosa | 48 | ||
2.6.1 NO Regulation of Biofilm Formation in P. aeruginosa | 48 | ||
2.6.2 The Discovery of a Novel Bacterial NO-sensing Protein (NosP) | 49 | ||
2.7 DNR:Transcriptional Regulator of Denitrification | 49 | ||
2.7.1 Protein Structure of Inactive and Active DNR | 50 | ||
2.7.2 Ligand-binding Properties of DNR | 51 | ||
2.7.3 Activation of DNR by NO | 52 | ||
2.8 Conclusions and Perspectives | 52 | ||
References | 54 | ||
Chapter 3 - Haem-based Sensors of Dioxygen | 60 | ||
3.1 Introduction | 60 | ||
3.2 Variations in the Sensor Domain of Haem-based O2-sensor Proteins | 62 | ||
3.2.1 PAS Domain | 62 | ||
3.2.2 GAF Domain | 63 | ||
3.2.3 GCS Domain | 63 | ||
3.3 Two-component Signal Transduction Regulated by O2 Sensing | 64 | ||
3.3.1 FixL | 65 | ||
3.3.2 DevS (DosS) and DosT | 70 | ||
3.3.3 AfGcHK | 72 | ||
3.4 Aerotaxis Control for the Regulation of Bacterial Flagellar Rotation | 74 | ||
3.4.1 HemAT | 75 | ||
3.4.2 Aer2 | 76 | ||
3.5 Synthesis and Hydrolysis of Nucleotide Second Messengers | 79 | ||
3.5.1 YddV (DosC) and EcDOS (DosP) | 81 | ||
3.5.2 HemDGC | 84 | ||
3.5.3 AvGReg and BpeGReg | 86 | ||
3.5.4 AxPDEA1 | 86 | ||
3.5.5 Atypical sGCs:Gyc-88E and GCY-35 | 86 | ||
3.5.6 HemAC-Lm | 89 | ||
3.6 Conclusions | 91 | ||
References | 92 | ||
Chapter 4 - Haem-based Sensors of Carbon Monoxide | 97 | ||
4.1 Introduction | 97 | ||
4.2 Biological Production of CO | 98 | ||
4.2.1 Endogenous CO Production for Ligand of the Metal Clusters in Hydrogenases | 98 | ||
4.2.2 Endogenous CO Production by Haemoxygenases | 100 | ||
4.3 Biological Utilization of CO | 102 | ||
4.3.1 Ni/Fe CO Dehydrogenase | 102 | ||
4.3.2 Mo/Cu CO Dehydrogenase | 104 | ||
4.4 Bacterial CO-sensor Protein CooA | 105 | ||
4.4.1 Structure of CooA | 107 | ||
4.4.2 Allosteric Control of CRP as a Model of CooA | 108 | ||
4.4.3 Allosteric Control of CooA by CO | 112 | ||
4.4.4 Coordination Structures of the Haem in CooA | 115 | ||
4.4.5 Redox Properties of the Haem in CooA | 117 | ||
4.4.6 Spectroscopic Properties of the Haem in CooA | 118 | ||
4.4.7 Ligand Discrimination of CooA | 119 | ||
4.4.8 CO-binding Kinetics of CooA | 120 | ||
4.4.9 DNA Binding and Transcriptional Activation of CooA | 121 | ||
4.5 Bacterial CO-sensor Protein RcoM | 123 | ||
4.5.1 PAS Domain in RcoM | 123 | ||
4.5.2 Spectroscopic Properties of the PAS Domain in RcoM | 124 | ||
4.5.3 Coordination Structure of the Haem in RcoM | 124 | ||
4.5.4 CO-binding Kinetics of RcoM | 126 | ||
4.5.5 LytTR Domain as a DNA-binding Motif | 126 | ||
4.5.6 DNA Binding of LytTR Domain | 127 | ||
4.6 Mammalian CO-sensor Proteins NPAS2 and CLOCK | 128 | ||
4.6.1 Structure of CLOCK/BMAL1 bHLH-PAS Domains | 129 | ||
4.6.2 DNA Binding of bHLH Domain | 129 | ||
4.6.3 Haem as a CO Sensor in the PAS Domains of NPAS2 and CLOCK | 131 | ||
4.6.4 Spectroscopic Properties of the Haem in NPAS2 and CLOCK | 132 | ||
4.7 Mammalian Cystathionine β-synthase (CBS) | 134 | ||
4.7.1 Structural and Spectroscopic Properties of the Haem in CBS | 134 | ||
4.7.2 Ligand Binding Properties of CBS | 136 | ||
4.7.3 Allosteric Control of CBS by CO | 137 | ||
4.8 Concluding Remarks | 138 | ||
Acknowledgements | 139 | ||
References | 139 | ||
Chapter 5 - Iron–Sulfur Cluster-based Sensors | 149 | ||
5.1 Introduction | 149 | ||
5.2 O2-sensing Iron–Sulfur Cluster Proteins | 152 | ||
5.2.1 FNR | 152 | ||
5.2.1.1 The Structure of FNR and the Monomer–Dimer Equilibrium | 152 | ||
5.2.1.2 The Cluster–Conversion Reaction | 155 | ||
5.2.1.3 FNR Cluster Repair | 160 | ||
5.2.1.4 Variation Within the FNR Family | 160 | ||
5.2.1.5 FNR and Pathogenicity | 161 | ||
5.2.2 NreB | 162 | ||
5.2.3 AirS | 163 | ||
5.3 Iron–Sulfur Cluster Proteins that Sense Reduced O2 (Reactive Oxygen Species) | 163 | ||
5.3.1 SoxR | 164 | ||
5.3.2 IscR | 167 | ||
5.3.3 RsrR | 168 | ||
5.4 Iron–Sulfur Cluster Proteins that Sense Nitric Oxide (NO) | 169 | ||
5.4.1 NsrR | 170 | ||
5.4.1.1 A [4Fe–4S] Cluster Containing Rrf2 Superfamily Regulator | 170 | ||
5.4.1.2 Structures of [4Fe–4S] and Apo-NsrR | 172 | ||
5.4.1.3 [4Fe–4S] to [2Fe–2S] Cluster Conversion in NsrR | 172 | ||
5.4.1.4 Reactivity of the [4Fe–4S] Cluster with NO | 173 | ||
5.4.2 WhiB-like (Wbl) [4Fe–4S] Cluster-containing Regulatory Protein Family in Actinobacteria | 176 | ||
5.4.3 FNR and FnrP | 178 | ||
5.4.4 SoxR | 179 | ||
5.4.5 Iron Regulatory Protein 1 (IRP1) | 179 | ||
5.4.6 Corynebacterium glutamicum ArnR | 181 | ||
5.5 Conclusions and Future Perspectives | 181 | ||
Acknowledgements | 182 | ||
References | 182 | ||
Chapter 6 - Nonhaem Iron-based Sensors of Reactive Oxygen and Nitrogen Species | 192 | ||
6.1 Introduction | 192 | ||
6.2 Sensors for Reactive Oxygen Species | 197 | ||
6.2.1 Transcription Factors that Defend Against ROS | 197 | ||
6.2.2 PerR as Peroxide Sensor | 198 | ||
6.2.2.1 Structure of PerR | 198 | ||
6.2.2.2 Regulation Mechanism and Specific Oxidative Modification of PerR | 200 | ||
6.2.3 Redox Sensor SoxR and Reactive Oxygen Species | 205 | ||
6.2.3.1 Structural Characterization of SoxR | 205 | ||
6.2.3.2 Unique Redox-dependent Transcriptional Activity of SoxR | 206 | ||
6.2.4 Regulator Protein Utilizing Nonhaem Iron and ROS | 209 | ||
6.2.4.1 Iron-responsive Regulator (Irr): Active-site Conversion from Haem Iron to Nonhaem Iron | 209 | ||
6.3 Sensor Proteins for Reactive Nitrogen Species | 210 | ||
6.3.1 Reactive Nitrogen Species in Biological System | 210 | ||
6.3.2 Biological Significance of NO: As a Key Molecule for Biological Signal Transduction and Respiratory Denitrification and as ... | 211 | ||
6.3.3 NorR as NO Sensor | 213 | ||
6.3.3.1 Identification of the NO-responding Regulator, NorR | 213 | ||
6.3.3.2 The Nonhaem Iron-binding Site in NorR | 217 | ||
6.3.3.3 NO-responsive Transcriptional Regulation in NorR | 219 | ||
6.3.4 Iron–Sulfur Proteins as NO Sensors | 222 | ||
6.3.4.1 High Sensitivity of the Iron–Sulfur Cluster to RNS and ROS | 222 | ||
6.3.4.2 Widespread NO Sensor in Bacteria: NsrR | 222 | ||
6.3.4.3 A Multistep Reaction Involving up to Eight NO Molecules per Cluster: Wbl Protein | 224 | ||
6.4 Conclusions | 226 | ||
References | 226 | ||
Chapter 7 - Mammalian O2 Sensing and Signalling | 232 | ||
7.1 Cellular O2 Sensing | 232 | ||
7.1.1 HIF Transcriptional Regulator | 233 | ||
7.1.1.1 HIFα | 234 | ||
7.1.1.2 HIF Hydroxylases | 234 | ||
7.1.2 Other O2-sensing Enzymes | 237 | ||
7.1.3 Discovering New Targets for the HIF Hydroxylases | 238 | ||
7.1.3.1 HIF-1α | 238 | ||
7.1.3.2 Ankryin Repeat Domains | 241 | ||
7.1.3.3 Alternate Substrates of PHD | 244 | ||
7.1.4 New Target Identification | 244 | ||
7.1.5 Selected Hypoxia-sensitive Pathways | 250 | ||
7.2 Hydrogen Sulfide and Hypoxia | 252 | ||
7.3 FBXL5 | 254 | ||
7.4 Tissue Signalling | 254 | ||
7.4.1 Acute Hypoxia Sensing by Mammalian Tissue | 254 | ||
7.4.1.1 Neuroepithelial Bodies (NEBs) and Type-1 Glomus Cells | 255 | ||
7.4.1.2 Smooth Muscle Cells (SMCs) | 258 | ||
7.5 Conclusions | 259 | ||
Acknowledgements | 259 | ||
References | 259 | ||
Chapter 8 - Plant Ethylene Sensing and Signalling | 266 | ||
8.1 Introduction | 266 | ||
8.2 Overview of Ethylene Biosynthesis | 267 | ||
8.3 Overview of the Ethylene Signal-transduction Pathway in Plants | 270 | ||
8.4 The Ethylene Receptors | 273 | ||
8.4.1 Ethylene-binding Domain | 275 | ||
8.4.2 Copper and Ethylene Binding | 278 | ||
8.4.3 GAF Domain | 280 | ||
8.4.4 Kinase Domain | 281 | ||
8.4.5 Receiver Domain | 282 | ||
8.4.6 Unique and Nonoverlapping Functions of the Ethylene Receptors in Arabidopsis | 284 | ||
8.4.7 Receptor Clusters | 286 | ||
8.4.8 Other Receptor–Protein Interactions | 287 | ||
8.5 Ethylene Signalling in Nonplants | 290 | ||
8.6 Summary | 293 | ||
Acknowledgements | 293 | ||
References | 293 | ||
Subject Index | 305 |