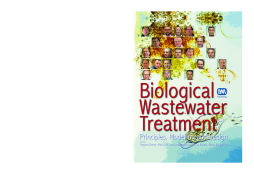
BOOK
Biological Wastewater Treatment
Mogens Henze | Mark C. M. van Loosdrecht | G.A. Ekama | Damir Brdjanovic
(2008)
Additional Information
Book Details
Abstract
For information on the online course in Biological Wastewater Treatment from UNESCO-IHE, visit: http://www.iwapublishing.co.uk/books/biological-wastewater-treatment-online-course-principles-modeling-and-designÂ
Over the past twenty years, the knowledge and understanding of wastewater treatment have advanced extensively and moved away from empirically-based approaches to a first principles approach embracing chemistry, microbiology, physical and bioprocess engineering, and mathematics. Many of these advances have matured to the degree that they have been codified into mathematical models for simulation with computers. For a new generation of young scientists and engineers entering the wastewater treatment profession, the quantity, complexity and diversity of these new developments can be overwhelming, particularly in developing countries where access is not readily available to advanced level tertiary education courses in wastewater treatment. Â
Biological Wastewater Treatment addresses this deficiency. It assembles and integrates the postgraduate course material of a dozen or so professors from research groups around the world that have made significant contributions to the advances in wastewater treatment. The book forms part of an internet-based curriculum in biological wastewater treatment which also includes:
- Â Summarized lecture handouts of the topics covered in bookÂ
- Filmed lectures by the author professorsÂ
- Tutorial exercises for students self-learning Â
Table of Contents
Section Title | Page | Action | Price |
---|---|---|---|
Half Title | 2 | ||
Title | 4 | ||
Copyright | 5 | ||
Preface | 6 | ||
About the book and online course | 8 | ||
Contents | 9 | ||
Chapter 1: Wastewater Treatment Development | 12 | ||
1.1 GLOBAL DRIVERS FOR SANITATION | 12 | ||
1.2 HISTORY OF WASTEWATER TREATMENT | 12 | ||
REFERENCES | 18 | ||
ACKNOWLEDGEMENT | 18 | ||
Chapter 2: Microbial Metabolism | 20 | ||
2.1 INTRODUCTION | 20 | ||
2.2 ELEMENTS OF MICROBIOLOGY | 21 | ||
2.2.1 Classification of microorganisms | 21 | ||
2.2.2 Cell structure and components | 22 | ||
2.2.3 Functions of bacteria | 24 | ||
2.2.4 Characterization of bacteria | 24 | ||
2.2.4.1 Fluorescent in situ hybridization (FISH) | 25 | ||
2.2.4.2 Polymerase chain reaction (PCR) and denaturing gradient gel electrophoresis (DGGE) | 25 | ||
2.2.5 Bacterial bioenergetics | 25 | ||
2.2.6 Nutritional requirements for microbial growth | 26 | ||
2.2.7 Carbon and energy sources and microbial diversity | 27 | ||
2.2.8 Environmental conditions (oxygen, temperature, toxicity) | 29 | ||
2.2.8.1 Oxygen | 29 | ||
2.2.8.2 Temperature | 29 | ||
2.3 STOICHIOMETRY AND ENERGETICS | 30 | ||
2.3.1 Theoretical chemical oxygen demand (thCOD) and electron equivalents | 30 | ||
2.3.2 Cell growth | 31 | ||
2.3.3 Yield and energy | 32 | ||
2.3.3.1 Energy from catabolism | 32 | ||
2.3.3.2 Synthesis fraction and biomass yield | 32 | ||
2.3.3.3 Observed yield from stoichiometry | 35 | ||
2.3.3.4 True yield estimation from bioenergetics | 36 | ||
2.3.3.5 Example: Estimating true yield from bioenergetics for the aerobic oxidation of glucose with ammonia as nitrogen source | 37 | ||
2.4 KINETICS | 38 | ||
2.4.1 Substrate utilisation rate | 38 | ||
2.4.1.1 Saturation function | 38 | ||
2.4.1.2 Inhibition function | 39 | ||
2.4.2 Growth rate | 39 | ||
2.4.3 Stoichiometric and kinetic parameter values | 40 | ||
REFERENCES | 41 | ||
NOMENCLATURE | 41 | ||
Chapter 3: Wastewater Characterization | 44 | ||
3.1 THE ORIGIN OF WASTEWATER | 44 | ||
3.2 WASTEWATER CONSTITUENTS | 44 | ||
3.3 BOD AND COD | 45 | ||
3.4 PERSON EQUIVALENTS AND PERSON LOAD | 46 | ||
3.5 IMPORTANT COMPONENTS | 46 | ||
3.6 SPECIAL COMPONENTS | 47 | ||
3.7 MICROORGANISMS | 48 | ||
3.8 SPECIAL WASTEWATERS AND INTERNAL PLANT RECYCLE STREAMS | 48 | ||
3.9 RATIOS | 51 | ||
3.10 VARIATIONS | 51 | ||
3.11 WASTEWATER FLOWS | 52 | ||
3.12 TRADITIONAL WASTE FROM HOUSEHOLDS | 53 | ||
3.13 WASTEWATER DESIGN FOR HOUSEHOLDS | 54 | ||
3.14 WASTEWATER AND BIOMASS FRACTIONATION | 55 | ||
3.15 SYMBOLS LIST OF VARIABLES FOR MODELS | 56 | ||
3.16 CHARACTERIZATION PROTOCOLS | 56 | ||
3.17 EXAMPLE COMPOSITION OF INFLUENT BIOREACTOR AND EFFLUENT | 56 | ||
3.18 WASTEWATER FINGERPRINT | 56 | ||
REFERENCES | 63 | ||
Chapter 4: Organic Material Removal | 64 | ||
4.1 INTRODUCTION | 64 | ||
4.1.1 Transformations in the biological reactor | 64 | ||
4.1.2 Steady state and dynamic simulation models | 65 | ||
4.2 ACTIVATED SLUDGE SYSTEM CONSTRAINTS | 66 | ||
4.2.1 Mixing regimes | 66 | ||
4.2.2 Sludge retention time (SRT) | 67 | ||
4.2.3 Nominal hydraulic retention time (HRTn) | 67 | ||
4.2.4 Connection between sludge age and hydraulic retention time | 68 | ||
4.3 SOME MODEL SIMPLIFICATIONS | 68 | ||
4.3.1 Complete utilization of biodegradable organics | 68 | ||
4.4 STEADY STATE SYSTEM EQUATIONS | 69 | ||
4.4.1 For the influent | 69 | ||
4.4.2 For the system | 69 | ||
4.4.2.1 Reactor VSS mass | 69 | ||
4.4.2.2 Reactor ISS mass | 70 | ||
4.4.2.3 Reactor TSS mass | 70 | ||
4.4.2.4 Carbonaceous oxygen demand | 71 | ||
4.4.3 Reactor volume and retention time | 71 | ||
4.4.4 Irrelevance of HRT | 71 | ||
4.4.5 Effluent COD concentration | 72 | ||
4.4.6 The COD (or e-) mass balance | 72 | ||
4.4.7 Active fraction of the sludge | 73 | ||
4.4.8 Steady state design | 73 | ||
4.4.9 The steady state design procedure | 74 | ||
4.5 DESIGN EXAMPLE | 74 | ||
4.5.1 Temperature effects | 75 | ||
4.5.2 Calculations for organic material degradation | 75 | ||
4.5.3 The COD mass balance | 77 | ||
4.6 REACTOR VOLUME REQUIREMENTS | 79 | ||
4.7 DETERMINATION OF REACTOR TSS CONCENTRATION | 80 | ||
4.7.1 Reactor cost | 80 | ||
4.7.2 Secondary settling tank cost | 80 | ||
4.7.3 Total cost | 81 | ||
4.8 CARBONACEOUS OXYGEN DEMAND | 82 | ||
4.8.1 Steady state (daily average) conditions | 82 | ||
4.8.2 Daily cyclic (dynamic) conditions | 82 | ||
4.9 DAILY SLUDGE PRODUCTION | 83 | ||
4.10 SYSTEM DESIGN AND CONTROL | 84 | ||
4.10.1 System sludge mass control | 84 | ||
4.10.2 Hydraulic control of sludge age | 86 | ||
4.11 SELECTION OF SLUDGE AGE | 87 | ||
4.11.1 Short sludge ages (1 to 5 days) | 87 | ||
4.11.1.1 Conventional plants | 87 | ||
4.11.1.2 Aerated lagoons | 88 | ||
4.11.2 Intermediate sludge ages (10 to 15 days) | 89 | ||
4.11.3 Long sludge ages (20 days or more) | 90 | ||
4.11.3.1 Aerobic plants | 90 | ||
4.11.3.2 Anoxic-aerobic plants | 91 | ||
4.11.3.3 Anaerobic-anoxic-aerobic plants | 91 | ||
4.11.4 Dominant drivers for activated sludge system size | 92 | ||
4.11.5 Some general comments | 93 | ||
REFERENCES | 94 | ||
NOMENCLATURE | 95 | ||
Chapter 5: Nitrogen Removal | 98 | ||
5.1 INTRODUCTION TO NITRIFICATION | 98 | ||
5.2 BIOLOGICAL KINETICS | 99 | ||
5.2.1 Growth | 99 | ||
5.2.2 Growth behaviour | 100 | ||
5.2.3 Endogenous respiration | 101 | ||
5.3 PROCESS KINETICS | 101 | ||
5.3.1 Effluent ammonia concentration | 101 | ||
5.4 FACTORS INFLUENCING NITRIFICATION | 103 | ||
5.4.1 Influent source | 103 | ||
5.4.2 Temperature | 103 | ||
5.4.3 Unaerated zones | 104 | ||
5.4.3.1 Maximum allowable unaerated mass fraction | 105 | ||
5.4.4 Dissolved oxygen concentration | 106 | ||
5.4.5 Cyclic flow and load | 106 | ||
5.4.6 pH and alkalinity | 107 | ||
5.5 NUTRIENT REQUIREMENTS FOR SLUDGE PRODUCTION | 110 | ||
5.5.1 Nitrogen requirements | 110 | ||
5.5.2 N (and P) removal by sludge production | 111 | ||
5.6 DESIGN CONSIDERATIONS | 112 | ||
5.6.1 Effluent TKN | 112 | ||
5.6.2 Nitrification capacity | 113 | ||
5.7 NITRIFICATION DESIGN EXAMPLE | 114 | ||
5.7.1 Effect of nitrification on mixed liquor pH | 115 | ||
5.7.2 Minimum sludge age for nitrification | 115 | ||
5.7.3 Raw wastewater N concentrations | 116 | ||
5.7.4 Settled wastewater | 116 | ||
5.7.5 Nitrification process behaviour | 116 | ||
5.8 BIOLOGICAL NITROGEN REMOVAL | 119 | ||
5.8.1 Interaction between nitrification and nitrogen removal | 119 | ||
5.8.2 Benefits of denitrification | 119 | ||
5.8.3 Nitrogen removal by denitrification | 120 | ||
5.8.4 Denitrification kinetics | 121 | ||
5.8.5 Denitrification systems | 121 | ||
5.8.5.1 The Ludzack-Ettinger system | 122 | ||
5.8.5.2 The 4 stage Bardenpho system | 123 | ||
5.8.6 Denitrification rates | 123 | ||
5.8.7 Denitrification potential | 126 | ||
5.8.8 Minimum primary anoxic sludge mass fraction | 128 | ||
5.8.9 Denitrification - influence on reactor volume and oxygen demand | 128 | ||
5.9 DEVELOPMENT AND DEMONSTRATION OF DESIGN PROCEDURE | 128 | ||
5.9.1 Review of calculations | 129 | ||
5.9.2 Allocation of unaerated sludge mass fraction | 130 | ||
5.9.3 Denitrification performance of the MLE system | 130 | ||
5.9.3.1 Optimum a-recycle ratio | 130 | ||
5.9.3.2 The balanced MLE system | 134 | ||
5.9.3.3 Effect of influent TKN/COD ratio | 137 | ||
5.9.3.4 MLE sensivity diagram | 138 | ||
5.10 SYSTEM VOLUME AND OXYGEN DEMAND | 141 | ||
5.10.1 System volume | 141 | ||
5.10.2 Daily average total oxygen demand | 141 | ||
5.11 SYSTEM DESIGN, OPERATION AND CONTROL | 143 | ||
REFERENCES | 143 | ||
NOMENCLATURE | 144 | ||
Chapter 6: Innovative Nitrogen Removal | 150 | ||
6.1 INTRODUCTION | 150 | ||
6.2 IMPACT OF SIDE STREAM PROCESSES | 151 | ||
6.3 THE NITROGEN CYCLE | 152 | ||
6.4 NITRITE BASED N-REMOVAL | 154 | ||
6.5 ANAEROBIC AMMONIA OXIDATION | 157 | ||
6.6 BIO-AUGMENTATION | 160 | ||
6.7 CONCLUSIONS | 162 | ||
REFERENCES | 163 | ||
NOMENCLATURE | 164 | ||
Chapter 7: Enhanced Biological Phosphorus Removal | 166 | ||
7.1 INTRODUCTION | 166 | ||
7.2 PRINCIPLE OF ENHANCED BIOLOGICAL PHOSPHORUS REMOVAL (EBPR) | 167 | ||
7.3 MECHANISM OF EBPR | 168 | ||
7.3.1 Background | 168 | ||
7.3.2 Biological P removal microorganisms | 168 | ||
7.3.3 Prerequisites | 169 | ||
7.3.4 Observations | 169 | ||
7.3.5 Biological P removal mechanism | 169 | ||
7.3.5.1 In the anaerobic reactor | 170 | ||
7.3.5.2 In the subsequent aerobic reactor | 171 | ||
7.3.5.3 Quantitative anaerobic-aerobic PAO model | 171 | ||
7.3.6 Fermentable COD and slowly biodegradable COD | 172 | ||
7.3.7 Functions of the anaerobic zone | 172 | ||
7.3.8 Influence of recycling oxygen and nitrate to the anaerobic reactor | 173 | ||
7.3.9 Denitrification by PAOs | 173 | ||
7.3.10 Relationship between influent COD components and sludge components | 173 | ||
7.4 OPTIMISATION AND DEVELOPMENT OF EBPR SYSTEMS | 173 | ||
7.4.1 Principles of EBPR optimization | 173 | ||
7.4.2 Discovery | 175 | ||
7.4.3 PhoStrip® system | 175 | ||
7.4.4 Modified Bardenpho | 177 | ||
7.4.5 Phoredox or anaerobic/oxic (A/O) system | 178 | ||
7.4.6 Effect of nitrate on EBPR | 179 | ||
7.4.7 University of Cape Town (UCT; VIP) system | 180 | ||
7.4.8 Modified UCT system | 181 | ||
7.4.9 Johannesburg (JHB) system | 181 | ||
7.4.10 Biological-chemical phosphorus removal (BCFS® system) | 182 | ||
7.5 MODEL DEVELOPMENT FOR EBPR | 182 | ||
7.5.1 Early developments | 182 | ||
7.5.2 Readily biodegradable COD | 182 | ||
7.5.3 Parametric model | 183 | ||
7.5.4 Comments on the parametric model | 183 | ||
7.5.5 NDEBPR system kinetics | 184 | ||
7.5.6 Enhanced PAO cultures | 184 | ||
7.5.6.1 Enhanced culture development | 184 | ||
7.5.6.2 Enhanced culture kinetic model | 185 | ||
7.5.6.3 Simplified enhanced culture steady state model | 186 | ||
7.5.7 Steady state mixed culture NDEBPR systems | 187 | ||
7.5.7.1 Mixed culture steady state model | 187 | ||
7.5.7.2 Incorporation of denitrification aspects in steady state mixed culture model | 189 | ||
7.6 MIXED CULTURE STEADY STATE MODEL | 190 | ||
7.6.1 Principles of the model | 190 | ||
7.6.2 Mass equations | 191 | ||
7.6.2.1 PAOs | 191 | ||
7.6.2.2 OHOs | 191 | ||
7.6.2.3 Inert mass | 191 | ||
7.6.3 Division of biodegradable COD between PAOs and OHOs | 192 | ||
7.6.3.1 Kinetics of conversion of fermentable organics to VFAs | 192 | ||
7.6.3.2 Effect of recycling nitrate or oxygen | 192 | ||
7.6.3.3 Steady state conversion equations | 193 | ||
7.6.3.4 Implications of conversion theory | 194 | ||
7.6.4 P release | 194 | ||
7.6.5 P removal and effluent total phosphorus concentration | 194 | ||
7.6.6 VSS and TSS sludge masses and P content of TSS | 195 | ||
7.6.6.1 VSS sludge mass | 195 | ||
7.6.6.2 FSS sludge mass | 195 | ||
7.6.6.3 TSS sludge mass and sludge VSS/TSS ratio | 196 | ||
7.6.6.4 P content of TSS | 196 | ||
7.6.7 Process volume requirements | 196 | ||
7.6.8 Nitrogen requirements for sludge production | 197 | ||
7.6.9 Oxygen demand | 197 | ||
7.6.9.1 Carbonaceous oxygen demand | 197 | ||
7.6.9.2 Nitrification oxygen demand | 198 | ||
7.6.9.3 Total oxygen demand | 198 | ||
7.7 DESIGN EXAMPLE | 199 | ||
7.7.1 Steady state design procedure | 199 | ||
7.7.2 Information provided | 199 | ||
7.7.3 Calculations | 201 | ||
7.8 INFLUENCE OF EBPR ON THE SYSTEM | 208 | ||
7.8.1 Influence on volatile and total suspended solids and oxygen demand | 208 | ||
7.8.2 P/VSS ratio | 209 | ||
7.9 FACTORS INFLUENCING THE MAGNITUDE | 210 | ||
7.9.1 Zero discharge of nitrate and oxygen to anaerobic reactor | 210 | ||
7.9.1.1 Sludge age and anaerobic mass fraction | 210 | ||
7.9.1.2 Influent COD | 210 | ||
7.9.1.3 Subdivision of f sub;AN | 211 | ||
7.9.1.4 Settled and unsettled influent | 211 | ||
7.9.1.5 Minimally required aerobic SRT for good EBPR | 211 | ||
7.9.2 Influence of influent RBCOD fraction | 212 | ||
7.9.3 Influence of recycling nitrate and oxygen to the anaerobic reactor | 212 | ||
7.9.4 Influence of temperature on EBPR | 213 | ||
7.9.4.1 Temperature effects on the physiology of EBPR | 214 | ||
7.9.4.2 Process and molecular ecological studies | 214 | ||
7.10 DENITRIFICATION IN NDEBPR SYSTEMS | 215 | ||
7.10.1 Background | 215 | ||
7.10.2 Denitrification potential in NDEBPR systems | 215 | ||
7.10.2.1 Denitrification potential of the primary anoxic reactor | 216 | ||
7.10.2.2 Denitrification potential of the secondary anoxic reactor | 217 | ||
7.10.3 Principles of denitrification design procedures for NDEBPR systems | 217 | ||
7.10.4 Analysis of denitrification in NDEBPR systems | 218 | ||
7.10.4.1 UCT System | 218 | ||
7.10.5 Maximum nitrate recycled to anaerobic reactor | 219 | ||
7.11 GLYCOGEN ACCUMULATING ORGANISMS (GAOS) | 219 | ||
7.12 CONCLUSION AND PERSPECTIVES | 221 | ||
REFERENCES | 221 | ||
ACKNOWLEDEGEMENT | 227 | ||
NOMENCLATURE | 227 | ||
Chapter 8: Pathogen Removal | 232 | ||
8.1 INTRODUCTION | 232 | ||
8.2 TYPES OF ENTERIC PATHOGENS | 232 | ||
8.2.1 Viruses | 233 | ||
8.2.2 Bacteria | 234 | ||
8.2.3 Protozoa | 235 | ||
8.2.4 Helminthes | 236 | ||
8.3 OCCURRENCE OF PATHOGENS IN SEWAGE | 237 | ||
8.3.1 Indicator organisms | 237 | ||
8.3.2 Bacterial indicators | 238 | ||
8.3.3 Bacteriophage as indicators | 239 | ||
8.3.4 Standards and criteria for indicators | 240 | ||
8.4 REMOVAL OF PATHOGENS AND INDICATORS BY WASTEWATER TREATMENT PROCESSES | 241 | ||
8.4.1 Ponds | 241 | ||
8.4.2 Tricking filters | 242 | ||
8.4.3 Activated sludge | 242 | ||
8.4.4 Membrane bioreactors | 243 | ||
8.4.5 Anaerobic reactors | 244 | ||
8.4.6 Wetlands and reed beds | 244 | ||
8.4.7 Land treatment | 245 | ||
8.4.7.1 Overland flow | 245 | ||
8.4.7.2 Infiltration | 245 | ||
8.4.8 Septic tanks | 246 | ||
8.4.9 Tertiary treatment | 246 | ||
8.4.10 Disinfection | 247 | ||
8.4.11 Chlorine disinfection | 247 | ||
8.4.12 Ozone | 248 | ||
8.4.12.1 Estimating the effectiveness of chlorine and ozone | 248 | ||
8.4.13 Ultraviolet light disinfection | 249 | ||
8.5 CONCLUSIONS | 250 | ||
REFERENCES | 251 | ||
NOMENCLATURE | 253 | ||
Chapter 9: Aeration and Mixing | 256 | ||
9.1 AERATION TECHNOLOGY | 256 | ||
9.1.1 Introduction | 256 | ||
9.1.2 Surface aerators | 258 | ||
9.1.3 Coarse-bubble systems | 260 | ||
9.1.4 Fine-bubble systems | 261 | ||
9.2 AIR BLOWER SYSTEMS | 264 | ||
9.2.1 State of the art | 264 | ||
9.2.2 Centrifugal blowers | 264 | ||
9.2.3 Positive displacement blowers | 265 | ||
9.2.4 Variable frequency drives | 265 | ||
9.2.5 Existing control systems | 266 | ||
9.2.6 Blower upgrades and recommendations | 266 | ||
9.3 CONVERTING MANUFACTURERS’ DATA TO PROCESS CONDITIONS | 267 | ||
9.3.1 The impact of cell retention time | 267 | ||
9.3.2 Role of selectors | 268 | ||
9.3.3 Diffuser fouling, scaling, and cleaning | 270 | ||
9.3.4 Surfactant effects | 272 | ||
9.3.5 Aeration performance monitoring | 273 | ||
9.4 SUSTAINABLE AERATION PRACTICE | 274 | ||
9.4.1 Mechanically-simple aerated wastewater treatment systems | 274 | ||
9.4.2 Energy-conservation strategies | 275 | ||
9.5 AERATION REQUIREMENTS | 278 | ||
9.5.1 Design algorithm | 278 | ||
9.5.2 Verification/upgrade algorithm | 279 | ||
REFERENCES | 279 | ||
ACKNOWLEDGEMENTS | 281 | ||
NOMENCLATURE | 281 | ||
Chapter 10: Toxicity | 284 | ||
10.1 INTRODUCTION | 284 | ||
10.2 MEASURES OF TOXICITY | 285 | ||
10.2.1 Respirometry | 285 | ||
10.2.2 Bioluminescence (Microtox®) | 286 | ||
10.2.3 Other tests | 286 | ||
10.2.4 Online toxicity meters | 287 | ||
10.3 KINETIC MODELS FOR TOXIC SUBSTRATES | 287 | ||
10.3.1 Models of enzyme inhibition | 288 | ||
10.3.1.1 Competitive inhibition | 288 | ||
10.3.1.2 Non-competitive inhibition | 289 | ||
10.3.1.3 Un-competitive inhibition | 289 | ||
10.3.2 Inhibition constant | 290 | ||
10.3.3 Substrate inhibition | 290 | ||
10.3.4 Product Inhibition | 291 | ||
10.3.5 Other kinetic expressions | 291 | ||
10.3.6 Physical causes of inhibition | 291 | ||
10.3.6.1 Temperature | 292 | ||
10.3.6.2 pH | 293 | ||
10.4 DEALING WITH TOXICITY | 293 | ||
10.4.1 Performance parameters under inhibition | 294 | ||
10.4.1.1 Case study 10.1: Wastewater treatment in chemical manufacturing | 294 | ||
10.4.1.2 Case study 10.2.: Textile wastewater treatment | 295 | ||
10.4.1.3 Case study 10.3: Urban wastewater treatment | 297 | ||
10.5 CONCLUDING REMARKS | 298 | ||
REFERENCES | 299 | ||
NOMENCLATURE | 300 | ||
Chapter 11: Bulking Sludge | 302 | ||
11.1 INTRODUCTION | 302 | ||
11.2 HISTORICAL ASPECTS | 304 | ||
11.3 RELATIONSHIP BETWEEN MORPHOLOGY AND ECOPHYSIOLOGY | 304 | ||
11.3.1 Microbiological approach | 305 | ||
11.3.2 Morphological-ecological approach | 306 | ||
11.4 FILAMENTOUS BACTERIA IDENTIFICATION AND CHARACTERISATION | 306 | ||
11.4.1 Microscopic characterisation versus molecular methods | 306 | ||
11.4.2 Physiology of filamentous bacteria | 307 | ||
11.5 CURRENT GENERAL THEORIES TO EXPLAIN BULKING SLUDGE | 307 | ||
11.5.1 Diffusion based selection | 308 | ||
11.5.2 Kinetic selection theory | 308 | ||
11.5.3 Storage selection theory | 310 | ||
11.6 REMEDIAL ACTIONS | 310 | ||
11.6.1 Selector | 310 | ||
11.6.1.1 Aerobic selectors | 311 | ||
11.6.1.2 Non-aerated selectors | 312 | ||
11.6.1.3 Anoxic selectors | 312 | ||
11.6.1.4 Anaerobic selectors | 313 | ||
11.7 MATHEMATIC MODELLING | 314 | ||
11.8 GRANULAR SLUDGE | 315 | ||
11.9 CONCLUSIONS | 315 | ||
REFERENCES | 316 | ||
NOMENCLATURE | 318 | ||
Chapter 12: Final Settling | 320 | ||
12.1 INTRODUCTION | 320 | ||
12.1.1 Objective of settling | 320 | ||
12.1.2 Functions of a secondary settling tank | 321 | ||
12.1.2.1 Clarification in secondary settlers | 321 | ||
12.1.2.2 Thickening in secondary settlers | 321 | ||
12.1.2.3 Sludge storage in secondary settlers | 322 | ||
12.2 SETTLING TANK CONFIGURATIONS IN PRACTICE | 322 | ||
12.2.1 Circular clarifiers with radial flow pattern | 322 | ||
12.2.2 Rectangular clarifiers with horizontal flow pattern | 323 | ||
12.2.3 Deep clarifiers with vertical flow pattern | 324 | ||
12.2.4 Improvements common to all clarifier types | 324 | ||
12.2.4.1 Flocculation well | 324 | ||
12.2.4.2 Scum removal | 324 | ||
12.2.4.3 Baffles | 325 | ||
12.2.4.4 Lamellae | 325 | ||
12.2.5 Operational problems | 325 | ||
12.2.5.1 Shallow tanks | 325 | ||
12.2.5.2 Uneven flow distribution | 325 | ||
12.2.5.3 Uneven weir loading | 326 | ||
12.2.5.4 Effect of wind | 326 | ||
12.2.5.5 Sudden temperature changes | 326 | ||
12.2.5.6 Freezing in cold weather | 326 | ||
12.2.5.7 Recycle problems | 327 | ||
12.2.5.8 Algae on weirs | 327 | ||
12.2.5.9 Anaerobic clumps | 327 | ||
12.2.5.10 Birds | 327 | ||
12.2.5.11 Bulking sludge | 327 | ||
12.2.5.12 Rising sludge | 327 | ||
12.3 MEASURES OF SLUDGE SETTLEABILITY | 327 | ||
12.3.1 Sludge Volume Index | 327 | ||
12.3.2 Other test methods | 328 | ||
12.4 FLUX THEORY FOR ESTIMATION OF SETTLING TANK CAPACITY | 328 | ||
12.4.1 Zone Settling Velocity test | 328 | ||
12.4.2 Discrete, flocculent, hindered (zone) and compression settling | 329 | ||
12.4.3 The Vesilind settling function | 330 | ||
12.4.4 Gravity, bulk and total flux curves | 331 | ||
12.4.5 Solids handling criteria limits of the clarifier | 332 | ||
12.4.5.1 Solids Handling Criterion I – minimum solids flux limiting | 332 | ||
12.4.5.2 Solids Handling Criterion II – applied flux (overflow rate) limiting | 333 | ||
12.4.6 State Point Analysis | 333 | ||
12.5 OVERVIEW OF THE USE OF FLUX THEORY AND OTHER METHODS FOR DESIGN AND OPERATION | 336 | ||
12.5.1 Design using flux theory | 337 | ||
12.5.2 Empirical design | 338 | ||
12.5.3 WRC design | 338 | ||
12.5.4 ATV design | 338 | ||
12.5.5 STOWA design | 339 | ||
12.5.6 Comparison of settlers designed using different methods | 340 | ||
12.6 MODELLING OF SECONDARY SETTLERS | 340 | ||
12.6.1 Zero dimensional models | 340 | ||
12.6.2 One-dimensional models | 341 | ||
12.6.3 Computational Fluid Dynamic models | 342 | ||
12.7 DESIGN EXAMPLES | 342 | ||
REFERENCES | 344 | ||
NOMENCLATURE | 345 | ||
Chapter 13: Membrane Bio-reactors | 346 | ||
13.1 MEMBRANE SEPARATION PRINCIPLES | 346 | ||
13.2 THE MEMBRANE BIOREACTOR PROCESS | 347 | ||
13.2.1 MBR process facets | 347 | ||
13.2.2 Process and membrane configurations | 348 | ||
13.2.3 Membrane fouling | 350 | ||
13.2.4 MBR process operation | 352 | ||
13.2.4.1 The membrane material | 352 | ||
13.2.4.2 Cleaning | 353 | ||
13.2.4.3 Feedwater and mixed liquor | 354 | ||
13.2.4.4 Aeration | 354 | ||
13.2.4.5 Sludge withdrawal and characteristics | 356 | ||
13.3 MBR PLANT DESIGN | 356 | ||
13.3.1 Liquid pumping | 356 | ||
13.3.2 Membrane maintenance: cleaning | 356 | ||
13.3.3 Aeration | 357 | ||
13.3.3.1 Aerobic treatment dema | 357 | ||
13.3.3.2 Membrane aeration demand | 358 | ||
13.3.3.3 Design: summary | 359 | ||
13.4 COMMERCIAL MEMBRANE TECHNOLOGIES | 359 | ||
13.4.1 Kubota | 360 | ||
13.4.2 GE Zenon | 363 | ||
13.4.3 KMS (Korea Membrane Separation) | 364 | ||
13.5 iMBR CASE STUDIES | 365 | ||
13.5.1 Swanage, UK | 365 | ||
13.5.2 Nordkanal wastewater treatment works at Kaarst, Germany | 366 | ||
13.5.3 Sewage treatment plant at Sari, Korea | 367 | ||
13.5.4 Summary data | 368 | ||
REFERENCE | 369 | ||
NOMENCLATURE | 370 | ||
Chapter 14: Modelling Activated Sludge Processes | 372 | ||
14.1 WHAT IS A MODEL? | 372 | ||
14.2 WHY MODELLING? | 376 | ||
14.3 MODELLING BASICS | 378 | ||
14.3.1 Model building | 378 | ||
14.3.2 General model set-up | 378 | ||
14.3.3 Stoichiometry | 380 | ||
14.3.4 Kinetics | 381 | ||
14.3.5 Transport | 382 | ||
14.3.6 Matrix notation | 382 | ||
14.4 STEPWISE DEVELOPMENT OF BIOKINETIC MODEL: ASM1 | 385 | ||
14.5 ASM3 | 390 | ||
14.6 METABOLIC MODEL | 392 | ||
14.7 ACTIVATED SLUDGE MODEL DEVELOPMENT HISTORY | 393 | ||
14.8 SIMULATOR ENVIRONMENTS | 396 | ||
14.9 CONCLUSIONS | 396 | ||
REFERENCES | 399 | ||
NOMENCLATURE | 400 | ||
Chapter 15: Process Control | 404 | ||
15.1 DRIVING FORCES AND MOTIVATIONS | 404 | ||
15.2 DISTURBANCES INTO WASTEWATER TREATMENT SYSTEMS | 405 | ||
15.3 THE ROLE OF CONTROL AND AUTOMATION | 408 | ||
15.3.1 Setting the priorities | 409 | ||
15.4 INSTRUMENTATION AND MONITORING | 409 | ||
15.5 THE IMPORTANCE OF DYNAMICS | 411 | ||
15.6 MANIPULATED VARIABLES ANDACTUATORS | 413 | ||
15.6.1 Hydraulic variables | 413 | ||
15.6.2 Chemical addition | 414 | ||
15.6.3 Carbon addition | 414 | ||
15.6.4 Air or oxygen supply | 415 | ||
15.7 BASIC CONTROL CONCEPTS | 415 | ||
15.8 EXAMPLES OF FEEDBACK INWASTE WATER TREATMENT SYSTEMS | 416 | ||
15.9 OPERATING COST SAVINGS DUE TOCONTROL | 420 | ||
15.10 INTEGRATION AND PLANT WIDECONTROL | 421 | ||
15.11 CONCLUDING REMARKS | 422 | ||
REFERENCES | 424 | ||
NOMENCLATURE | 425 | ||
Chapter 16: Anaerobic Wastewater Treatment | 426 | ||
16.1 SUSTAINABILITY IN WASTEWATER TREATMENT | 426 | ||
16.1.1 Definition and environmental benefits of anaerobic processes | 426 | ||
16.2 MICROBIOLOGY OF ANAEROBICCONVERSIONS | 428 | ||
16.2.1 Anaerobic degradation of organic polymers | 428 | ||
16.2.1.1 Hydrolysis | 429 | ||
16.2.1.2 Acidogenesis | 430 | ||
16.2.1.3 Acetogenesis | 431 | ||
16.2.1.4 Methanogenesis | 433 | ||
16.3 PREDICTING THE CH4 PRODUCTION | 434 | ||
16.3.1 COD | 435 | ||
16.4 IMPACTS OF ALTERNATIVE ELECTRON ACCEPTORS | 437 | ||
16.4.1 Bacterial conversions under anoxic conditions | 437 | ||
16.4.1.1 Sulphate reduction | 437 | ||
16.4.1.2 Denitrification | 439 | ||
16.5 WORKING WITH THE COD BALANCE | 440 | ||
16.6 IMMOBILISATION AND SLUDGE GRANULATION | 441 | ||
16.6.1 Mechanism underlying sludge granulation | 442 | ||
16.7 ANAEROBIC REACTOR SYSTEMS | 444 | ||
16.7.1 High-rate anaerobic systems | 444 | ||
16.7.2 Single stage anaerobic reactors | 446 | ||
16.7.2.1 The Anaerobic Contact Process (ACP) | 446 | ||
16.7.2.2 Anaerobic Filters (AF) | 446 | ||
16.7.2.3 Anaerobic Sludge Bed Reactors (ASBR) | 447 | ||
16.7.2.4 Anaerobic expanded and fluidized bed systems (EGSB and FB) | 450 | ||
16.7.2.5 Other anaerobic high rate systems | 452 | ||
16.7.2.6 Acidifying and hydrolytic reactors | 453 | ||
16.8 UPFLOW ANAEROBIC SLUDGE BLANKET (UASB) REACTOR | 453 | ||
16.8.1 Process description | 453 | ||
16.8.2 Design considerations of the UASB reactor | 453 | ||
16.8.2.1 Maximum hydraulic surface loading | 453 | ||
16.8.2.2 Organic loading capacity | 454 | ||
16.8.2.3 Reactor internals | 456 | ||
16.8.3 UASB septic tank | 456 | ||
16.9 ANAEROBIC PROCESS KINETICS | 457 | ||
16.10 ANAEROBIC TREATMENT OF DOMESTIC AND MUNICIPAL SEWAGE | 457 | ||
REFERENCES | 462 | ||
NOMENCLATURE | 465 | ||
Chapter 17: Modelling Biofilms | 468 | ||
17.1 WHAT ARE BIOFILMS? | 468 | ||
17.2 MOTIVATION FOR MODELING BIOFILMS AND HOW TO CHOOSE APPROPRIATE MATHEMATICAL MODELING APPROACHES? | 469 | ||
17.3 MODELING APPROACH FOR A BIOFILM ASSUMING A SINGLE LIMITING SUBSTRATE AND NEGLECTING EXTERNAL MASS TRANSFER RESISTANCE | 470 | ||
17.3.1 Basic equations | 471 | ||
17.3.2 Solutions of the diffusion-reaction biofilm equation for different rate expressions | 472 | ||
17.3.2.1 First order substrate removal rate within the biofilm | 472 | ||
17.3.2.2 Zero order substrate removal rate within the biofilm | 474 | ||
17.3.2.3 Monod kinetics within the biofilm | 476 | ||
17.3.3 Summary of analytical solutions for a single limiting substrate | 477 | ||
17.3.4 SIDEBAR: derivation of reaction diffusion equation (Eq. 17.1) from a mass balance within the biofilm | 477 | ||
17.3.5 SIDEBAR: Overview of AQUASIM | 479 | ||
17.4 EXAMPLE OF HOW JLF = F(CLF) CAN BE USED TO PREDICT BIOFILM REACTOR PERFORMANCE | 480 | ||
17.4.1.1 Analytical solution | 480 | ||
17.4.1.2 Trial and error or iterative approach | 480 | ||
17.4.1.3 Graphical solution | 481 | ||
17.4.1.4 Numerical solution (e.g., using AQUASIM) | 481 | ||
17.5 EFFECT OF EXTERNAL MASS TRANSFER RESISTANCE | 481 | ||
17.5.1 Substrate flux for first order reaction rate with external boundary layer | 482 | ||
17.5.2 Substrate flux for Monod kinetics inside the biofilm with external boundary layer | 482 | ||
17.6 COMBINING GROWTH AND DECAY WITH DETACHMENT | 483 | ||
17.6.1 Influence of detachment (ud,S) on the steady state biofilm thickness (LF) and the substrate flux (JLF) | 484 | ||
17.7 DERIVED PARAMETERS | 485 | ||
17.7.1 Solids retention time | 485 | ||
17.7.2 Smallest effluent substrate concentration supporting biomass growth (Cmin) | 486 | ||
17.7.3 Characteristic times and non-dimensional numbers to describe biofilm dynamics | 487 | ||
17.7.3.1. Application of characteristic times to estimate response times | 488 | ||
17.7.3.2 Non-dimensional numbers: | 489 | ||
17.8 MULTI-COMPONENT DIFFUSION | 490 | ||
17.8.1 Two-component diffusion of electron donor and acceptor | 490 | ||
17.8.2 General case of multi-component diffusion | 492 | ||
17.8.3 Complications for multiple processesinside the biofilm | 493 | ||
17.9 IMPLICATIONS OF SUBSTRATE AVAILABILITY ON LIMITING SUBSTRATES, MICROBIAL COMPETITION, AND REACTOR PERFORMANCE | 493 | ||
17.10 HOW DOES 2D/3D STRUCTURE INFLUENCE BIOFILM PERFORMANCE? | 497 | ||
17.11 MODEL PARAMETERS | 497 | ||
17.11.1 Density (XF) | 498 | ||
17.11.2 Diffusion coefficients (DW, DF) | 498 | ||
17.11.3 External mass transfer (LL, RL) | 498 | ||
17.11.4 Biofilm thickness (LF) and biofilm detachment (ud,S, ud,V, ud,M) | 499 | ||
17.11.5 Caution when using parameters fromother types of models | 500 | ||
17.12 MODELING TOOLS | 500 | ||
REFERENCES | 502 | ||
ACKNOWLEDGEMENTS | 503 | ||
Chapter 18: Biofilm Reactors | 504 | ||
18.1 BIOFILM REACTORS | 504 | ||
18.1.1 Types of reactors | 505 | ||
18.1.1.1 Trickling filters | 505 | ||
18.1.1.2 Rotating biological contactors | 506 | ||
18.1.1.3 Submerged fixed bed biofilm reactors | 507 | ||
18.1.1.4 Fluidized and expanded bed biofilm reactors | 508 | ||
18.1.1.5 Granular sludge reactors | 509 | ||
18.1.1.6 Moving bed biofilm reactors | 510 | ||
18.1.1.7 Hybrid biofilm/activated sludge systems | 510 | ||
18.1.1.8 Membrane attached biofilm reactors | 510 | ||
18.1.2 Choice of different filter material | 511 | ||
18.2 DESIGN PARAMETERS | 511 | ||
18.2.1 Substrate flux and loading rates | 511 | ||
18.2.2 Hydraulic loading | 512 | ||
18.3 HOW TO DETERMINE MAXIMUM DESIGN FLUXES OR DESIGN LOADINGS RATES? | 512 | ||
18.3.1 Model based estimation of the maximum substrate flux | 512 | ||
18.3.1.1 Level 1 design: The compound of interest is the rate limiting substrate | 513 | ||
18.3.1.2 Level 2 design: Removal of the compound of interest is limited by the corresponding electron donor/acceptor | 513 | ||
18.3.1.3 Level 3 design: Removal of the compound of interest is limited by growth processes and microbial competition within the biofilm for substrate and space | 513 | ||
18.3.1.4 Level 4 design: Detailed modeling of concentration profiles and heterogeneous biofilm structure and design for dynamic environmental conditions | 513 | ||
18.3.2 Empirical maximum loading rates | 514 | ||
18.3.3 Design examples | 514 | ||
18.4 OTHER DESIGN CONSIDERATIONS | 518 | ||
18.4.1 Aeration | 518 | ||
18.4.2 Flow distribution | 518 | ||
18.4.3 Biofilm control | 518 | ||
18.4.4 Solids removal | 518 | ||
REFERENCES | 518 | ||
ACKNOWLEDGEMENTS | 519 | ||
NOMENCLATURE | 520 |