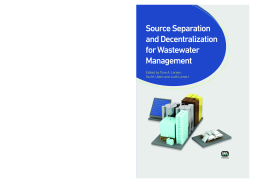
BOOK
Source Separation and Decentralization for Wastewater Management
Tove A. Larsen | Kai M. Udert | Judit Lienert
(2013)
Additional Information
Book Details
Abstract
Is sewer-based wastewater treatment really the optimal technical solution in urban water management? This paradigm is increasingly being questioned. Growing water scarcity and the insight that water will be an important limiting factor for the quality of urban life are main drivers for new approaches in wastewater management.
Source Separation and Decentralization for Wastewater Management sets up a comprehensive view of the resources involved in urban water management. It explores the potential of source separation and decentralization to provide viable alternatives to sewer-based urban water management. During the 1990s, several research groups started working on source-separating technologies for wastewater treatment. Source separation was not new, but had only been propagated as a cheap and environmentally friendly technology for the poor. The novelty was the discussion whether source separation could be a sustainable alternative to existing end-of-pipe systems, even in urban areas and industrialized countries. Since then, sustainable resource management and many different source-separating technologies have been investigated. The theoretical framework and also possible technologies have now developed to a more mature state. At the same time, many interesting technologies to process combined or concentrated wastewaters have evolved, which are equally suited for the treatment of source-separated domestic wastewater.
The book presents a comprehensive view of the state of the art of source separation and decentralization. It discusses the technical possibilities and practical experience with source separation in different countries around the world. The area is in rapid development, but many of the fundamental insights presented in this book will stay valid. Source Separation and Decentralization for Wastewater Management is intended for all professionals and researchers interested in wastewater management, whether or not they are familiar with source separation.
Editors: Tove A. Larsen, Kai M. Udert and Judit Lienert, Eawag - Swiss Federal Institute of Aquatic Science and Technology, Switzerland.
Contributors: Yuval Alfiya, Technion - Israel Institute of Technology, Faculty of Civil and Environmental Engineering; Prof. Dr. M. Bruce Beck, University of Georgia, Warnell School of Forestry and Natural Resources; Dr. Christian Binz, Eawag, Swiss Federal Institute of Aquatic Science and Technology, Innovation Research in Utility Sectors (Cirus); Prof. em. Dr. Markus Boller, Eawag, Swiss Federal Institute of Aquatic Science and Technology, Department of Urban Water Management (SWW); Prof. Dr. Eran Friedler, Technion – Israel Institute of Technology, Faculty of Civil and Environmental Engineering; Zenah Bradford-Hartke, The University of New South Wales, School of Chemical Engineering and UNESCO Centre for Membrane Science and Technology; Dr. Shelley Brown-Malker, Very Small Particle Company Ltd; Bert Bundervoet, Ghent University, Laboratory Microbial Ecology and Technology (LabMET); Prof. Dr. David Butler, University of Exeter, Centre for Water Systems; Dr. Christopher A. Buzie, Hamburg University of Technology, Institute of Wastewater Management and Water Protection; Dr. Dana Cordell, University of Technology, Sydney (UTS), Institute for Sustainable Futures (ISF); Dr. Vasileios Diamantis, Democritus University of Thrace, Department of Environmental Engineering; Prof. Dr. Jan Willem Erisman, Louis Bolk Institute; VU University Amsterdam, Department of Earth Sciences; Barbara Evans, University of Leeds, School of Civil Engineering; Prof. Dr. Malin Falkenmark, Stockholm International Water Institute; Dr. Ted Gardner, Central Queensland University, Institute for Resource Industries and Sustainability; Dr. Heiko Gebauer, Eawag, Swiss Federal Institute of Aquatic Science and Technology, Innovation Research in Utility Sectors (Cirus); Prof. em. Dr. Willi Gujer, Swiss Federal Institute of Technology Zürich (ETHZ), Department of Civil, Environmental and Geomatic Engineering (BAUG); Prof. Dr. Bruce Jefferson, Cranfield University, Cranfield Water Science Institute; Prof. Dr. Paul Jeffrey, Cranfield University, Cranfield Water Science Institute; Sarina Jenni, Eawag, Swiss Federal Institute of Aquatic Science and Technology, Process Engineering Department (Eng); Prof. Dr. Håkan Jönsson, SLU - Swedish University of Agricultural Sciences, Department of Energy and Technology; Prof. Dr. Ïsik Kabdasli, Ïstanbul Technical University, Civil Engineering Faculty; Prof. Dr. Jörg Keller, The University of Queensland, Advanced Water Management Centre (AWMC); Prof. Dr. Klaus Kömmerer, Leuphana Universität Lüneburg, Institute of Sustainable and Environmental Chemistry; Dr. Katarzyna Kujawa-Roeleveld, Wageningen University, Agrotechnology and Food Sciences Group; Dr. Tove A. Larsen, Eawag, Swiss Federal Institute of Aquatic Science and Technology, Department of Urban Water Management (SWW); Michele Laureni, Eawag, Swiss Federal Institute of Aquatic Science and Technology, Process Engineering Department (Eng); Prof. Dr. Gregory Leslie, The University of New South Wales, School of Chemical Engineering and UNESCO Centre for Membrane Science and Technology; Dr. Harold Leverenz, University of California at Davis, Department of Civil and Environmental Engineering; Dr. Judit Lienert, Eawag, Swiss Federal Institute of Aquatic Science and Technology, Department of Environmental Social Sciences (ESS); Prof. Dr. Jürg Londong, Bauhaus-Universität Weimar, Department of Urban Water Management and Sanitation; Dr. Christoph Lüthi, Eawag, Swiss Federal Institute of Aquatic Science and Technology, Water and Sanitation in Developing Countries (Sandec); Prof. Dr. Max Maurer, Eawag, Swiss Federal Institute of Aquatic Science and Technology, Department of Urban Water Management (SWW); Swiss Federal Institute of Technology Zürich (ETHZ), Department of Civil, Environmental and Geomatic Engineering; Prof. em. Dr. Gustaf Olsson, Lund University, Department of Measurement Technology and Industrial Electrical Engineering (MIE); Prof. Dr. Ralf Otterpohl, Hamburg University of Technology, Institute of Wastewater Management and Water Protection; Dr. Bert Palsma, STOWA, Dutch Foundation for Applied Water Research; Dr. Arne R. Panesar, Deutsche Gesellschaft für Internationale Zusammenarbeit (GIZ) GmbH; Prof. Dr. Bruce E. Rittmann, Arizona State University, Swette Center for Environmental Biotechnology; Prof. Dr. Hansruedi Siegrist, Eawag, Swiss Federal Institute of Aquatic Science and Technology, Process Engineering Department (Eng); Dr. Ashok Sharma, Commonwealth Scientific and Industrial Research Organisation, Australia, Land and Water Division; Prof. Dr. Thor Axel Stenström, Stockholm Environment Institute, Bioresources Group; Norwegian University of Life Sciences, Department of Mathematical Science and Technology; Dr. Eckhard Störmer, Eawag, Swiss Federal Institute of Aquatic Science and Technology, Innovation Research in Utility Sectors (Cirus); Bjartur Swart, STOWA, Dutch Foundation for Applied Water Research; MWH North Europe; Prof. em. Dr. George Tchobanoglous, University of California at Davis, Department of Civil and Environmental Engineering; Elizabeth Tilley, Eawag, Swiss Federal Institute of Aquatic Science and Technology, Department of Water and Sanitation in Developing Countries (Sandec); Swiss Federal Institute of Technology Zürich (ETHZ), Centre for Development and Cooperation (NADEL); Prof. Dr. Bernhard Truffer, Eawag, Swiss Federal Institute of Aquatic Science and Technology; Innovation Research in Utility Sectors (Cirus); Prof. Dr. Olcay Tünay, Ïstanbul Technical University, Civil Engineering Faculty; Dr. Kai M. Udert, Eawag, Swiss Federal Institute of Aquatic Science and Technology, Process Engineering Department (Eng); Prof. em. Dr. Willy Verstraete, Ghent University, Laboratory Microbial Ecology and Technology (LabMET); Prof. Dr. Björn Vinnerås, SLU - Swedish University of Agricultural Sciences, Department of Energy and Technology; Prof. Dr. Urs von Gunten, Eawag, Swiss Federal Institute of Aquatic Science and Technology, Department of Water Resources and Drinking Water (W+T); Ecole Polytechnique Fédérale de Lausanne (EPFL),School of Architecture, Civil and Environmental Engineering (ENAC); Prof. em. Dr. Peter A. Wilderer, Technische Universität München, Institute for Advanced Study; Prof. Dr. Jun Xia, Chinese Academy of Sciences (CAS), Center for Water Resources Research and Key Laboratory of Water Cycle and Related Surface Processes; Prof. Dr. Grietje Zeeman, Wageningen University, Agrotechnology and Food Sciences Group
Table of Contents
Section Title | Page | Action | Price |
---|---|---|---|
Cover\r | Cover | ||
Contents | v | ||
Authors’ List | xxi | ||
Preface | xxxi | ||
Chapter 1: Editorial\r | 1 | ||
Part I: The advantages of source separation and decentralization\r | 11 | ||
Chapter 2: The energy issue in urban water management\r | 12 | ||
2.1 INTRODUCTION – THINK GLOBALLY AND ACT LOCALLY | 12 | ||
2.2 GLOBAL ENERGY GOAL | 13 | ||
2.3 RENEWABLE ENERGY SOURCES | 14 | ||
2.4 PHOTOSYNTHESIS, BIOMASS, AND BOD | 14 | ||
2.5 MICROBIAL ENERGY CONVERSION | 15 | ||
2.6 NUTRIENT RECOVERY | 19 | ||
2.7 NEW BIOMASS FROM PHOTOSYNTHETIC MICROORGANISMS | 20 | ||
2.8 LOWER ENERGY USE | 22 | ||
2.9 THE IMPACT OF SOURCE SEPARATION AND DECENTRALIZATION | 23 | ||
REFERENCES | 24 | ||
Chapter 3: Peak phosphorus and the role of P recovery in achieving food security\r | 27 | ||
3.1 INTRODUCTION | 27 | ||
3.2 PHOSPHORUS AND GLOBAL FOOD SECURITY | 27 | ||
3.3 GLOBAL PHOSPHORUS SCARCITY AND POLLUTION | 28 | ||
3.4 FIVE DIMENSIONS OF PHOSPHORUS SCARCITY | 30 | ||
3.5 PHOSPHORUS USE IN THE GLOBAL FOOD SYSTEM | 34 | ||
3.6 ACHIEVING PHOSPHORUS SECURITY | 36 | ||
3.6.1 An integrated approach is required | 37 | ||
3.6.2 The role of decentralized sanitation systems | 38 | ||
3.6.3 Key challenges and opportunities | 38 | ||
3.7 CONCLUSIONS | 39 | ||
REFERENCES | 40 | ||
Chapter 4: Nitrogen economy of the 21st Century\r | 43 | ||
4.1 INTRODUCTION | 43 | ||
4.2 NITROGEN SOURCES | 44 | ||
4.3 RELEASE OF NITROGEN TO THE ENVIRONMENT | 45 | ||
4.4 ENVIRONMENTAL CONSEQUENCES | 49 | ||
4.5 THE FUTURE AND POSSIBLE INTERVENTIONS | 51 | ||
4.6 CONCLUSIONS | 53 | ||
REFERENCES | 54 | ||
Chapter 5: Urban water supply under expanding water scarcity\r | 57 | ||
5.1 INTRODUCTION | 57 | ||
5.2 WATER SUPPLY OF URBAN AREAS | 57 | ||
5.2.1 On the verge of a new water scarcity | 59 | ||
5.3 IMPLICATIONS OF INCREASING COMPETITION? | 60 | ||
5.4 ADAPTING TO INCREASING WATER SHORTAGE | 61 | ||
5.5 REASONABLE BLUE-WATER ALLOCATION | 63 | ||
5.6 IRRIGATION POTENTIAL | 65 | ||
5.7 ADDITIVE VERSUS COMPETING WATER USES | 66 | ||
5.8 CONCLUSION | 66 | ||
Acknowledgement | 67 | ||
REFERENCES | 67 | ||
Chapter 6: The issue of micropollutants in urban water management | 69 | ||
6.1 INTRODUCTION | 69 | ||
6.2 PARENT COMPOUNDS, METABOLITES AND TRANSFORMATION PRODUCTS | 70 | ||
6.3 CLASSIFICATION | 71 | ||
6.4 SOME EXAMPLES OF MICROPOLLUTANTS | 71 | ||
6.4.1 Flame retardants | 72 | ||
6.4.2 Biocides and pesticides | 72 | ||
6.4.3 Endocrine disrupting chemicals | 73 | ||
6.4.4 Anti-corrosive additives | 74 | ||
6.4.5 Personal care products | 74 | ||
6.4.6 Perfluorinated surfactants – PFOS and PFOA | 74 | ||
6.4.7 Pharmaceuticals | 74 | ||
6.4.8 Artificial sweeteners | 75 | ||
6.4.9 Engineered nanoparticles | 75 | ||
6.5 MANAGEMENT OPTIONS | 76 | ||
6.5.1 Technology | 76 | ||
6.5.2 Education and training | 76 | ||
6.5.3 Source separation | 77 | ||
6.5.4 Benign by design | 79 | ||
REFERENCES | 81 | ||
Chapter 7: Full costs, (dis-)economies of scale and the price of uncertainty\r | 83 | ||
7.1 INTRODUCTION | 83 | ||
7.2 CONVEYANCE-BASED WASTEWATER TREATMENT | 84 | ||
7.3 (DIS-)ECONOMIES OF SCALE | 86 | ||
7.4 DEFICITS OF THE NET PRESENT VALUE METHOD | 88 | ||
7.5 THE COST OF UNCERTAINTY | 90 | ||
7.6 ON-SITE TREATMENT SYSTEMS | 92 | ||
7.7 CONCLUSIONS | 95 | ||
REFERENCES | 96 | ||
Chapter 8: The rationale for decentralization of wastewater infrastructure\r | 99 | ||
8.1 TYPES OF WASTEWATER INFRASTRUCTURE | 99 | ||
8.2 CENTRALIZED TREATMENT SYSTEMS | 100 | ||
8.3 DISTRIBUTED CENTRALIZED SYSTEMS | 100 | ||
8.4 CENTRALIZED SYSTEMS WITH SATELLITES | 102 | ||
8.4.1 Implementation of satellite systems | 106 | ||
8.5 DECENTRALIZED SYSTEMS | 106 | ||
8.5.1 Types of decentralized wastewater systems | 106 | ||
8.5.2 Historical development of decentralized systems | 107 | ||
8.5.3 Modern development of decentralized systems | 107 | ||
8.5.4 Advantages and disadvantages of decentralization | 108 | ||
8.5.5 Continued developments in decentralized systems | 109 | ||
8.5.6 Future evolution of decentralized wastewater systems | 110 | ||
8.6 THE FUTURE | 110 | ||
8.7 SUMMARY | 112 | ||
REFERENCES | 113 | ||
Chapter 9: Cities of the global South - is decentralized sanitation a solution?\r | 114 | ||
9.1 INTRODUCTION | 114 | ||
9.2 CENTRALIZED SYSTEMS | 116 | ||
9.3 UNBUNDLING\r | 118 | ||
9.3.1 The value chain | 118 | ||
9.3.2 Vertical unbundling | 119 | ||
9.3.3 Horizontal unbundling | 120 | ||
9.4 DECENTRALIZATION | 121 | ||
9.5 TECHNOLOGIES | 121 | ||
9.6 CREATING INCENTIVES | 124 | ||
9.6.1 Contractual incentives | 124 | ||
9.6.2 Financial incentives | 125 | ||
9.6.3 Political incentives | 125 | ||
9.6.4 Professional incentives | 126 | ||
9.7 SUMMARY | 126 | ||
REFERENCES | 128 | ||
Part II: The challenges of source separation and decentralization\r | 130 | ||
Chapter 10: Implementation of source separation and decentralization in cities\r | 131 | ||
10.1 INTRODUCTION | 131 | ||
10.2 THE MAIN ADVANTAGES OF SOURCE SEPARATION AND DECENTRALIZATION IN CITIES | 133 | ||
10.3 CHALLENGES OF SOURCE SEPARATION AND DECENTRALIZATION IN CITIES | 134 | ||
10.3.1 The challenge of transport | 135 | ||
10.3.2 The challenge of developing treatment processes | 137 | ||
10.4 TRANSITION | 142 | ||
10.5 CONCLUSIONS | 144 | ||
REFERENCES | 144 | ||
Chapter 11: Hygiene, a major challenge for source separation and decentralizat\rion | 147 | ||
11.1 INTRODUCTION | 147 | ||
11.2 HAZARD IDENTIFICATION IN A SYSTEM PERSPECTIVE | 149 | ||
11.3 HUMAN EXPOSURE ASSESSMENT | 151 | ||
11.4 TREATMENT BARRIERS AND EXAMPLES OF THEIR REDUCTION EFFICIENCY | 152 | ||
11.5 QUANTIFICATIONS OF RISKS AND RISK-BENEFIT STRATEGIES | 154 | ||
11.6 FUTURE CHALLENGES AND KNOWLEDGE GAPS | 155 | ||
REFERENCES | 155 | ||
Chapter 12: Closing the loop: Recycling nutrients to agriculture\r | 158 | ||
12.1 NUTRIENT BALANCE CLOSE TO CROP REMOVAL | 158 | ||
12.2 SOURCE-SEPARATED TOILET WASTES ARE UNIQUE BIOLOGICAL FERTILIZERS | 162 | ||
12.3 NUTRIENT REQUIREMENTS AND FERTILIZERS USED IN PRACTICE | 162 | ||
12.4 ECONOMIC AND GWP VALUE OF NUTRIENTS | 165 | ||
12.5 URINE IS VERY LOW IN POLLUTANTS | 166 | ||
12.6 LOW HYGIENE RISK | 167 | ||
12.7 SPREADING MACHINERY | 168 | ||
12.8 THE FARMER – BUSINESSMAN, SOIL STEWARD AND ENTREPRENEUR | 170 | ||
REFERENCES | 171 | ||
Chapter 13: The potential of control and monitoring | 174 | ||
13.1 INTRODUCTION | 174 | ||
13.1.1 Instrumentation, control and automation aspects | 176 | ||
13.2 THE INFLUENT | 177 | ||
13.3 TREATMENT TECHNOLOGIES | 178 | ||
13.4 INSTRUMENTATION | 179 | ||
13.5 MONITORING | 180 | ||
13.6 ACTUATORS | 181 | ||
13.7 OPERATING COMPETENCE | 181 | ||
13.8 THE NEED FOR STANDARDIZATION | 182 | ||
13.9 CONCLUSIONS | 184 | ||
REFERENCES | 185 | ||
Chapter 14: High acceptance of sourceseparating technologies - but…\r | 188 | ||
14.1 INTRODUCTION | 188 | ||
14.2 SOCIAL SCIENCE METHODS | 189 | ||
14.2.1 Quantitative questionnaire surveys | 190 | ||
14.2.2 Qualitative methods | 192 | ||
14.3 ACCEPTANCE OF NOMIX TECHNOLOGY\r | 193 | ||
14.3.1 Some results from qualitative approaches | 193 | ||
14.3.2 Results from quantitative approaches | 195 | ||
14.4 ACCEPTANCE OF URINE-BASED FERTILIZERS | 197 | ||
14.5 TECHNOLOGY REQUIREMENTS AND OUTLOOK\r | 198 | ||
14.5.1 Drawbacks of NoMix toilets for users | 198 | ||
14.6 CONCLUSIONS | 199 | ||
REFERENCES | 200 | ||
Chapter 15: Market success of on-site treatment: a systemic innovation problem\r | 203 | ||
15.1 INTRODUCTION | 203 | ||
15.2 THE SYSTEMIC INNOVATION PROBLEM | 204 | ||
15.3 THE GERMAN ON-SITE INDUSTRY | 207 | ||
15.4 MAJOR INNOVATION CHALLENGES | 209 | ||
15.5 THREE POTENTIAL TRAJECTORIES | 211 | ||
15.6 CONCLUSIONS | 214 | ||
REFERENCES | 216 | ||
Part III: Potential technologies for source separation\r | 218 | ||
Chapter 16: Conceptualizing sanitation systems to account for new complexities in processing and management\r | 219 | ||
16.1 INTRODUCTION | 219 | ||
16.2 EMERGING PRODUCTS | 219 | ||
16.3 FUNCTIONAL GROUPS FOR TARGETED PRODUCT PROCESSING | 221 | ||
16.3.1 User interface | 222 | ||
16.3.2 Collection and storage | 222 | ||
16.3.3 Conveyance | 223 | ||
16.3.4 Treatment | 223 | ||
16.3.5 Use and disposal | 224 | ||
16.4 OPERATION AND MANAGEMENT: IMPLICATIONS FOR SYSTEM BOUNDARIES | 226 | ||
16.4.1 User interface | 226 | ||
16.4.2 Collection and storage | 226 | ||
16.4.3 Conveyance | 227 | ||
16.4.4 Treatment | 229 | ||
16.4.5 Use and disposal | 229 | ||
16.5 CONCLUSIONS AND RECOMMENDATIONS | 230 | ||
REFERENCES | 230 | ||
Chapter 17: Wastewater composition\r | 232 | ||
17.1 INTRODUCTION | 232 | ||
17.2 DOMESTIC WASTEWATER FLOWS | 232 | ||
17.3 WASTEWATER FLOW PATTERNS | 235 | ||
17.4 BLACKWATER | 237 | ||
17.4.1 Yellowwater | 237 | ||
17.4.2 Brownwater | 239 | ||
17.5 GREYWATER | 240 | ||
17.6 PROPORTIONAL CONTRIBUTION OF NUTRIENTS AND ORGANICS | 244 | ||
17.7 DISCUSSION AND SIGNIFICANCE | 245 | ||
REFERENCES | 245 | ||
Chapter 18: Treatment of the solid fraction\r | 249 | ||
18.1 INTRODUCTION | 249 | ||
18.2 COMPOSITION OF FAECAL SOLIDS | 249 | ||
18.3 TREATMENT GOALS | 250 | ||
18.4 COMPOSTING\r | 251 | ||
18.4.1 Process description\r | 251 | ||
18.4.2 Stage of development | 252 | ||
18.4.3 Operational requirements | 252 | ||
18.4.4 Environmental and health concerns | 253 | ||
18.4.5 Configurations | 253 | ||
18.5 VERMICOMPOSTING\r | 254 | ||
18.5.1 Process description | 254 | ||
18.5.2 Stage of development | 254 | ||
18.5.3 Operational requirements | 255 | ||
18.5.4 Environmental and health concerns | 255 | ||
18.5.5 Configurations | 256 | ||
18.6 TERRA PRETA SANITATION\r | 256 | ||
18.6.1 Process description and stage of development | 256 | ||
18.6.2 Operational requirements | 257 | ||
18.6.3 Environmental and health concerns | 257 | ||
18.6.4 Configuration | 257 | ||
18.7 DEHYDRATION\r | 258 | ||
18.7.1 Process description | 258 | ||
18.7.2 Stage of development | 258 | ||
18.7.3 Operational requirements | 258 | ||
18.7.4 Environmental and health concerns | 259 | ||
18.7.5 Configurations | 259 | ||
18.8 PASTEURIZATION | 259 | ||
18.9 CONCLUSIONS AND OUTLOOK | 260 | ||
REFERENCES | 260 | ||
Chapter 19: Aerobic elimination of organics and pathogens: greywater treatment\r | 264 | ||
19.1 INTRODUCTION | 264 | ||
19.2 COMPOSITION AND TREATABILITY\r | 265 | ||
19.2.1 Organic compounds | 265 | ||
19.2.2 Xenobiotics | 267 | ||
19.2.3 Pathogens | 268 | ||
19.3 TECHNOLOGIES FOR AEROBIC TREATMENT | 269 | ||
19.3.1 Removal of organic compounds | 270 | ||
19.3.1.1 Intensive biological processes | 270 | ||
19.3.1.2 Extensive biological processes | 271 | ||
19.3.1.3 Most effective processes | 271 | ||
19.3.2 Xenobiotics removal | 272 | ||
19.3.3 Pathogen removal | 274 | ||
19.4 CONCLUSIONS | 275 | ||
REFERENCES | 276 | ||
Chapter 20: Biological nitrogen conversion processes\r | 279 | ||
20.1 INTRODUCTION | 279 | ||
20.2 BIOLOGICAL NITROGEN CONVERSION\r | 279 | ||
20.2.1 Nitrogen uptake\r | 279 | ||
20.2.2 Nitrification | 280 | ||
20.2.3 Heterotrophic denitrification | 281 | ||
20.2.4 Anaerobic ammonium oxidation (Anammox) | 282 | ||
20.3 NITROGEN STABILIZATION IN URINE\r | 283 | ||
20.3.1 Conditions in stored urine | 283 | ||
20.3.2 Nitrification without base dosage | 283 | ||
20.3.3 Complete ammonia oxidation with base dosage | 284 | ||
20.3.4 Use of nitrified urine | 285 | ||
20.4 NITROGEN REMOVAL FROM URINE | 285 | ||
20.4.1 Nitritation/anammox in a two-reactor set-up | 286 | ||
20.4.2 Nitritation/anammox in a single reactor | 286 | ||
20.5 NITROGEN REMOVAL FROM BLACKWATER\r | 287 | ||
20.5.1 Blackwater collected in vacuum toilets | 287 | ||
20.5.2 Conventionally collected blackwater | 289 | ||
20.6 CONCLUSIONS | 290 | ||
REFERENCES | 290 | ||
Chapter 21: Anaerobic treatment of sourceseparated domestic wastewater\r | 294 | ||
21.1 INTRODUCTION | 294 | ||
21.2 THE ANAEROBIC CONVERSION PROCESS | 295 | ||
21.3 REACTOR TYPES | 295 | ||
21.3.1 Reactors without enhanced biomass retention | 295 | ||
21.3.1.1 Continuous Stirred-Tank Reactor (CSTR) | 295 | ||
21.3.1.2 Accumulation (AC) system | 297 | ||
21.3.2 Reactors with enhanced biomass retention | 297 | ||
21.3.2.1 Upflow Anaerobic Sludge Blanket (UASB) | 297 | ||
21.3.2.2 UASB septic tank | 298 | ||
21.3.2.3 Anaerobic MBR | 299 | ||
21.4 ANAEROBIC TREATMENT OF BLACKAND BROWNWATER\r | 299 | ||
21.4.1 Maximum process temperature | 299 | ||
21.4.2 Removal of organic substances and methane recovery for different waste streams | 300 | ||
21.4.2.1 Vacuum-collected blackwater | 300 | ||
21.4.2.2 Conventionally collected blackwater | 301 | ||
21.4.2.3 Black waste collected with minimum water | 302 | ||
21.4.3 Boosting energy production by adding kitchen waste | 302 | ||
21.4.4 Nutrient recovery and removal | 303 | ||
21.4.5 Removal of pharmaceuticals and hormones | 303 | ||
21.5 ANAEROBIC TREATMENT OF GREYWATER | 304 | ||
21.6 CONCLUSIONS | 305 | ||
REFERENCES | 305 | ||
Chapter 22: Electrochemical systems\r | 308 | ||
22.1 INTRODUCTION | 308 | ||
22.2 ELECTROCHEMICAL OXIDATION AND REDUCTION PROCESSES | 308 | ||
22.3 OXIDATION AND REDUCTION OF POLLUTANTS | 309 | ||
22.3.1 Suitable anode materials | 309 | ||
22.3.2 Electrochemical ammonia oxidation | 309 | ||
22.3.3 Electrochemical urea degradation | 310 | ||
22.3.4 Influence of urea hydrolysis | 310 | ||
22.3.5 Removal of organic pollutants and pathogens | 311 | ||
22.4 ELECTROCHEMICAL DISSOLUTION OF METALS\r | 311 | ||
22.4.1 Electrocoagulation | 311 | ||
22.4.2 Electrochemical precipitation of phosphate from urine | 311 | ||
22.5 BIOELECTROCHEMICAL PROCESSES\r | 312 | ||
22.5.1 Electroactive bacteria | 312 | ||
22.5.2 Reactor set-ups | 313 | ||
22.5.3 Advantages and challenges | 313 | ||
22.6 USE OF BIOELECTROCHEMICAL SYSTEMS FOR WASTEWATER CONTAINING ORGANIC SOLIDS\r | 313 | ||
22.6.1 Degradation of complex organic substrates | 313 | ||
22.6.2 Combining bioelectrochemical systems with organic solids pre-treatment | 314 | ||
22.7 BIOELECTROCHEMICAL URINE TREATMENT\r | 315 | ||
22.7.1 Influence of urine composition | 315 | ||
22.7.2 Ammonium exchange | 316 | ||
22.7.3 Inhibition by ammonia | 316 | ||
22.7.4 Sulfate removal | 316 | ||
22.7.5 Micropollutant removal | 317 | ||
22.8 ENERGY CONVERSION AND DEGRADATION RATES | 317 | ||
22.9 CONCLUSIONS | 319 | ||
REFERENCES | 319 | ||
Chapter 23: Transfer into the gas phase: ammonia stripping\r | 323 | ||
23.1 INTRODUCTION | 323 | ||
23.2 WASTEWATERS WITH HIGH AMMONIA CONTENT | 323 | ||
23.3 CHEMICAL EQUILIBRIA | 325 | ||
23.3.1 Acid-base equilibrium | 325 | ||
23.3.2 Gas exchange equilibrium | 327 | ||
23.4 AMMONIA STRIPPING WITH AIR | 328 | ||
23.5 AMMONIA STRIPPING WITH AIR AND CARBON DIOXIDE PRE-STRIPPING | 330 | ||
23.6 AMMONIA STRIPPING FROM STORED URINE | 331 | ||
23.6.1 Stripping reactors with adsorption in acid | 331 | ||
23.6.2 Steam stripping | 332 | ||
23.6.3 Passive ammonia stripping in urine-collecting systems | 333 | ||
23.7 PRODUCTS OF AMMONIA STRIPPING | 334 | ||
23.8 CONCLUSIONS | 335 | ||
REFERENCES | 335 | ||
Chapter 24: Transfer into the solid phase\r | 337 | ||
24.1 INTRODUCTION | 337 | ||
24.2 STRUVITE PRECIPITATION | 337 | ||
24.2.1 Conditions for struvite formation | 338 | ||
24.2.2 Magnesium sources | 338 | ||
24.2.3 Struvite precipitation in urine | 339 | ||
24.2.4 Struvite precipitation in blackwater | 339 | ||
24.2.5 Micropollutants and pathogens | 340 | ||
24.2.6 Use of struvite | 340 | ||
24.3 OTHER PRECIPITATION PROCESSES | 341 | ||
24.4 PHOSPHATE ADSORPTION\r | 341 | ||
24.4.1 Phosphate removal with adsorption | 341 | ||
24.4.2 Applications | 341 | ||
24.4.3 Adsorbents | 342 | ||
24.4.4 Mechanisms | 342 | ||
24.4.5 Phosphorus recovery | 343 | ||
24.5 NUTRIENT REMOVAL BY ION EXCHANGE | 343 | ||
24.5.1 Operational conditions | 343 | ||
24.5.2 Ion exchange in source-separated urine | 344 | ||
24.5.3 Amount of zeolite | 345 | ||
24.5.4 Blackwater and greywater | 345 | ||
24.5.5 Use of zeolites as fertilizer carrier | 345 | ||
24.6 CONCENTRATION PROCESSES | 346 | ||
24.6.1 Freeze and thaw | 346 | ||
24.6.2 Electrodialysis | 346 | ||
24.6.3 Reverse osmosis | 346 | ||
24.6.4 Distillation | 347 | ||
24.6.5 Energy demand | 347 | ||
24.7 CONCLUDING REMARKS | 347 | ||
REFERENCES | 348 | ||
Chapter 25: Membrane processes\r | 352 | ||
25.1 INTRODUCTION | 352 | ||
25.2 BASIC FEATURES OF MEMBRANE SYSTEMS | 353 | ||
25.2.1 Precautions for decentralized systems | 354 | ||
25.3 DECENTRALIZED APPLICATIONS\r | 354 | ||
25.3.1 Introduction | 354 | ||
25.3.2 Greywater | 354 | ||
25.3.2.1 Performance | 355 | ||
25.3.2.2 Challenges and limitations | 355 | ||
25.3.3 Blackwater | 356 | ||
25.3.3.1 Performance | 356 | ||
25.3.3.2 Challenges and limitations | 358 | ||
25.3.4 Source-separated urine | 359 | ||
25.3.4.1 Performance | 359 | ||
25.3.4.2 Challenges and limitations | 360 | ||
25.3.5 Combined wastewater | 360 | ||
25.3.5.1 Performance | 361 | ||
25.3.5.2 Challenges and limitations | 362 | ||
25.4 INDUSTRY TRENDS | 362 | ||
25.4.1 Accounting for the cost of carbon | 362 | ||
25.4.2 Increased monitoring and regulation of trace contaminants | 364 | ||
25.4.3 Application of risk management procedures | 364 | ||
25.5 CONCLUSIONS | 364 | ||
REFERENCES | 365 | ||
Chapter 26: Chemical oxidation processes\r | 367 | ||
26.1 INTRODUCTION | 367 | ||
26.1.1 Common chemical oxidants | 367 | ||
26.1.2 Oxidation/disinfection by-products | 368 | ||
26.1.3 Kinetics of oxidation/disinfection processes | 369 | ||
26.1.4 Transformation products and their biological activity | 372 | ||
26.2 APPLICATION OF OXIDATION PROCESSES TO SOURCE-SEPARATED WASTE STREAMS\r | 373 | ||
26.2.1 General considerations | 373 | ||
26.2.2 Efficiency of oxidation/disinfection processes: role of water matrix components | 373 | ||
26.2.3 Efficiency of oxidation/disinfection with ozone: the role of DOC concentration | 374 | ||
26.2.4 Application of oxidation/disinfection processes to source-separated urine | 376 | ||
26.2.5 Application of oxidation/disinfection processes to greywater | 376 | ||
26.2.6 Application of oxidation/disinfection processes to effluents of biological reactors | 376 | ||
26.3 CONCLUSIONS | 377 | ||
REFERENCES | 377 | ||
Chapter 27: Enhanced fractionation of mixed wastewater as an alternative to separation at the source\r | 382 | ||
27.1 INTRODUCTION | 382 | ||
27.2 MOTIVES FOR PRE-CONCENTRATION | 383 | ||
27.3 APPROACHES FOR SMALL COMMUNITIES\r | 384 | ||
27.3.1 Fractionation of mixed wastewater | 384 | ||
27.3.1.1 Chemically enhanced primary treatment (CEPT) \rand sedimentation | 385 | ||
27.3.1.2 Dissolved air flotation | 385 | ||
27.3.1.3 Rapidly activated sludge bioflocculation and sedimentation | 386 | ||
27.3.2 Post-treatment options for water reuse | 387 | ||
27.3.3 The potential for energy recovery | 388 | ||
27.3.4 The potential for nutrient recovery | 388 | ||
27.4 APPROACHES AT HOUSEHOLD LEVEL | 389 | ||
27.5 THE FUTURE OF MEMBRANE FILTRATION | 390 | ||
27.6 SUMMARY | 391 | ||
ACKNOWLEDGEMENT | 392 | ||
REFERENCES | 392 | ||
Part IV: The international experience\r | 395 | ||
Chapter 28: The Swedish experience with source separation\r | 396 | ||
28.1 THE EARLY 1990s – INTRODUCTION OF UD | 396 | ||
28.2 LATE 1990s TO PRESENT – ON-SITE SANITATION | 397 | ||
28.3 THREE TYPICAL SWEDISH CASES STUDIES\r | 400 | ||
28.3.1 Single household with local reuse of urine and faeces\r | 400 | ||
28.3.2 Local blackwater system for eco-fertilizer production | 400 | ||
28.3.3 Municipality demanding source separation (Tanum) | 401 | ||
28.4 CONCLUSIONS | 402 | ||
REFERENCES | 402 | ||
Chapter 29: Practical experience with source separation in Germany\r | 404 | ||
29.1 BACKGROUND | 404 | ||
29.2 THE DWA STORY | 404 | ||
29.3 THE PILOT PLANT STORY | 405 | ||
29.3.1 Pilot projects with anaerobic digestion of blackwater | 405 | ||
29.3.2 Pilot projects with urine source separation | 408 | ||
29.4 NECESSITY FOR TECHNICAL IMPROVEMENTS AND SCIENTIFIC RESEARCH | 409 | ||
29.5 PERSPECTIVE | 410 | ||
REFERENCES | 410 | ||
Chapter 30: The Netherlands: “Nieuwe Sanitatie”\r | 412 | ||
30.1 INTRODUCTION | 412 | ||
30.2 “NEW SANITATION” IN THE NETHERLANDS | 412 | ||
30.3 FROM RESEARCH TO IMPLEMENTATION | 415 | ||
REFERENCES | 418 | ||
Chapter 31: Source control and source separation: the Swiss experience\r | 420 | ||
31.1 INTRODUCTION | 420 | ||
31.2 DRIVERS FOR CHANGE IN SWITZERLAND | 420 | ||
31.3 CASE STUDIES IN SWITZERLAND | 422 | ||
31.3.1 On-site wastewater treatment and reuse | 422 | ||
31.3.1.1 Cableway station Zermatt | 422 | ||
31.3.1.2 Aquamin detached house | 423 | ||
31.3.2 Urine separation and processing | 424 | ||
31.3.2.1 Office building Forum Chriesbach at Eawag | 424 | ||
31.3.2.2 Urine processing at the Liestal public library | 424 | ||
31.3.3 Energy and water autarky | 425 | ||
31.3.3.1 High alpine resort Monte Rosa | 425 | ||
31.3.3.2 Self-sufficient housing “Self” (www.empa.ch/self) | 425 | ||
31.4 WHAT DID WE LEARN? | 425 | ||
REFERENCES | 426 | ||
Chapter 32: Development of decentralized systems in Australia\r | 428 | ||
32.1 INTRODUCTION | 428 | ||
32.2 DRIVERS FOR DECENTRALIZATION | 428 | ||
32.3 OVERVIEW OF DECENTRALIZED SYSTEMS | 429 | ||
32.3.1 Cluster Scale Developments | 429 | ||
32.3.2 Urine-Separating Toilets | 433 | ||
32.4 CONCLUSIONS | 433 | ||
REFERENCES | 434 | ||
Chapter 33: Source separation in middleand low-income countries\r | 436 | ||
33.1 INTRODUCTION | 436 | ||
33.2 DRIVERS FOR SOURCE SEPARATION IN MIDDLEAND LOW-INCOME COUNTRIES | 437 | ||
33.3 EXAMPLES OF SCALED-UP DISSEMINATION OF SOURCE SEPARATION IN AFRICA AND ASIA | 438 | ||
33.3.1 Scaling-up urine diverting toilets in peri-urban areas of eThekwini, South Africa | 438 | ||
33.3.1.1 Costs and economics | 439 | ||
33.3.2 Community-led water and environmental sanitation improvement in Shaanxi, China | 440 | ||
33.3.2.1 Costs and economics | 440 | ||
33.3.2.2 Evaluation | 441 | ||
33.4 CONCLUSION AND OUTLOOK | 442 | ||
REFERENCES | 442 | ||
Part V: The paradigm shift | 444 | ||
Chapter 34: Why question the prevailing paradigm of wastewater management?\r | 445 | ||
34.1 INTRODUCTION | 445 | ||
34.2 IMAGINING THE CITY AS A FORCE FOR GOOD IN ITS ENVIRONMENT | 447 | ||
34.3 SOURCE SEPARATION AND DECENTRALIZATION | 448 | ||
34.4 SOCIAL SCIENCE AND ECONOMICS | 450 | ||
34.5 CONCLUSIONS | 451 | ||
REFERENCES | 452 | ||
Chapter 35: How to spur innovation?\r | 454 | ||
35.1 WHY INNOVATION? | 454 | ||
35.2 THEORY OF INNOVATION | 456 | ||
35.3 SUSTAINABLE INNOVATION | 457 | ||
35.4 SUSTAINABLE WASTEWATER MANAGEMENT | 458 | ||
35.5 SPURING SUSTAINABLE INNOVATION | 461 | ||
REFERENCES | 461 | ||
Index | 463 |