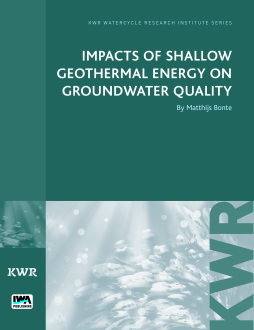
Additional Information
Book Details
Abstract
The use of shallow geothermal energy (SGE) systems to acclimatize buildings has increased exponentially in the Netherlands and worldwide. In certain areas, SGE systems are constructed in aquifers also used for drinking water supply raising the question of potential groundwater quality impact.
Impacts of Shallow Geothermal Energy on Groundwater Quality provides a hydrochemical and geomicrobial overview of the effects of ground source heat pumps and aquifer thermal energy storage. The area is investigated with field and laboratory experiments, and reactive transport models, showing that shallow geothermal energy systems can influence groundwater quality in a number of ways. Most prominent in open ground source heating systems operating at low temperature (
Table of Contents
Section Title | Page | Action | Price |
---|---|---|---|
Cover | Cover | ||
Contents | v | ||
Chapter 1: Introduction | 1 | ||
1.1 BACKGROUND | 1 | ||
1.2 SHALLOW GEOTHERMAL ENERGY, SUBSURFACE USE AND DRINKING WATER PRODUCTION | 3 | ||
1.3 RESEARCH OBJECTIVE AND QUESTIONS | 5 | ||
1.4 METHODOLOGY AND OUTLINE | 6 | ||
Chapter 2: Shallow geothermal energy: A review of impacts on groundwater quality and policy in the Netherlands and\rEuropean Union | 7 | ||
ABSTRACT | 7 | ||
2.1 INTRODUCTION | 7 | ||
2.2 A REVIEW ON THE POTENTIAL IMPACTS OF SGE ON GROUNDWATER QUALITY | 8 | ||
2.2.1 Hydrological impacts | 9 | ||
2.2.2 Thermal impacts | 10 | ||
2.2.3 Chemical impacts in clean groundwater systems | 11 | ||
2.2.4 Chemical impacts in contaminated groundwater systems | 12 | ||
2.2.5 Chemical impacts of BTES systems | 12 | ||
2.2.6 Microbiological Impacts | 13 | ||
2.3 PAST AND CURRENT POLICY FOR SGE | 14 | ||
2.3.1 SGE policy in the Netherlands prior to July 2013 | 14 | ||
2.3.2 SGE policy in the Netherlands after July 2013 | 14 | ||
2.3.3 Policies of European member states on SGE | 16 | ||
2.4 DISCUSSION: SUBSURFACE TECHNOLOGY DEVELOPMENT AND REGULATION | 17 | ||
2.5 CONCLUSIONS | 18 | ||
Chapter 3: A field and modeling study of the impacts of aquifer thermal energy storage on groundwater quality | 21 | ||
ABSTRACT | 21 | ||
3.1 INTRODUCTION | 21 | ||
3.2 SITE DESCRIPTION | 22 | ||
3.3 METHODS | 24 | ||
3.3.1 Field and laboratory methods | 24 | ||
3.3.2 Numerical modeling of the field data | 25 | ||
3.4 RESULTS | 27 | ||
3.4.1 Flow and temperature data | 27 | ||
3.4.2 Ambient chemical groundwater quality | 27 | ||
3.4.3 ATES chemical water quality | 29 | ||
3.4.4 ATES microbiological water quality | 30 | ||
3.4.5 Numerical modeling of field data | 31 | ||
3.5 DISCUSSION | 33 | ||
3.6 CONCLUSIONS | 34 | ||
Chapter 4: Temperature-induced impacts on mobility of arsenic and other trace elements | 37 | ||
ABSTRACT | 37 | ||
4.1 INTRODUCTION | 37 | ||
4.2 MATERIALS AND METHODS | 38 | ||
4.2.1 Sediment collection, characterization, and geochemical analyses | 38 | ||
4.2.2 Influent water collection and characterization | 40 | ||
4.2.3 Experimental setup | 41 | ||
4.2.4 Hydrochemical analyses | 42 | ||
4.2.5 Data analysis | 42 | ||
4.2.6 Arsenic sorption isotherms | 42 | ||
4.2.7 Sorption thermodynamics | 43 | ||
4.3 RESULTS AND DISCUSSION | 44 | ||
4.3.1 General patterns | 44 | ||
4.3.2 Silicate minerals dissolution | 46 | ||
4.3.3 Dissolved organic carbon mobilization | 47 | ||
4.3.4 Mobilization of arsenic and other trace compounds | 48 | ||
4.3.5 Arsenic sorption isotherms | 49 | ||
4.3.6 Temperature influence | 50 | ||
4.3.7 Environmental implications | 52 | ||
4.4 CONCLUSIONS | 52 | ||
Chapter 5: Temperature-induced impacts on redox processes and microbial communities | 55 | ||
ABSTRACT | 55 | ||
5.1 INTRODUCTION | 55 | ||
5.2 MATERIALS AND METHODS | 56 | ||
5.2.1 Sediment and groundwater collection | 56 | ||
5.2.2 Experimental setup | 57 | ||
5.2.3 Chemical and microbiological analyses | 57 | ||
5.2.4 Deriving kinetic and thermodynamic parameters | 57 | ||
5.3 RESULTS | 58 | ||
5.3.1 Increasing residence time (IRT) experiments | 58 | ||
5.3.2 Temperature ramping (TR) experiments | 59 | ||
5.3.3 Microbial community changes | 59 | ||
5.3.4 Kinetics and thermodynamics of sulfate-reduction | 61 | ||
5.4 DISCUSSION | 62 | ||
5.4.1 Impact of temperature on prevailing redox reactions | 62 | ||
5.4.2 Thermophilic redox processes and microbial communities | 63 | ||
5.4.3 Accumulation of dissolved organic carbon (DOC) and organic carbon turnover | 64 | ||
5.4.4 Sulfate-reduction kinetics and thermodynamics | 65 | ||
5.5 ENVIRONMENTAL AND TECHNOLOGICAL IMPLICATIONS | 66 | ||
Chapter 6: Reactive transport modeling of thermal column experiments to investigate the impacts of aquifer thermal energy storage on groundwater quality | 69 | ||
6.1 INTRODUCTION | 69 | ||
6.2 EXPERIMENTAL METHODS | 70 | ||
6.3 MODELING FRAMEWORK | 71 | ||
6.3.1 Modeling framework, boundary, and initial conditions | 71 | ||
6.3.2 Heat boundary conditions and transport | 73 | ||
6.3.3 Temperature correction for mineral equilibria and reaction rates | 74 | ||
6.3.4 Surface complexation modeling | 74 | ||
6.3.5 Cation-exchange | 75 | ||
6.3.6 Kinetic dissolution of K-feldspar | 76 | ||
6.3.7 Mineral interactions in thermodynamic equilibrium | 76 | ||
6.3.8 Automatic model optimization, sensitivity and uncertainty analysis | 77 | ||
6.4 RESULTS AND DISCUSSION | 77 | ||
6.4.1 Surface complexation modeling results | 77 | ||
6.4.2 Contrasting sorption behavior anions versus cations | 78 | ||
6.4.3 Application to a virtual aquifer thermal energy storage system | 79 | ||
6.4.4 Limitations and environmental implications | 81 | ||
6.5 ACKNOWLEDGMENT | 81 | ||
Chapter 7: Summary and synthesis | 83 | ||
7.1 INTRODUCTION | 83 | ||
7.2 SUMMARY OF RESEARCH | 83 | ||
7.2.1 Chapter 2: Shallow geothermal energy: A review of impacts\ron groundwater quality and policy in the Netherlands and\rEuropean Union | 83 | ||
7.2.2 Chapter 3: A field and modeling study of the impacts of aquifer thermal energy storage on groundwater quality | 84 | ||
7.2.3 Chapter 4: Temperature-induced impacts on mobility of arsenic and other trace elements | 84 | ||
7.2.4 Chapter 5: Temperature-induced impacts on redox processes and microbial communities | 84 | ||
7.2.5 hapter 6: Reactive transport modeling of thermal column experiments to investigate the impacts of aquifer thermal energy storage on groundwater quality | 85 | ||
7.3 TRANSLATING HYDROCHEMICAL EFFECTS TO IMPACTS ON DRINKING WATER PRODUCTION | 85 | ||
7.4 GROUNDWATER QUALITY MONITORING NEAR SGE SYSTEMS | 87 | ||
7.5 POLICY PERSPECTIVES | 88 | ||
7.6 RESEARCH PERSPECTIVES | 90 | ||
References | 93 | ||
Appendix 1: Supporting information | 107 | ||
Appendix 2: Supporting information | 115 | ||
S2.1 EXPERIMENTAL DETAILS | 115 | ||
S2.2 METHODS: MICROBIOLOGICAL ANALYSES | 115 | ||
S2.2.1 DNA Extraction | 115 | ||
S2.2.2 T-RFLP fingerprinting of bacterial communities | 117 | ||
S2.2.3 Amplicon preparation and pyrosequencing of bacterial 16S rRNA genes | 118 | ||
S2.2.4 Denaturing gradient gel electrophoresis (DGGE) of archaeal communities | 118 | ||
S2.3 HYDROGEN THRESHOLDS FOR REDOX PROCESSES | 119 | ||
S2.4 MICROBIOLOGICAL RESULTS | 120 | ||
S2.5 KINETIC AND THERMODYNAMIC PARAMETERS OF SULFATE REDUCTION REPORTED IN LITERATURE | 122 | ||
Appendix 3: Supplementary information | 125 | ||
S3.1 METHODS | 125 | ||
S3.1.1 Sediment sampling and characterization | 125 | ||
S3.1.2 Experimental setup | 125 | ||
S3.1.3 Chemical analyses | 126 | ||
S3.2 ANALYSIS OF CONSERVATIVE BREAKTHROUGH TESTS | 127 | ||
S3.3 ADDITIONAL INFORMATION ON SIMULATED CATION EXCHANGE | 128 | ||
S3.4 ADDITIONAL INFORMATION ON THE KINETIC DISSOLUTION OF K-FELDSPAR | 129 | ||
S3.5 ADDITIONAL INFORMATION ON THE METHODOLOGY FOR SENSITIVITY AND UNCERTAINTY ANALYSIS | 130 | ||
S3.6 ADDITIONAL RESULTS | 130 | ||
S3.6.1 Surface complexation | 130 | ||
S3.6.2 K-feldspar dissolution and cation exchange | 131 | ||
S3.7 OTHER INFORMATION | 132 | ||
S3.8 ADDITIONAL SIMULATION RESULTS OF THE AQUIFER THERMAL ENERGY STORAGE SCENARIO CASE STUDIES | 135 |