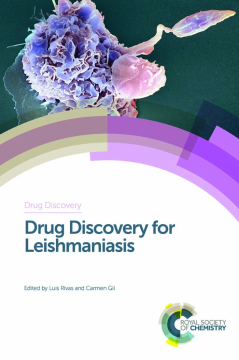
Additional Information
Book Details
Abstract
For human health, leishmaniasis is among the most important protozoan diseases, superseded only by malaria. Globally, 10 to 12 million people are infected with 1.5 million new cases every year. The development of cheaper new drugs is urgently needed for this neglected disease that is developing resistance to current treatments. Chemotherapy remains the only treatment option for the bulk of patients. However, this is largely unaffordable for most. In the past three years numerous advances in drug discovery have been made for treating this disease by exploiting diverging metabolic pathways between the Leishmania enzymes and their hosts, using nanotechnology to target the immune cell phagolysosomes where Leishmania resides.
Drug Discovery for Leishmaniasis aims to provide a perspective of the current treatments and their challenges, blended with the emerging strategies and methodologies that will drive new target appraisals and drug developments, as well as addressing the molecular basis of resistance in Leishmania.
Recent studies have shown that leishmaniasis affects some of the poorest people in the world, with 95% of fatal cases occurring in only 6 countries. With the WHO goal of eliminating this public health problem in the South-east Asia Region by 2020, this book will be important for anyone who is interested in neglected tropical diseases.
This is a timely and highly relevant book that provides a comprehensive and in-depth overview of the current state and future directions of drug discovery for leishmaniasis. It has a broad scientific scope and wonderful insights from leading experts in the field which ensures that there is something for everyone, and that this book is truly a must-have for anyone working in the area of leishmaniasis.
Dr. Steven L. Cobb, Durham University (UK)
Table of Contents
Section Title | Page | Action | Price |
---|---|---|---|
Cover | Cover | ||
Foreword | vii | ||
Contents | ix | ||
I: Appraisal of Leishmaniasis Chemotherapy, Current Status and Pipeline Strategies | 1 | ||
Chapter 1 Leishmaniasis, Impact and Therapeutic Needs | 3 | ||
1.1 The Natural History of Leishmaniasis | 3 | ||
1.1.1 Post-kala-azar Dermal Leishmaniasis (PKDL) | 10 | ||
1.1.2 Leishmania-HIV Co-infection | 10 | ||
1.1.3 Asymptomatic Carriers | 11 | ||
1.1.4 Outbreaks | 11 | ||
1.2 Control Measures | 12 | ||
1.2.1 Diagnostics and Biomarkers | 12 | ||
1.2.2 Disease Control Strategies by Region | 14 | ||
1.3 Existing Treatment Options, Recent Advances and Unmet Needs | 16 | ||
1.3.1 Visceral Leishmaniasis | 16 | ||
1.3.2 Cutaneous Leishmaniasis | 18 | ||
1.3.3 Drug Access | 19 | ||
1.4 Conclusion. Development of an Elimination Tool | 20 | ||
References | 21 | ||
Chapter 2 Anti-leishmanial Drug Discovery: Past, Present and Future Perspectives | 24 | ||
2.1 Introduction | 24 | ||
2.2 Current Drugs for Leishmaniasis | 25 | ||
2.2.1 Lessons Learned from the Properties of Current Drugs for Leishmaniasis | 27 | ||
2.3 Medicinal Chemistry Challenges and Strategies to Discover New Anti-leishmanial Drugs | 27 | ||
2.3.1 Phenotypic Drug Discovery Approaches | 28 | ||
2.4 Future Perspectives | 34 | ||
Acknowledgments | 34 | ||
References | 35 | ||
Chapter 3 From Bench to Bedside: Development and Optimization of Clinical Therapies for Visceral Leishmaniasis | 37 | ||
3.1 Introduction | 37 | ||
3.2 The Role of Preclinical Models in Preclinical to Clinical Translation | 38 | ||
3.2.1 Strategies for in vitro Screening | 38 | ||
3.2.2 Preclinical Animal Models for Visceral Leishmaniasis | 39 | ||
3.2.3 Issues in Interpretation of Preclinical Models | 40 | ||
3.2.4 How can Pre-clinical Data be Translated \r\nto Facilitate Clinical Development? | 41 | ||
3.3 Challenges and Opportunities to Optimize Therapies for Leishmaniasis: The Case of Miltefosine | 42 | ||
3.3.1 Discovery and Development History of Miltefosine for Leishmaniasis | 42 | ||
3.3.2 Changing Patterns in Clinical Efficacy | 43 | ||
3.3.3 Dose Optimization of Miltefosine for Future Combination Strategies | 46 | ||
3.3.4 Translational Approach to Manage Miltefosine’s Main Safety Concern: Teratogenicity | 49 | ||
3.4 Final Remarks | 50 | ||
Acknowledgments | 50 | ||
References | 51 | ||
II: Methodologies and Medicinal Chemistry Strategies to Discover and Develop New Treatments | 55 | ||
Chapter 4 Drug Assay Methodology in Leishmaniasis: From the Microplate to Image Analysis | 57 | ||
4.1 Introduction | 57 | ||
4.2 Drug Assay Methods | 60 | ||
4.3 Models of Infection | 60 | ||
4.3.1 In Vitro | 60 | ||
4.3.2 Ex Vivo | 63 | ||
4.3.3 In Vivo | 63 | ||
4.4 Detection | 65 | ||
4.4.1 Conventional Methods | 66 | ||
4.4.2 Transgenic Leishmania and Related Methods | 68 | ||
4.5 Technological Innovations and Developments | 70 | ||
4.6 Summary | 71 | ||
4.6.1 Optimism for the Future | 72 | ||
Acknowledgments | 72 | ||
References | 73 | ||
Chapter 5 The Pursuit of Novel Anti-leishmanial Agents by High-throughput Screening (HTS) of Chemical Libraries | 77 | ||
5.1 New Chemical Entities (NCEs) are an Unmet Need for Therapeutics of Kinetoplastid Diseases | 77 | ||
5.2 The Challenge of Choosing the Right Screening Tactics and Assays | 79 | ||
5.2.1 The Screening Approach: Target vs. Phenotypic Screening | 80 | ||
5.2.2 The Parasite | 83 | ||
5.2.3 The Host Cell | 84 | ||
5.2.4 The Screening Assay Format | 85 | ||
5.2.5 The Analysis of Screening Data | 85 | ||
5.2.6 Hit Triage: Progression Cascade and Selection Criteria | 87 | ||
5.3 Case Studies of HTS Campaigns for Anti-Leishmanials | 93 | ||
5.4 Conclusions | 97 | ||
References | 97 | ||
Chapter 6 Omics and Their Impact on the Development of Chemotherapy Against Leishmania | 101 | ||
6.1 Introduction | 101 | ||
6.2 Genomics | 103 | ||
6.2.1 Classical Genomic Strategies in Leishmania | 103 | ||
6.2.2 Novel Gain-of-function Genomic Approaches | 104 | ||
6.2.3 Current Limitations and Future Development of Genomic Approaches | 107 | ||
6.3 Transcriptomics | 108 | ||
6.3.1 Transcriptomic Toolkit for Target Elucidation in Leishmania | 108 | ||
6.3.2 Recent Advances in and Perspectives on Transcriptomics for Studying Chemotherapy Against Leishmania | 111 | ||
6.4 Proteomics | 112 | ||
6.4.1 Proteomics Methods | 112 | ||
6.4.2 Proteomics in the Discovery of Modes-of-action and Drug-resistance Mechanisms in Leishmania | 113 | ||
6.4.3 The Future of Proteomics in Drug Discovery | 115 | ||
6.5 Metabolomics | 115 | ||
6.5.1 Metabolomics Methods | 116 | ||
6.5.2 Metabolomics and Anti-leishmanial Drugs | 117 | ||
6.5.3 Resistance and Synergy | 118 | ||
6.5.4 The Future of Metabolomics in Leishmania Drug Development | 119 | ||
6.6 Concluding Remarks | 120 | ||
Acknowledgments | 120 | ||
References | 121 | ||
Chapter 7 In silico Tools for Target Identification and Drug Molecular Docking in Leishmania | 130 | ||
7.1 Introduction | 130 | ||
7.2 Leishmania Targets Identification | 131 | ||
7.3 Anti-leishmanial Drug Development | 133 | ||
7.3.1 Ligand-based Drug Design | 134 | ||
7.3.2 Structure-based Drug Design | 137 | ||
7.3.3 Drug Repurposing | 144 | ||
7.4 Target Databases | 144 | ||
7.5 Concluding Remarks and Outlook | 146 | ||
Acknowledgments | 147 | ||
References | 147 | ||
Chapter 8 Medicinal Chemistry Strategies to Discover New Leishmanicidal Drugs | 153 | ||
8.1 Introduction | 153 | ||
8.2 Functional Scaffolds for Drug Discovery Against Leishmania | 155 | ||
8.2.1 Quinones | 155 | ||
8.2.2 Nitroaromatic Compounds | 157 | ||
8.2.3 Nitrogen Heterocycles | 158 | ||
8.3 Targets for Leishmaniasis Drug Discovery | 161 | ||
8.3.1 Enzymes of Polyamine Biosynthesis | 162 | ||
8.3.2 Enzymes of Thiol Metabolism | 164 | ||
8.3.3 Enzymes of Folate Metabolism | 165 | ||
8.3.4 Sterol Biosynthesis Pathway | 167 | ||
8.3.5 Leishmania Proteases | 168 | ||
8.3.6 Leishmania Kinases | 170 | ||
8.3.7 Leishmania Phosphodiesterases | 171 | ||
8.4 Conclusions | 172 | ||
Acknowledgments | 172 | ||
References | 172 | ||
Chapter 9 Natural Products as a Source of New Drugs Against Leishmania | 179 | ||
9.1 Leishmania-A Challenging Parasite | 179 | ||
9.2 The Interest in Natural Products as Scaffolds: Using a Rational Approach for Drug Discovery | 181 | ||
9.2.1 Major Topics During Biological (In Vitro and In Vivo) Tests of Natural Products | 182 | ||
9.3 From a Crude Extract to a Pure Compound-Aspects of Bioactive Compounds Isolation and Chemical Identification | 184 | ||
9.4 The Anti-leishmanial Potential of Natural Products in Animal Models | 185 | ||
9.4.1 Alkaloids | 185 | ||
9.4.2 Terpenoids | 187 | ||
9.4.3 Saponins | 187 | ||
9.4.4 Phenylpropanoids | 188 | ||
9.4.5 Flavonoids | 189 | ||
9.4.6 Lignoid | 191 | ||
9.4.7 Naphthoquinones | 191 | ||
9.4.8 Iridoid | 192 | ||
9.5 Conclusions | 196 | ||
Acknowledgments | 196 | ||
References | 196 | ||
Chapter 10 Organometallic Compounds in Chemotherapy Against Leishmania | 199 | ||
10.1 Introduction | 199 | ||
10.2 History and Clinical Use of Antimony-based Drugs | 201 | ||
10.3 Organoantimonial Compounds | 203 | ||
10.4 Organobismuth Compounds | 208 | ||
10.5 Organotin(iv) Compounds | 213 | ||
10.6 Organotellurium(iv) Compounds | 215 | ||
10.7 Iron- and Ruthenium-based Organometallic Compounds | 215 | ||
10.8 Palladium, Rhodium and Iridium-based Organometallic Compounds | 218 | ||
10.9 Conclusion | 219 | ||
Acknowledgments | 219 | ||
References | 219 | ||
Chapter 11 New Avenues for Drug Delivery in Leishmania: Using Treatment of Visceral Leishmaniasis with Amphotericin B as an Exemplar | 224 | ||
11.1 Introduction | 224 | ||
11.2 Drug Delivery Systems (DDS) | 225 | ||
11.3 Parenteral Administration | 227 | ||
11.4 Oral Administration | 228 | ||
11.5 Pulmonary Administration | 231 | ||
11.6 Summary | 231 | ||
References | 232 | ||
III: The Quest for Achille's Heel of Leishmania. Singular Targets as New Avenues for Drug Development | 235 | ||
Chapter 12 Addressing the Molecular Biology of Leishmania for Drug Development | 237 | ||
12.1 Introduction | 237 | ||
12.2 The Leishmania Genome | 238 | ||
12.3 The Leishmania Transcriptome | 238 | ||
12.3.1 Polycistronic Transcription and Trans-splicing Mechanisms | 239 | ||
12.3.2 Spliceosome | 241 | ||
12.3.3 Mitochondrial mRNA Editing | 241 | ||
12.4 Post-transcriptional Regulation of Gene Expression | 243 | ||
12.4.1 3' UTR Control and mRNA Degradation | 243 | ||
12.5 Perspectives | 243 | ||
References | 244 | ||
Chapter 13 The Physical Matrix of the Plasma Membrane as a Target: The Charm of Drugs with Low Specificity | 248 | ||
13.1 Outline for an Antimicrobial Peptide-based Chemotherapy against Leishmaniasis | 248 | ||
13.2 General Appraisal of Peptide-based Therapies | 249 | ||
13.3 Natural History of AMPs | 249 | ||
13.4 Mechanism of Action of Antimicrobial Peptides | 250 | ||
13.4.1 Molecular Characteristics of AMPs | 250 | ||
13.4.2 Antimicrobial Peptide-Membrane Interaction | 250 | ||
13.4.3 Models for Antimicrobial Peptide-Membrane Interaction | 251 | ||
13.4.4 The Plasma Membrane of Leishmania as a Target for Antimicrobial Peptides | 252 | ||
13.5 Natural AMPs as Leishmanicidal Agents | 254 | ||
13.6 Assessment of Plasma Membrane Permeabilisation by AMPs in Leishmania | 260 | ||
13.7 Intracellular Targets | 262 | ||
13.8 Induction of Programmed Cell Death of Leishmania by Antimicrobial Peptides | 262 | ||
13.9 Structure-Activity Relationship of Leishmanicidal Antimicrobial Peptides | 263 | ||
13.10 Antimicrobial Peptides in Animal Models of Leishmaniasis | 268 | ||
13.11 Immunomodulation by Leishmanicidal AMPs | 268 | ||
13.12 The Outlook for AMP-based Therapies for Leishmaniasis | 269 | ||
13.12.1 Intrinsic Resistance of Leishmania to Antimicrobial Peptides | 269 | ||
13.12.2 Role of Enviromental Conditions for AMPs on CL Lesions | 269 | ||
13.12.3 Synergism and AMPs for Leishmaniasis | 270 | ||
13.12.4 Antimicrobial Peptide Production. Scale-up Production or In situ Expression | 270 | ||
13.13 Conclusions | 271 | ||
Acknowledgments | 272 | ||
References | 272 | ||
Chapter 14 Nutrient Transport and Sensing as Pharmacological Targets for Leishmaniasis | 282 | ||
14.1 Introduction | 282 | ||
14.2 The Metabolic Environment Inside the Vector and Host | 283 | ||
14.2.1 The Insect Vector | 283 | ||
14.2.2 Development Inside the Host | 284 | ||
14.3 Amino Acid Transport and Homeostasis | 285 | ||
14.3.1 The Amino Acid Pool in Leishmania Cells | 285 | ||
14.3.2 LdAAP24: A New Role for Permease N-termini in Transport Regulation | 286 | ||
14.3.3 A Leishmania-Specific Gene Encodes the Arginine Transporter AAP3 Family | 287 | ||
14.3.4 Lysine Transport | 288 | ||
14.3.5 Transporters of Other Amino Acids | 288 | ||
14.4 Glucose Transport | 288 | ||
14.5 Purine, Pyrimidine and Nucleoside Transport | 290 | ||
14.6 Nutrient Sensing Has a Role in Virulence | 290 | ||
14.6.1 Intracellular Sensing: Response to Purine Starvation | 290 | ||
14.6.2 Extracellular Sensing: Arginine-deprivation Response | 291 | ||
14.6.3 Proteins Involved in Sensing are Localized in the Flagella Membrane | 291 | ||
14.7 Can Nutrient Transporters Deliver Drugs into Leishmania Cells? | 292 | ||
14.7.1 Amino Acid Transporters | 292 | ||
14.7.2 Pentamidine Transport in Leishmania | 292 | ||
14.8 Concluding Remarks | 293 | ||
Acknowledgments | 293 | ||
References | 293 | ||
Chapter 15 Carbon Metabolism as a Drug Target in Leishmania | 297 | ||
15.1 Introduction | 297 | ||
15.2 Carbon Metabolic Network of Leishmania | 300 | ||
15.3 Drug Targets of Carbon Metabolism in Leishmania | 303 | ||
15.4 Functional and Structural Properties of Carbon-metabolic Enzymes that are Potential Drug Targets in Leishmania | 304 | ||
15.5 Drug Discovery | 308 | ||
15.6 Conclusions | 311 | ||
Acknowledgments | 312 | ||
References | 312 | ||
Chapter 16 The Redox Metabolism and Oxidative Stress in Leishmania as a Crossroads for the Lethal Effect of Drugs | 316 | ||
16.1 Reactive Oxygen and Nitrogen Species and Leishmania | 316 | ||
16.1.1 Generation of ROS and RNS within Leishmania | 317 | ||
16.1.2 Exogenous Sources of Oxidative and Nitrosative Stress to Leishmania | 320 | ||
16.2 Elimination of ROS and RNS | 321 | ||
16.2.1 Superoxide Dismutases | 321 | ||
16.2.2 Class I Haem Peroxidases | 322 | ||
16.2.3 The Trypanothione Redox System | 323 | ||
16.3 Conclusions | 335 | ||
Acknowledgments | 336 | ||
References | 336 | ||
Chapter 17 DNA Topoisomerases as Promising Targets for Leishmania Chemotherapy | 348 | ||
17.1 Introduction | 348 | ||
17.2 Supercoiled vs. Relaxed DNA. The Need for DNA Topoisomerases | 350 | ||
17.3 DNA Topoisomerases in Leishmania | 352 | ||
17.3.1 Leishmania infantum DNA Topoisomerase IB | 353 | ||
17.3.2 Inhibitors of DNA Topoisomerase IB | 356 | ||
17.4 Other DNA Topoisomerases in Leishmania | 361 | ||
17.4.1 Type II DNA Topoisomerases | 361 | ||
17.4.2 Type IA DNA Topoisomerases | 362 | ||
17.4.3 Inhibitors of DNA Topoisomerase II | 362 | ||
17.5 Conclusions | 363 | ||
Acknowledgments | 364 | ||
References | 364 | ||
Chapter 18 Molecular Basis of Drug Resistance in Leishmania | 371 | ||
18.1 Emerging Drug Resistance | 371 | ||
18.2 Methods to Study Resistance | 372 | ||
18.3 Molecular Mechanisms of Resistance and Current Limitations | 372 | ||
18.3.1 Antimonials | 373 | ||
18.3.2 Miltefosine | 376 | ||
18.3.3 Paromomycin | 378 | ||
18.3.4 Amphotericin B | 379 | ||
18.4 Conclusions | 379 | ||
Acknowledgments | 379 | ||
References | 380 | ||
Chapter 19 The Macrophage-Parasite Interface as a Chemotherapeutic Target in Leishmaniasis | 387 | ||
19.1 Introduction | 387 | ||
19.2 Phagocytosis and Phagolysosome Biogenesis | 389 | ||
19.3 The Membrane Fusion Machinery and the Phagosome | 389 | ||
19.4 Leishmania and Phagosome Remodelling | 390 | ||
19.5 The Parasitophorous Vacuole as a Potential Target for Drug Development | 391 | ||
19.6 Conclusions | 393 | ||
References | 393 | ||
Subject Index | 396 |