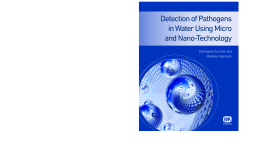
BOOK
Detection of Pathogens in Water Using Micro and Nano-Technology
Giampaolo Zuccheri | Nikolaos Asproulis
(2012)
Additional Information
Book Details
Abstract
Detection of Pathogens in Water Using Micro and Nano-Technology aims to promote the uptake of innovative micro and nano-technological approaches towards the development of an integrated, cost-effective nano-biological sensor useful for security and environmental assays.
The book describes the concerted efforts of a large European research project and the achievements of additional leading research groups. The reported knowledge and expertise should support in the innovation and integration of often separated unitary processes. Sampling, cell lysis and DNA/RNA extraction, DNA hybridisation detection micro- and nanosensors, microfluidics, together also with computational modelling and risk assessment can be integrated in the framework of the current and evolving European regulations and needs. The development and uptake of molecular methods is revolutionizing the field of waterborne pathogens detection, commonly performed with time-consuming cultural methods. The molecular detection methods are enabling the development of integrated instruments based on biosensor that will ultimately automate the full pathway of the microbiological analysis of water.
Editors: Giampaolo Zuccheri, University of Bologna, Italy and Nikolaos Asproulis, Cranfield University, UK
Table of Contents
Section Title | Page | Action | Price |
---|---|---|---|
Cover page | 1 | ||
Half-title page | 2 | ||
Title page | 3 | ||
Copyright page | 4 | ||
Contents | 5 | ||
Preface | 13 | ||
Contributors | 16 | ||
Chapter 1 | 21 | ||
Overview of European regulation and standards on microbiological water analysis | 21 | ||
1.1 INTRODUCTION | 21 | ||
1.2 EUROPEAN REGULATION ON MICROBIOLOGICAL ANALYSIS OF DRINKING WATER | 21 | ||
1.3 EUROPEAN REGULATION ON MICROBIOLOGICAL ANALYSIS OF RECREATIONALWATER | 22 | ||
1.3.1 Case of non-treated recreational water (seas, rivers, coastal water…) | 22 | ||
1.3.2 Case of treated recreational water (swimming & spa pools etc.) | 23 | ||
1.4 EUROPEAN AND INTERNATIONAL STANDARDS FOR MICROBIOLOGICALWATER ANALYSIS | 23 | ||
ABBREVIATIONS | 26 | ||
REFERENCES | 26 | ||
Chapter 2 | 27 | ||
Risk analysis of bio-terroristic attacks on drinking water systems | 27 | ||
2.1 INTRODUCTION | 27 | ||
2.2 DEFINITIONS | 28 | ||
2.3 RISK ANALYSIS FOR TERRORISM | 29 | ||
2.3.1 Critical infrastructure and key asset inventory | 30 | ||
2.3.2 Criticality assessment | 30 | ||
2.3.3 Threat assessment | 30 | ||
2.3.4 Vulnerability assessment | 32 | ||
2.3.5 Risk evaluation | 32 | ||
2.4 RISK ANALYSIS OF BIO-TERRORISTIC ATTACKS ON DRINKING WATER SYSTEMS | 32 | ||
2.4.1 Threat assessment | 32 | ||
2.4.2 Criticality assessment | 41 | ||
2.4.3 Vulnerability assessment | 41 | ||
2.5 RISK ESTIMATION | 42 | ||
2.5.1 Hierarchical risk estimation | 42 | ||
2.5.2 Quantitative microbial risk assessment | 45 | ||
2.5.3 Data sources | 46 | ||
REFERENCES | 47 | ||
Chapter 3 | 53 | ||
Sample collection procedures for Online Contaminant Monitoring System | 53 | ||
3.1 INTRODUCTION | 53 | ||
3.2 MICROBIAL MONITORING OF DRINKING WATER | 54 | ||
3.3 SAMPLING PLAN | 56 | ||
3.3.1 What should the sampling plan include? | 57 | ||
3.4 NEW SECURITY APPROACHES FOR DRINKING WATER | 60 | ||
3.4.1 Water sources | 61 | ||
3.4.2 Raw water transport | 62 | ||
3.4.3 Treatment plants | 62 | ||
3.4.4 Service reservoirs and distribution | 62 | ||
3.5 NEW APPROACH OF ONLINE CONTAMINATION MONITORING DEVICE (OCMD) | 63 | ||
3.5.1 Location of OCMD in water system | 64 | ||
REFERENCES | 65 | ||
Chapter 4 | 67 | ||
A device to extract highly diluted specimens out of large volumes of water for analysis in lab-on-a-chip detection systems | 67 | ||
4.1 THE NEED FOR A MACRO-TO-MICRO-FLUIDIC INTERFACE | 67 | ||
4.1.1 The DINAMICS EU research project\r | 67 | ||
4.1.2 Device needed for generating input for analytics | 67 | ||
4.2 PRINCIPLES OF SEPARATION | 68 | ||
4.2.1 Survey of concentration methods | 68 | ||
4.3 THE NEEDLE IN THE HAYSTACK | 72 | ||
4.4 TECHNICAL DESCRIPTION OF THE DINAMICS CONCENTRATION APPARATUS | 73 | ||
4.4.1 First draft of the dead-end filtration system | 74 | ||
4.4.2 Tangential (cross-flow) filtration system | 76 | ||
4.4.3 Steps towards a continuous working device | 76 | ||
4.5 CONCLUSIONS | 79 | ||
REFERENCES | 79 | ||
Chapter 5 | 80 | ||
Sustainable DNA/RNA release methods for in-line waterborne pathogen screening devices | 80 | ||
DEFINITIONS AND GLOSSARY | 80 | ||
5.1 INTRODUCTION | 80 | ||
5.2 SURVEY ON PATHOGEN-LYSIS/CELL-DISRUPTION METHODS | 82 | ||
5.2.1 Examples for devices utilizing electric field | 84 | ||
5.2.2 Examples for devices harnessing mechanical impact | 86 | ||
5.2.3 Chemical impact based methods (i.e. no Cell-Wall digestive enzymes added) | 91 | ||
5.3 CONSIDERATIONS FOR WATER SAMPLES AND IN-LINE (QUASI CONTINUOUS) OPERATION | 91 | ||
5.4 THE REALISED CASE IN THE DINAMICS PROJECT | 95 | ||
REFERENCES | 98 | ||
Chapter 6 | 100 | ||
The microsystem based core of the DINAMICS water testing system: Design considerations and realization of the chip units | 100 | ||
6.1 MICROFLUIDICS IN DINAMICS | 100 | ||
6.2 LAYOUT OF THE FLUIDIC PARTS OF DINAMICS | 100 | ||
6.3 MICROFLUIDIC COMPONENTS IN DINAMICS | 102 | ||
6.3.1 The valve component | 102 | ||
6.3.2 The mixer component | 103 | ||
6.3.3 The DNA isolation component | 105 | ||
6.3.4 The PCR reaction component | 107 | ||
6.3.5 The hybridization chamber component | 109 | ||
6.3.6 Fabrication – Technology platform | 109 | ||
6.4 CURRENT STATUS | 111 | ||
6.5 CONCLUSION | 112 | ||
REFERENCES | 112 | ||
Chapter 7 | 113 | ||
Electrochemical biosensor strategies for pathogen detection in water security | 113 | ||
7.1 INTRODUCTION | 113 | ||
7.2 BIOSENSOR STRATEGIES FOR PATHOGEN DETECTION IN WATER SECURITY | 113 | ||
7.2.1 Conventional laboratory analysis | 114 | ||
7.2.2 Biosensor analysis | 115 | ||
7.2.3 Conclusions | 119 | ||
7.3 COMMON ELECTROCHEMICAL DETECTION SYSTEMS FOR DNA BIOSENSORS | 119 | ||
7.4 THE ON-CHIP SIMPLIFIED ELECTROCHEMICAL TECHNIQUE DEVELOPED IN THE DINAMICS PROJECT | 124 | ||
7.4.1 On-chip voltammetry | 124 | ||
7.4.2 Electrochemical reporter | 125 | ||
7.4.3 Measurement principle | 125 | ||
7.4.4 Interpretation of the measurements | 126 | ||
7.4.5 Results | 127 | ||
7.4.6 Conclusions | 129 | ||
Acknowledgements | 129 | ||
REFERENCES | 129 | ||
Chapter 8 | 131 | ||
Biochemical and nanotechnological strategies for signal enhancement in the detection of nucleic acids with biosensors | 131 | ||
8.1 INTRODUCTION | 131 | ||
8.1.1 Rationale of the classification | 132 | ||
8.2 ENHANCEMENT METHODS BASED ON ENZYMATIC (OR CATALYZED) REACTIONS | 133 | ||
8.2.1 Peroxidase to enhance the signal of nucleic acids detection | 134 | ||
8.2.2 One to several instances of alkaline phosphatase for the electrochemical detection of nucleic acids | 134 | ||
8.2.3 Terminal transferase to grow DNA at the recognition site | 136 | ||
8.2.4 Signal enhancement of fluorescence through the use of a nickase | 136 | ||
8.2.5 RNase H as a target recycling operator for RNA-based sensors | 137 | ||
8.2.6 Nucleic acid sequence-based amplification (NASBA) | 138 | ||
8.2.7 Strand displacement amplification | 139 | ||
8.2.8 Loop mediated isothermal amplification (LAMP) | 139 | ||
8.2.9 Metal nanoparticles as active enhancer labels | 140 | ||
8.3 ENHANCEMENT METHODS BASED ON NANOPARTICLES OR NANOSTRUCTURES\r | 140 | ||
8.3.1 Methods that employ liposomes | 140 | ||
8.3.2 Fluorescent nanoparticle labels | 141 | ||
8.3.3 Colorimetric assays with metal nanoparticles | 141 | ||
8.3.4 Signal amplification by conjugate breakdown: the bio-barcode assay | 142 | ||
8.3.5 Quantum dots instead of organic dyes | 142 | ||
8.4 METHOD BASED ON DNA NANOSTRUCTURES | 142 | ||
8.4.1 Cascade signal amplification by the combined use of rolling circle amplification | 143 | ||
8.4.2 Branched DNA signal amplification | 144 | ||
8.4.3 Aptamers and DNAzymes | 145 | ||
8.4.4 Hybridization chain reaction and surface-initiated DNA polymerization | 145 | ||
8.5 CONCLUSIONS AND PERSPECTIVES | 148 | ||
REFERENCES | 148 | ||
Chapter 9 | 153 | ||
Computational modelling of aqueous environments in micro and nanochannels | 153 | ||
9.1 INTRODUCTION | 153 | ||
9.2 EFFECTS OF PHYSICAL CHARACTERISTICS\r | 155 | ||
9.2.1 Surface roughness | 155 | ||
9.2.2 Surface stiffness | 155 | ||
9.2.3 Wetting – surface energy – contact angle | 156 | ||
9.2.4 Shear rate – pressure | 156 | ||
9.3 COMPUTATIONAL APPROACHES\r | 157 | ||
9.3.1 Atomistic modelling | 157 | ||
9.3.2 Continuum modelling | 159 | ||
9.4 LIQUID FLOW IN CONFINED GEOMETRIES 9.4.1 Flow behaviour in nanochannels | 168 | ||
9.5 MOLECULAR MODELLING OF WATER | 175 | ||
REFERENCES | 176 | ||
Chapter 10 | 180 | ||
Computational recipes of transport phenomena in micro and nanofluidics | 180 | ||
10.1 INTRODUCTION | 180 | ||
10.2 MODELLING APPROACHES | 181 | ||
10.2.1 Modelling multiple scales | 181 | ||
10.2.2 Brownian motion | 182 | ||
10.2.4 Continuum scale diffusion | 184 | ||
10.3 META-MODELLING FOR MACROMOLECULES | 186 | ||
10.4 HYBRID CONTINUUM-MOLECULAR MODELS | 187 | ||
REFERENCES | 188 | ||
Chapter 11 | 190 | ||
Multi-detection of waterborne pathogens in raw and treated water samples by using ultrafiltration concentration and DNA array te | 190 | ||
11.1 INTRODUCTION | 190 | ||
11.2 IMPROVED AND SIMPLIFIED METHOD FOR CONCENTRATING VIRAL, BACTERIAL, AND PROTOZOAN PATHOGENS | 191 | ||
11.2.1 Technical challenges for a universal concentration protocol | 191 | ||
11.2.2 Protocol | 194 | ||
11.3 INTEGRATED PROTOCOL FOR NUCLEIC ACID EXTRACTION, AMPLIFICATION AND SEQUENCE IDENTIFICATION THROUGH HIGH DENSITY MICROARRAY | 195 | ||
11.4 RESULTS | 198 | ||
11.4.1 Recovery from 30 L-initial volume to final concentrate | 198 | ||
11.4.2 Impact of BSA blocking and elution agents on waterborne pathogen recovery using two-step ultrafiltration protocol from 30 | 199 | ||
11.4.3 Back volume calculation and comparison with other available detection methods | 201 | ||
11.4.4 Multi-detection of waterborne pathogens by DNA Chip hybridization | 201 | ||
11.5 CONCLUSIONS | 203 | ||
Acknowledgements | 204 | ||
REFERENCES | 204 | ||
Chapter 12 | 207 | ||
Detection and enumeration of waterborne mycobacteria | 207 | ||
12.1 ECOLOGY OF WATERBORNE MYCOBACTERIA | 207 | ||
12.1.1 Mycobacterial diseases | 207 | ||
12.1.2 Mycobacterial habitats | 208 | ||
12.1.3 Transmission of mycobacteria | 208 | ||
12.2 PHYSIOLOGICAL ECOLOGY OF WATERBORNE MYCOBACTERIA | 208 | ||
12.2.1 The lipid-rich mycobacterial envelope | 209 | ||
12.2.2 Consequences of the slow growth of mycobacteria | 209 | ||
12.2.3 Viable but unculturable mycobacteria | 210 | ||
12.3 RISK ANALYSIS AND SOURCE-TRACKING ENVIRONMENTAL MYCOBACTERIA | 210 | ||
12.3.1 Mycobacterium avium and the candidate contaminant list | 210 | ||
12.3.2 Risk analysis for mycobacteria | 211 | ||
12.3.3 Source-tracking and DNA fingerprinting | 211 | ||
12.4 SAMPLING AND SAMPLE TREATMENT STRATEGIES FOR MYCOBACTERIAL DETECTION AND ENUMERATION | 211 | ||
12.4.1 Sampling strategies | 211 | ||
12.4.2 Sample treatment | 212 | ||
12.4.3 Sample concentration methods | 212 | ||
12.5 MYCOBACTERIAL DETECTION OR ENUMERATION | 213 | ||
12.5.1 Detection or enumeration | 213 | ||
12.5.2 Culture, PCR, or qPCR | 213 | ||
12.5.3 Culture of mycobacteria | 213 | ||
12.5.4 PCR-detection and qPCR enumeration of mycobacteria | 214 | ||
REFERENCES | 214 | ||
Chapter 13 | 219 | ||
New molecular technologies for the rapid detection of Legionella in water | 219 | ||
13.1 INTRODUCTION | 219 | ||
13.2 IMMUNODETECTION AND FAST DETECTION\r | 220 | ||
13.3 LEGIONELLA DETECTION USING MICROFLUIDICS | 221 | ||
13.3.1 Microarray platforms using antibodies | 221 | ||
13.3.2 Microarray platforms using DNA | 223 | ||
13.4 FUTURE RESEARCH DIRECTIONS | 227 | ||
REFERENCES | 228 | ||
Chapter 14 | 229 | ||
Detection of virus in the water environment | 229 | ||
14.1 INTRODUCTION | 229 | ||
14.1.1 The waterborne viruses | 229 | ||
14.1.2 Transmission of virus in the water environment | 231 | ||
14.1.3 Virus in wastewater treatment plants | 234 | ||
14.1.4 Monitoring of virus in sewage water – an epidemiological tool | 234 | ||
14.2 CONCENTRATION OF VIRUS FROM WATER SAMPLES | 235 | ||
14.2.1 Concentration based on ionic charge (electrostatic adsorption/elution) | 235 | ||
14.2.2 Concentration based on particle size separation (ultrafiltration) | 238 | ||
14.2.3 Other concentration techniques | 239 | ||
14.3 DETECTION AND QUANTIFICATION METHODS | 241 | ||
14.3.1 Cell culture assays | 241 | ||
14.3.2 Molecular assays (PCR and real-time PCR) | 243 | ||
14.3.3 ICC-PCR and detection of viral mRNA | 244 | ||
14.4 PERSPECTIVES | 244 | ||
Acknowledgements | 245 | ||
REFERENCES | 245 | ||
Chapter 15 | 252 | ||
Design of PCR primers for the detection of waterborne bacteria | 252 | ||
15.1 INTRODUCTION | 252 | ||
15.2 THE TARGET GENES | 252 | ||
15.2.1 rRNA genes | 253 | ||
15.2.2 Housekeeping genes | 253 | ||
15.2.3 Pathogenicity genes | 253 | ||
15.2.4 Deep sequencing | 253 | ||
15.3 DESIGN OF PCR PRIMERS | 254 | ||
15.3.1 The features of PCR primers | 254 | ||
15.3.2 The softwares for designing PCR primers | 255 | ||
15.4 DNA-BASED DETECTION TECHNOLOGIES\r | 258 | ||
15.4.1 Specific detection | 258 | ||
15.4.2 Global detection: the sequencing | 265 | ||
15.5 CONCLUSIONS | 266 | ||
REFERENCES | 267 | ||
Chapter 16 | 270 | ||
Fluid structure and boundary slippage in nanoscale liquid films | 270 | ||
16.1 ABSTRACT | 270 | ||
16.2 INTRODUCTION | 270 | ||
16.3 MOLECULAR DYNAMICS SIMULATION MODEL | 272 | ||
16.4 RESULTS\r | 275 | ||
16.4.1 Fluid density, velocity, and temperature profiles | 275 | ||
16.4.2 Shear viscosity and slip length | 277 | ||
16.4.3 Friction coefficient versus slip velocity | 279 | ||
16.4.4 Friction coefficient and induced fluid structure | 282 | ||
16.5 CONCLUSIONS | 287 | ||
Acknowledgments | 288 | ||
REFERENCES | 288 | ||
Chapter 17 | 291 | ||
Understanding slip at the nanoscale in fluid flows using atomistic simulations | 291 | ||
17.1 INTRODUCTION – DEFINITION OF SLIP\r | 291 | ||
17.1.1 Continuum theory and slip | 291 | ||
17.1.2 Incorporating velocity slip in continuum models | 292 | ||
17.2 IMPORTANCE OF SLIP | 292 | ||
17.3 EXPERIMENTAL MEASUREMENT OF SLIP | 294 | ||
17.4 ATOMISTIC SIMULATIONS | 294 | ||
17.4.1 Methodological issues | 295 | ||
17.4.2 Property calculations | 298 | ||
17.4.3 Transport properties | 298 | ||
17.4.4 Slip velocity/length calculation | 299 | ||
17.5 ATOMISTIC SIMULATIONS RESULTS ABOUT SLIP | 300 | ||
17.5.1 Wall roughness effects | 302 | ||
17.5.2 Effect of periodic wall patterns | 303 | ||
17.5.3 Effect of nanostripes | 306 | ||
17.6 CONCLUSIONS | 308 | ||
REFERENCES | 308 |