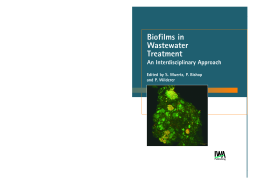
Additional Information
Book Details
Abstract
The central theme of the book is the flow of information from experimental approaches in biofilm research to simulation and modeling of complex wastewater systems. Probably the greatest challenge in wastewater research lies in using the methods and the results obtained in one scientific discipline to design intelligent experiments in other disciplines, and eventually to improve the knowledge base the practitioner needs to run wastewater treatment plants. The purpose of Biofilms in Wastewater Treatment is to provide engineers with the knowledge needed to apply the new insights gained by researchers. The authors provide an authoritative insight into the function of biofilms on a technical and on a lab-scale, cover some of the exciting new basic microbiological and wastewater engineering research involving molecular biology techniques and microscopy, and discuss recent attempts to predict the development of biofilms. This book is divided into 3 sections: Modeling and Simulation; Architecture, Population Structure and Function; and From Fundamentals to Practical Application, which all start with a scientific question. Individual chapters attempt to answer the question and present different angles of looking at problems. In addition there is an extensive glossary to familiarize the non-expert with unfamiliar terminology used by microbiologists and computational scientists.  The colour plate section of this book can be downloaded by clicking here. (PDF Format 1 MB)
Table of Contents
Section Title | Page | Action | Price |
---|---|---|---|
Contents | 4 | ||
Preface | 9 | ||
Contributors | 12 | ||
Modeling and simulation: Introduction | 18 | ||
References | 21 | ||
1.0 What do biofilm models, mechanical ducks and artificial life have in common? | 23 | ||
1.1 MATHEMATICS AND BIOLOGY: HOW DO THEY GO TOGETHER? | 24 | ||
1.2 WHAT ACTUALLY ARE MATHEMATICAL MODELS AND WHAT IS MATHEMATICAL MODELING? | 25 | ||
1.3 HOW MATHEMATICAL MODELS CAN BE USED TO STUDY COMPLICATED BIOFILM ARCHITECTURES | 31 | ||
1.4 BEYOND MATHEMATICAL MODELS: REDUCTIONISM AND HOLISM, PHYSICALISM AND VITALISM AS PHILOSOPHICAL CONCEPTS IN THEORETICAL BI | 35 | ||
1.5 INTERDISCIPLINARITY: WHY IT IS DIFFICULT TO COMMUNICATE WITH MATHEMATICIANS | 40 | ||
1.6 CONCLUSION | 43 | ||
1.7 REFERENCES | 43 | ||
2.0 Biofilm architecture: interplay of models and experiments | 47 | ||
2.1 TOOLS FOR BIOFILM STUDIES | 47 | ||
2.2 EARLY OBSERVATION AND MODELS | 49 | ||
2.3 STANDARD DIFFUSION-REACTION MODEL | 51 | ||
2.3.1 Mathematical formulation | 51 | ||
2.3.2 Standard model validation | 52 | ||
2.4 NEW EXPERIMENTAL TECHNIQUES | 52 | ||
2.5 CHALLENGES IN MODELING | 53 | ||
2.5.1 Characterization of morphology | 53 | ||
2.5.2 Description of microbial communities | 54 | ||
2.6 MODELING OF STRUCTURAL HETEROGENEITY | 56 | ||
2.6.1 Discrete models | 56 | ||
2.7 CONCLUSIONS AND FUTURE DIRECTIONS | 58 | ||
2.8 REFERENCES | 60 | ||
3.0 Towards new mathematical models for biofilms | 64 | ||
3.1 INTRODUCTION | 64 | ||
3.2 STRATEGIES OF MATHEMATICAL MODELING | 65 | ||
3.3 EXAMPLES OF MATHEMATICAL MODELS | 67 | ||
3.3.1 Physical approach | 67 | ||
3.3.2 Introducing randomness | 68 | ||
3.3.3 Models from system theory | 68 | ||
3.3.4 Compound models | 68 | ||
3.4 ESTABLISHED BIOFILM MODELS | 69 | ||
3.5 MODELS FROM MATHEMATICAL BIOLOGY | 70 | ||
3.6 IDEAS FOR STOCHASTIC MODELS | 71 | ||
3.7 CONCLUDING REMARKS | 73 | ||
3.8 REFERENCES | 73 | ||
4.0 Beyond models: requirements and chances of computational biofilms | 75 | ||
4.1\tINTRODUCTION: SIMULATING BIOFILM SYSTEMS | 75 | ||
Mathematical modeling | 78 | ||
Numerical treatment | 78 | ||
Implementation | 78 | ||
Embedding | 78 | ||
Visualization | 79 | ||
Validation | 79 | ||
4.2\tMATHEMATICAL MODELS | 79 | ||
4.3\tNUMERICAL METHODS | 84 | ||
4.3.1\tDiscretization of the domain | 84 | ||
4.3.2\tDiscretization of the equations | 87 | ||
4.3.3\tSolving the discrete equations | 91 | ||
4.4\tIMPLEMENTATION | 93 | ||
4.5\tEMBEDDING | 95 | ||
4.6\tVISUALIZATION: HOT AIR OR CATALYST? | 96 | ||
4.7\tVALIDATION | 98 | ||
4.8\tCONCLUDING REMARKS | 99 | ||
4.9\tREFERENCES | 100 | ||
5.0 On the influence of fluid flow in a packed-bed biofilm reactor | 103 | ||
5.1 Introduction | 103 | ||
5.1.1 Basic questions and considerations | 105 | ||
5.1.2 Conclusions from the \"state-of -the-art | 106 | ||
5.2 SOME COMMENTS ON SPECIFIC TOPICS | 112 | ||
5.2.1 Elephant versus microorganism: the different scales to simulate | 112 | ||
5.2.2 On fields, layers, flow regimes, pressure gradients, and their importance in packed-bed biofilm reactors | 113 | ||
5.3 CURRENT INVESTIGATIONS | 116 | ||
5.3.1 Numerical method | 116 | ||
5.3.2 A comparison between laboratory and pilot reactor or the scale up problem | 118 | ||
5.4 INFLUENCE OF FLUID FLOW AND SUBSTRATE CONCENTRATION ON BIOFILM ARCHITECTURE | 125 | ||
5.5 CONCLUSIONS AND OUTLOOK | 126 | ||
5.6 REFERNCES | 129 | ||
6.0 The effect of biofilm heterogeneity on metabolic processes | 138 | ||
6.1 Introduction | 138 | ||
6.2 BIOFILM PROPERTIES | 139 | ||
6.2.1 Heterogeneity | 139 | ||
6.2.2 Extracellular polymeric substances | 142 | ||
6.2.3 Diffusivity | 143 | ||
6.2.4 Detachment | 149 | ||
6.3 CONFOCAL LASER SCANNING MICROSCOPY | 151 | ||
6.4 CELL-TO-CELL COMMUNICATION | 152 | ||
6.5 MOLECULAR PROBES | 152 | ||
6.6 CONCLUDING REMARKS | 154 | ||
6.7 REFERENCES | 155 | ||
7.0 Mass transport in heterogeneous biofilms | 162 | ||
7.1 INTRODUCTION | 162 | ||
7.2 BIOFILM HETEROGENEITY AND BIOFILM MODELS | 165 | ||
7.3 QUANTIFYING NUTRIENT UPTAKE KINETICS FROM THE NUTRIENT CONCENTRATION PROFILES | 168 | ||
7.4 QUANTIFYING MASS TRANSPORT MECHANISMS FROM FLOW VELOCITY PROFILES IN BIOFILMS | 171 | ||
7.5 LOCAL MASS TRANSPORT RATES IN HETEROGENEOUS BIOFILMS | 174 | ||
7.6 THE CONCEPT OF BIOFILMS COMPOSED OF DISCRETE LAYERS | 177 | ||
7.7 MODELING MASS TRANSPORT AND ACTIVITY IN BIOFILMS COMPOSED OF DISCRETE LAYERS | 181 | ||
7.8 EXPERIMENTAL VALIDATION OF THE MODEL OF BIOFILMS COMPOSED OF DISCRETE LAYERS | 184 | ||
7.9 CAN DISCRETIZING BIOFILMS REFLECT THE EFFECT OF BIOFILM HETEROGENEITY? | 184 | ||
7.10 BIOFILMS GROWN AT HIGH FLOW VELOCITIES | 186 | ||
7.11 CONCLUDING REMARKS | 187 | ||
7.12 REFERENCES | 190 | ||
8.0 The crucial role of extracellular polymeric substances in biofilms | 193 | ||
8.1 INTRODUCTION | 193 | ||
8.2 DEFINITION OF EPS | 194 | ||
8.3 COMPOSITION AND PROPERTIES OF EPS | 197 | ||
8.4 MECHANICAL STABILITY MEDIATED BY EPS | 202 | ||
8.5 ROLE OF EPS IN MICROBIAL AGGREGATION | 203 | ||
8.6 FUNCTIONS OF EPS | 209 | ||
8.7 TECHNICAL ASPECTS OF EPS | 212 | ||
8.7.1 Settleability | 212 | ||
8.7.2 Foam formation | 213 | ||
8.7.3 Dewaterability | 213 | ||
8.7.4 Sorption of organic and inorganic molecules | 214 | ||
8.7.5 Diffusion | 215 | ||
8.7.6 Formation of a gel layer on surfaces | 215 | ||
8.8 ECOLOGICAL ASPECTS | 215 | ||
8.9 OUTLOOK | 217 | ||
8.10 REFERENCES | 218 | ||
9.0 The importance of physicochemical properties in biofilm formation and activity | 226 | ||
9.1 INTRODUCTION | 226 | ||
9.2 HOW ADHESION HAS BEEN PREDICTED | 227 | ||
9.2.1 Thermodynamic approach | 227 | ||
9.2.2 DLVO theory | 229 | ||
9.2.3 XDLVO theory | 232 | ||
9.3 SURFACE PROPERTIES RELEVANT FOR ADHESION | 233 | ||
9.3.1 Hydrophobicity | 233 | ||
9.3.2 Surface charge and cation bridging | 238 | ||
9.3.3 Surface roughness and porosity | 241 | ||
9.4 CONCLUDING REMARKS | 243 | ||
9.5 REFERENCES | 243 | ||
10.0 influence of population structure on the performance of biofilm reactors | 247 | ||
10.1 INTRODUCTION | 247 | ||
10.2 INVESTIGATION OF THE BIOTIC STRUCTURE OF BIOFILMS: SURVEY OF METHODS | 249 | ||
10.2.1 Methods for the investigation of the microbial composition of biofilm communities | 249 | ||
10.2.2 Utilization of protozoa and metazoa as indicators of the ecological conditions in and around biofilms | 252 | ||
10.2.3 Microscopic techniques for the examination of biofilm architecture | 253 | ||
10.3 CASE STUDIES | 255 | ||
10.3.1 Membrane-grown bio lms for the treatment of wastewater containing xenobiotics | 255 | ||
10.3.1.1 Results of microbiological examination | 258 | ||
10.3.1.2 Protozoan and metazoan communities of the bio lms | 263 | ||
10.3.2 Nitrifying bio lms in a fluidized bed reactor | 267 | ||
10.3.2.1 Microbial composition of the bio lms | 267 | ||
10.3.2.2 Properties of the carrying material and the bio lm | 271 | ||
10.4 CONCLUDING REMARKS | 273 | ||
10.5 REFERENCES | 274 | ||
11.0 Detatchment: an often-overlooked phenomenon in biofilm research and modelling | 279 | ||
11.1 INTRODUCTION | 279 | ||
11.2 DETATCHMENT MECHANISMS | 280 | ||
11.2.1 Categories | 280 | ||
11.2.2 Experimental determination | 282 | ||
11.2.3 Mathematical description | 283 | ||
11.2.4 Location of detachment | 286 | ||
11.2.5 Residence time distribution | 289 | ||
11.2.6 Summary | 291 | ||
11.3 INFLUENCE OF DETACHMENT ON COMPETITION IN BIOFILMS AND ON OVERALL PROCESS PERFORMANCE | 291 | ||
11.3.1 Competition between fast- and slow-growing bacteria | 292 | ||
11.3.1.1 Influence on overall mass bacterial species | 292 | ||
11.3.1.2 Influence on system performance | 293 | ||
11.3.1.3 Local cell residence time distribution | 294 | ||
11.3.1.4 Summary | 295 | ||
11.3.2 Detachment as a relevant part of the process | 297 | ||
11.3.2.1 Short local residence times are bene cial | 298 | ||
11.3.2.2 Loss of active biomass | 300 | ||
11.3.2.3 Summary | 300 | ||
11.4 CONCLUDING REMARKS | 301 | ||
11.5 REFERENCES | 303 | ||
12.0 Deduction and induction in design and operation of biofilm reactors | 314 | ||
12.1 INTRODUCTION | 314 | ||
12.2 \tTECHNOLOGICAL DEVELOPMENT | 315 | ||
12.2.1\t\tBasic principles | 315 | ||
12.2.2\t\tDemands on performance | 316 | ||
12.3 PRAGMATISM VERSUS THEORY-BASED MODELS | 317 | ||
12.3.1 Engineering craftsmanship | 317 | ||
12.3.2 Science-based determinism | 320 | ||
12.4\tMODELS OF BIOFILM REACTORS | 321 | ||
12.4.1\tModel structure, variables, parameters and forcing input | 321 | ||
12.4.1.1\tModel structure | 321 | ||
12.4.1.2\tModels state variables | 321 | ||
12.4.1.3\tModel parameters | 321 | ||
12.4.1.4\tForcing input | 321 | ||
12.4.2\t\tModel applications | 322 | ||
12.4.2.1\tPlanning tool | 322 | ||
12.4.2.2\tAnalysis of existing plants | 322 | ||
12.4.2.3\tDesign of new plants | 322 | ||
12.4.2.4\tReal-time control of plant | 323 | ||
12.4.2.5\tModels as research tools | 323 | ||
12.4.3\t\tLevel of aggregation | 323 | ||
12.5\tMODEL CALIBRATION AND PARAMETER ESTIMATION | 324 | ||
12.5.1\t\tModel structure | 324 | ||
12.5.2\t\tParameter calibration, veri?cation, and estimation | 325 | ||
12.5.2.1\tCalibration | 325 | ||
12.5.2.2 Veri?cation | 326 | ||
12.5.3\t\tParameter estimation | 327 | ||
12.5.4\t\tExperimental design | 327 | ||
12.5.5\t\tModel uncertainty | 328 | ||
12.5.5.1\tSensitivity analysis | 329 | ||
12.5.5.2\tUncertainty analysis | 329 | ||
12.6\tTREATMENT PLANT DESIGN | 329 | ||
12.6.1\t\tIdenti?cation of problem | 329 | ||
12.6.2\t\tModel for bio?lm system | 330 | ||
12.6.3\t\tPilot plant investigations | 332 | ||
12.7\tANALYSIS OF EXISTING PLANT / PILOT PLANT | 334 | ||
12.7.1 Identi?cation of the problem | 334 | ||
12.7.2\t\tDesign of experimental program | 334 | ||
12.8\tOUTSTANDING ISSUES OF ENGINEERING SIGNIFICANCE | 335 | ||
12.8.1\t\tKey phenomena not fully understood | 335 | ||
12.8.1.1\tBiomass | 335 | ||
12.8.1.2\tPopulation dynamics | 335 | ||
12.8.1.3\tParticulate organic matter | 336 | ||
12.8.1.4\tUnidenti?ed reactions in bio?lms | 336 | ||
12.8.1.5\tDiffusivity into bio?lms | 336 | ||
12.8.1.6\tBiomass control | 337 | ||
12.8.2\t\tResearch needs and engineering development | 337 | ||
12.9\tReferences | 338 | ||
13.0 Effect of clay particles on biofilm composition and reactor efficiency | 340 | ||
13.1 INTRODUCTION | 340 | ||
13.2 PROPERTIES OF CLAY PARTICLES | 341 | ||
13.3 MICROORGANISMS IN SOILS AND IN AQUEOUS SOLUTIONS: INTERACTIONS WITH CLAYS | 344 | ||
13.4 MICROHABITATS CREATED BY CLAY PARTICLES | 346 | ||
13.5 GENETIC EXCHANGE | 347 | ||
13.6 EFFECT OF PARTICLES ON THE TOXICITY OF BIOCIDES | 348 | ||
13.7 EFFECTS OF PARTICLES ON BIOFILM PHYSICAL PROPERTIES | 351 | ||
13.8 CLAY PARTICLES IN WASTEWATER TREATMENT BIOREACTORS | 352 | ||
13.9 SUMMARY AND FUTURE RESEARCH | 353 | ||
13.10 REFERENCES | 355 | ||
14.0 Bioprocess engineering and microbiologists: a profit-sharing alliance | 358 | ||
14.1 POSTULATE | 358 | ||
14.2 ANALYSIS OF THE CURRENT STATE OF BIOTECHNOLOGY | 360 | ||
14.3 CHANCES AND REQUIREMENTS | 365 | ||
14.4 CONCLUDING REMARKS | 369 | ||
14.5 REFERENCES | 370 | ||
APPENDIX: BIOGRAPHY OF MICROBIAL SAMPLES | 372 | ||
From fundamentals to practical applications: Conclusions | 389 | ||
Glossary | 392 | ||
Index | 406 |