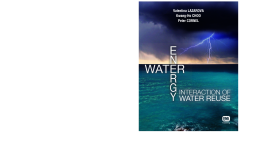
BOOK
Water - Energy Interactions in Water Reuse
Valentina Lazarova | Kwang-Ho Choo | Peter Cornel
(2012)
Additional Information
Book Details
Abstract
The focus of Water-Energy Interactions in Water Reuse is to collect original contributions and some relevant publications from recent conference proceedings in order to provide state-of-art information on the use of energy in wastewater treatment and reuse systems. Special focus is given to innovative technologies, such as membrane bioreactors, high pressure membrane filtration systems, and novel water reuse processes. A comparison of energy consumption in water reuse systems and desalination will be also provided.
Water-Energy Interactions in Water Reuse covers the use of energy in conventional and advanced wastewater treatment for various water reuse applications, including carbon footprint, energy efficiency, energy self-sufficient facilities and novel technologies, such as microbial fuel cells and biogas valorisation. It is of real value to water utility managers; policy makers for water and wastewater treatment; water resources planners, and researchers and students in environmental engineering and science.
Editors: Valentina Lazarova, Suez Environnement, France, Kwang-Ho Choo, Kyungpook National University, Korea, Peter Cornel, Technical University of Darmstadt, Germany
Table of Contents
Section Title | Page | Action | Price |
---|---|---|---|
Cover page | 1 | ||
Half title page | 2 | ||
Title page | 3 | ||
Copyright Page | 4 | ||
Contents | 5 | ||
List of contributors | 15 | ||
Foreword | 22 | ||
Introduction | 24 | ||
Part 1 | 26 | ||
The Water-Energy Nexus | 26 | ||
FOREWORD | 26 | ||
Chapter 1 | 28 | ||
Designing and implementing urban water and resource management systems which recover water, energy, and nutrients | 28 | ||
1.1 INTRODUCTION | 28 | ||
1.2 EVOLVING URBAN WATER AND RESOURCE MANAGEMENT SYSTEM REQUIREMENTS | 28 | ||
1.2.1 The necessity for change | 28 | ||
1.2.2 Contrasting historical and future approaches | 32 | ||
1.3 COMPONENTS OF EVOLVING URBAN WATER AND RESOURCE MANAGEMENT SYSTEMS | 33 | ||
1.3.1 Water management | 33 | ||
1.3.2 Energy management | 34 | ||
1.3.4 Nutrient management | 35 | ||
1.4 INTEGRATED SYSTEMS | 36 | ||
1.4.1 System approaches | 36 | ||
1.4.2 An example system | 38 | ||
1.4.3 Developing and analyzing integrated urban water and resource management systems | 40 | ||
1.5 IMPLEMENTING INTEGRATED URBAN WATER AND RESOURCE MANAGEMENT SYSTEMS | 42 | ||
REFERENCES | 43 | ||
Chapter 2 | 45 | ||
Energy and water: relations and recovery potential | 45 | ||
2.1 ENERGY AND WATER – AN INTRINSICALLY BOUND COUPLE | 45 | ||
2.1.1 Energy for water | 45 | ||
2.1.2 Energy from water | 46 | ||
2.1.2.1 Kinetic energy | 47 | ||
2.1.2.2 Potential energy | 47 | ||
2.1.2.3 Thermal energy | 47 | ||
2.1.2.4 Chemically bound energy | 47 | ||
2.1.3 Water for energy | 48 | ||
2.2 ENERGY AND WATER RECOVERY | 49 | ||
2.2.1 Water demand | 49 | ||
2.2.2 Recovery by water reuse | 50 | ||
2.2.3 Possibilities of energy recovery from (waste)water | 51 | ||
2.2.3.1 Potential energy | 51 | ||
2.2.3.2 Thermal energy | 51 | ||
2.2.3.3 Chemically bound energy | 51 | ||
2.3 POTENTIAL OF WATER REUSE | 52 | ||
2.3.1 Reuse options | 53 | ||
2.3.2 Water quality requirements | 54 | ||
2.3.3 Treatment options | 55 | ||
2.3.4 Energy requirements | 56 | ||
2.4 CONCLUSIONS | 57 | ||
REFERENCES | 57 | ||
Chapter 3 | 60 | ||
Water and energy link in the cities of thefuture – achieving net zero carbon andpollution emissions footprint | 60 | ||
3.1 INTRODUCTION | 60 | ||
3.2 URBAN METABOLISM | 60 | ||
3.2.1 Urban metabolism – reclaim, reuse and recycle | 60 | ||
3.2.2 Water footprint – direct use of water | 61 | ||
3.2.3 Water-energy nexus | 61 | ||
3.2.4 Distributed (hybrid) vs. centralized (linear) systems | 65 | ||
3.2.5 A double loop hybrid system – source separation | 66 | ||
3.2.6 Energy (CO2) balance for a city switching to sustainable water management | 68 | ||
3.3 INTEGRATED RESOURCE RECOVERY FACILITY (IRRF) | 70 | ||
3.3.1 A concept of a future IRRF | 71 | ||
3.4 COMPARISON OF ALTERNATIVES | 76 | ||
3.4.1 Description of three alternatives | 76 | ||
3.4.2 Discussion of the alternatives | 78 | ||
3.5 CONCLUSIONS | 79 | ||
REFERENCES | 80 | ||
Chapter 4 | 83 | ||
Embodied energy in the water cycle | 83 | ||
4.1 INTRODUCTION | 83 | ||
4.2 ENERGY CONTENT OF WASTEWATER | 83 | ||
4.3 EMBODIED ENERGY IN THE WATER CYCLE | 84 | ||
4.4 WATER QUALITY AND ENERGY INTENSITY | 85 | ||
4.5 WASTEWATER RECLAMATION AS PRODUCT | 86 | ||
4.6 SUSTAINABILITY PRINCIPLE IN WASTEWATER RECLAMATION | 89 | ||
4.7 LIFE CYCLE ANALYSIS OF WATER REUSE | 91 | ||
4.8 CARBON FOOTPRINT AND GREENHOUSE GASES | 92 | ||
4.9 ENERGY OPTIMIZATION OF SYSTEMS | 92 | ||
4.10 WATER RECYCLING PURPLE PIPE INFRASTRUCTURE | 94 | ||
4.11 SUMMARY | 94 | ||
REFERENCES | 94 | ||
Chapter 5 | 96 | ||
Microbial electrochemical technologies for energy sustainability of the water infrastructure | 96 | ||
5.1 INTRODUCTION | 96 | ||
5.2 THE WATER-ENERGY NEXUS | 96 | ||
5.3 MICROBIAL FUEL CELL BASED TECHNOLOGIES FOR ENERGY RECOVERY | 98 | ||
5.3.1 Microbial fuel cells for bioelectricity generation | 98 | ||
5.3.2 Microbial electrolysis cells for hydrogen or methane gas production | 100 | ||
5.3.3 Microbial desalination cells | 102 | ||
5.3.4 New MFC-based technologies | 103 | ||
5.4 CONCLUSIONS AND OUTLOOK | 103 | ||
Acknowledgement | 104 | ||
REFERENCES | 104 | ||
Part 2 | 106 | ||
Energy Footprint of Wastewater Treatment | 106 | ||
FOREWORD | 106 | ||
Chapter 6 | 108 | ||
Toward energy self-sufficiency of wastewater treatment | 108 | ||
6.1 INTRODUCTION | 108 | ||
6.1.1 Energy consumption of wastewater treatment plants | 109 | ||
6.1.2 Energy recovery by anaerobic digestion | 112 | ||
6.1.3 The concept of positive energy plant | 113 | ||
6.2 ENERGY SAVING | 115 | ||
6.2.1 Major components of energy consumption of wastewater treatment plants | 115 | ||
6.2.2 Improvement of aeration system’s efficiency | 116 | ||
6.2.2.1 Optimisation of air production | 117 | ||
6.2.2.2 Optimisation of air diffusion | 120 | ||
6.2.2.3 Optimisation of pollution removal | 120 | ||
6.2.2.4 Aeration control strategy | 120 | ||
6.2.3 Treatment of high ammonium concentration effluents from anaerobic sludge digestion | 122 | ||
6.3 ENERGY RECOVERY FROM SEWAGE FLOWS | 122 | ||
6.3.1 Hydro electricity | 122 | ||
6.3.2 Heat exchange from sewage flows | 123 | ||
6.4 ENERGY FROM SLUDGE | 124 | ||
6.4.1 Energy from solid fuels derived from sewage sludge | 126 | ||
6.4.3 Energy from biogas derived from sewage sludge | 127 | ||
6.4.3.1 Biogas production by anaerobic digestion | 127 | ||
6.4.3.2 Improvement of biogas production from anaerobic digestion | 127 | ||
6.4.3.3 Biogas quality enhancement and end uses | 131 | ||
6.4.3.3 Gasification and pyrolysis | 133 | ||
6.4.4 Low temperature heat to electricity | 134 | ||
6.5 PRODUCTION OF RENEWABLE ENERGY | 135 | ||
6.5.1 Solar energy | 135 | ||
6.5.2 Wind energy | 137 | ||
6.6 EXAMPLES OF ENERGY SELF-SUFFICIENT WASTEWATER TREATMENT PLANTS | 138 | ||
6.7 CONCLUSIONS | 141 | ||
Acknowledgement | 142 | ||
REFERENCES | 143 | ||
Chapter 7 | 148 | ||
Performance improvement of full scale membrane bioreactors | 148 | ||
7.1 INTRODUCTION | 148 | ||
7.2 METHODOLOGY | 148 | ||
7.3 OPTIMISATION OF MBR PERFORMANCE | 151 | ||
7.3.1 Nitrogen removal | 151 | ||
7.3.2 Energy consumption | 153 | ||
7.4 IMPROVEMENT OF MBR ENERGY EFFICIENCY | 156 | ||
7.4.1 A new operating paradigm | 156 | ||
7.4.2 Practical application | 157 | ||
7.5 CONCLUSIONS | 158 | ||
REFERENCES | 158 | ||
Chapter 8 | 160 | ||
Energy optimization of large scalemembrane bioreactors – importanceof the design flux | 160 | ||
8.1 INTRODUCTION | 160 | ||
8.2 METHODOLOGY AND STUDY SITES | 160 | ||
8.2.1 Study sites | 160 | ||
8.2.2 Model WWTP | 161 | ||
8.3 MAIN RESULTS | 162 | ||
8.3.1 Hydraulic capacity utilisation | 162 | ||
8.3.2 Energy consumption | 163 | ||
8.3.3 MBR operation regimes to respond to influent flow fluctuations | 163 | ||
8.3.4 Comparison of the two filtration regimes on the basis of large scale operational data | 164 | ||
8.4 CONCLUSIONS | 167 | ||
Acknowledgements | 168 | ||
REFERENCES | 168 | ||
Chapter 9 | 170 | ||
Designing a membrane bioreactor to minimize energy use while meeting a low nitrogen limit | 170 | ||
9.1 INTRODUCTION | 170 | ||
9.1.1 Preliminary and primary unit processes | 171 | ||
9.1.2 Membrane selection | 172 | ||
9.1.3 BNR process selection | 173 | ||
9.1.4 Process aeration design | 174 | ||
9.2 AERATION CONTROL STRATEGY | 176 | ||
9.2.1 Process air control strategy | 176 | ||
9.2.2 Membrane scour air control strategy | 177 | ||
9.3 CONCLUSIONS | 178 | ||
REFERENCES | 179 | ||
Chapter 10 | 181 | ||
Finding the balance between greenhouse gas emission and energy efficiency of wastewater treatment | 181 | ||
10.1 INTRODUCTION | 181 | ||
10.1.1 Energy production at wastewater treatment plants | 181 | ||
10.1.2 Greenhouse gas emission at wastewater treatment plants | 182 | ||
10.2 SOURCES OF CH4 AND N2O EMISSIONS | 182 | ||
10.3 INITIATIVES TO ENERGY EFFICIENCY IMPROVEMENT | 184 | ||
10.3.1 Short-term measures to increase energy efficiency | 184 | ||
10.3.2 Long-term measures to increase energy efficiency | 184 | ||
10.4 GHG EMISSION DUE TO ENERGY SAVING AT WWTPS | 185 | ||
10.4.1 Effects of short-term measures on GHG emission | 185 | ||
10.4.2 Effects of long-term measures on GHG emission | 187 | ||
10.5 CONCLUSIONS | 187 | ||
REFERENCES | 188 | ||
Part 3 | 189 | ||
Energy Footprint of Water Reuse | 189 | ||
FOREWORD | 189 | ||
Chapter 11 | 191 | ||
Semizentral Germany: energy self-sufficient infrastructure systems for livable cities of the future | 191 | ||
11.1 CHALLENGES OF THE FUTURE | 191 | ||
11.1.1 New infrastructure solutions needed to cope with urban growth | 192 | ||
11.1.2 Strategies to cope with the water challenge | 193 | ||
11.2 THE SEMIZENTRAL APPROACH | 195 | ||
11.2.1 Integrated energy and material flows for improved efficiency | 195 | ||
11.2.2 A matter of scale | 196 | ||
11.2.3 A matter of flexibility: the construction kit | 197 | ||
11.2.3.1 Module A: greywater treatment | 197 | ||
11.2.3.2 Module B: blackwater treatment | 197 | ||
11.2.3.3 Module C: energy center – waste to energy | 198 | ||
11.3 THE NEXT LEVEL – NEXUS OF SCALE AND FLEXIBILITY | 199 | ||
11.4 CONCLUSIONS | 200 | ||
REFERENCES | 200 | ||
Chapter 12 | 202 | ||
Groundwater Replenishment System – energy usage implications | 202 | ||
12.1 INTRODUCTION | 202 | ||
12.2 DESIGN OF THE ADVANCED WATER PURIFICATION FACILITY | 204 | ||
12.3 WATER QUALITY | 207 | ||
12.4 ENERGY CONSUMPTION | 207 | ||
12.5 CONCLUSIONS | 210 | ||
REFERENCES | 211 | ||
Chapter 13 | 212 | ||
Comparative study of carbon footprint of water reuse | 212 | ||
13.1 INTRODUCTION | 212 | ||
13.3 DESCRIPTION OF WATER REUSE FACILITIES | 212 | ||
13.3.1 El Prat de Llobregat water reclamation plant | 213 | ||
13.3.2 Bundamba advanced water recycling plant | 214 | ||
13.3.3 Water quality requirements and uses | 215 | ||
13.4 METHODOLOGY | 216 | ||
13.4.1 Carbon footprint | 216 | ||
13.5 COMPARISON OF CARBON FOOTPRINTS | 218 | ||
13.6 CONCLUSIONS | 220 | ||
REFERENCES | 221 | ||
Chapter 14 | 222 | ||
Comparison of processes for greywater treatment for urban water reuse: energy consumption and footprint | 222 | ||
14.1 INTRODUCTION | 222 | ||
14.2 METHODOLOGY | 223 | ||
14.3 EFFICIENCY OF GREYWATER TREATMENT | 224 | ||
14.3.1 BAF | 224 | ||
14.3.2 MBR | 225 | ||
14.3.3 SBR | 226 | ||
14.4 COMPARISON OF PERFORMANCE AND ENERGY DEMAND OF GREYWATER TREATMENT TECHNOLOGIES | 227 | ||
14.5 CONCLUSIONS | 228 | ||
Acknowledgement | 228 | ||
REFERENCES | 228 | ||
Chapter 15 | 231 | ||
Sustainable approaches to water reuse systems with emphasis on energy requirements | 231 | ||
15.1 INTRODUCTION | 231 | ||
15.2 ENVIRONMENTAL SUSTAINABILITY | 232 | ||
15.3 TECHNICAL VIABILITY | 233 | ||
15.3.1 Meeting water quantity requirements | 233 | ||
15.3.2 Meeting water quality requirements | 234 | ||
15.4 SOCIAL AND ECONOMIC ASPECTS | 235 | ||
15.5 SYSTEM ANALYSIS INCLUDING ENERGY USE | 236 | ||
15.5.1 Methodology | 237 | ||
15.5.2 Analysis of water reuse options | 238 | ||
15.6 CONCLUSIONS | 239 | ||
REFERENCES | 240 | ||
Part 4 | 242 | ||
Energy Footprint of Alternative Water Resources | 242 | ||
FOREWORD | 242 | ||
Chapter 16 | 244 | ||
Energy use for seawater desalination –current status and future trends | 244 | ||
16.1 INTRODUCTION | 244 | ||
16.2 ENERGY USE FOR DESALINATION | 245 | ||
16.2.1 Current status of energy use for seawater reverse osmosis (SWRO) | 245 | ||
16.2.2 Minimum energy demand for SWRO desalination | 246 | ||
16.3 DESALINATION ENERGY USE FACTORS AND TECHNOLOGY TRENDS | 247 | ||
16.3.1 Collocation of desalination and power plants – use of warmer source water | 247 | ||
16.3.2 Using lower salinity source water to reduce energy consumption | 250 | ||
16.3.3 Higher productivity SWRO elements yield lower energy costs | 251 | ||
16.3.4 Hybrid membrane configuration reduces energy | 252 | ||
16.3.5 Split-flow RO system configuration for improved energy & cost efficiency | 254 | ||
16.3.6 Increased high pressure pump efficiency | 255 | ||
16.3.7 Improved energy recovery | 255 | ||
16.4 FUTURE DESALINATION TECHNOLOGY ADVANCES | 256 | ||
16.5 SUMMARY AND CONCLUSIONS | 258 | ||
REFERENCES | 258 | ||
Chapter 17 | 259 | ||
Water reuse versus seawater desalination –evaluation of the economic and environmental viability | 259 | ||
17.1 INTRODUCTION | 259 | ||
17.2 METHODOLOGY | 260 | ||
17.2.1 Case study characteristics | 260 | ||
17.2.2 Cross-case analysis | 260 | ||
17.2.3 Water balancing and water system modelling | 262 | ||
17.2.4 Cost estimations for selected technological options | 262 | ||
17.3 ANALYSIS OF DIFFERENT WATER MANAGEMENT SCENARIOS | 263 | ||
17.3.1 Cross-case analysis of selected case studies | 263 | ||
17.3.2 Water balance and major drivers | 264 | ||
17.3.3 Economic viability of water reuse and desalination | 266 | ||
17.3.4 Cost estimates for desalination | 266 | ||
17.3.5 Influence of the location and the cost of water transport | 267 | ||
17.3.6 Cost and energy demand of water reuse | 268 | ||
17.3.7 Environmental impact | 269 | ||
17.4 CONCLUSIONS | 270 | ||
REFERENCES | 271 | ||
Chapter 18 | 273 | ||
Desalination vs water reuse: An energyanalysis illustrated by case studies inLos Angeles and London | 273 | ||
18.1 INTRODUCTION | 273 | ||
18.2 BACKGROUND | 274 | ||
18.3 TECHNOLOGY | 274 | ||
18.4 RESOURCES | 275 | ||
18.5 ENERGY USE IN TREATMENT | 277 | ||
18.6 CASE STUDY: ORANGE COUNTY, CALIFORNIA, USA | 278 | ||
18.7 CASE STUDY: BECKTON, EAST END OF LONDON, UK | 280 | ||
18.8 ENERGY USE IN TRANSFER AND DISTRIBUTION | 281 | ||
18.9 CONCLUSIONS | 282 | ||
REFERENCES | 283 | ||
Chapter 19 | 284 | ||
Operational energy consumption and carbon dioxide emissions from rainwater harvesting systems | 284 | ||
19.1 INTRODUCTION | 284 | ||
19.2 DISINFECTION | 285 | ||
19.3 PRESSURE DRIVEN SYSTEMS | 285 | ||
19.3.1 Directly and indirectly pumped systems | 285 | ||
19.3.1.1 Fixed speed pumps | 285 | ||
19.3.1.2 Estimating RWH system energy consumption and CO2 emissions by proxy | 285 | ||
19.3.1.3 Variable speed/higher efficiency pumps | 287 | ||
19.3.1.4 Integration with renewable energy sources | 287 | ||
19.3.1.5 Integrated energy monitoring | 288 | ||
19.4 EMERGING GRAVITY SYSTEMS | 288 | ||
19.4.1 Internal building systems | 288 | ||
19.4.2 External building systems | 288 | ||
19.5 IMPROVEMENT OF ENERGY EFFICIENCY OF RWH | 291 | ||
19.5.1 Energy saving in sewers from RWH | 291 | ||
19.5.2 Energy generation from RWH | 291 | ||
19.6 CONCLUSIONS | 292 | ||
REFERENCES | 292 | ||
Chapter 20 | 294 | ||
LCA as a tool to assess environmental impact and energy efficiency of reverse osmosis desalination | 294 | ||
20.1 INTRODUCTION | 294 | ||
20.2 METHODOLOGY | 295 | ||
20.2.1 Desalination with reverse osmosis | 295 | ||
20.2.2 Application of life cycle assessment | 296 | ||
20.3 COMPARISON OF THE RESULTS OF THE LCA METHODS | 298 | ||
20.3.1 CML | 298 | ||
20.3.2 Ecoindicator 99 | 299 | ||
20.3.3 Ecopoints 97 | 299 | ||
20.3.4 Discussion | 301 | ||
20.5 CONCLUSIONS | 302 | ||
REFERENCES | 303 | ||
Part 5 | 305 | ||
Water Footprint of Energy Production | 305 | ||
FOREWORD | 305 | ||
Chapter 21 | 307 | ||
Water for energy, the use of the water footprint for the assessment of water use for bioenergy | 307 | ||
21.1 INTRODUCTION | 307 | ||
21.2 BIOENERGY | 308 | ||
21.3 THE WATER FOOTPRINT CONCEPT | 308 | ||
21.4 THE WATER FOOTPRINT OF BIO-ENERGY | 309 | ||
21.4.1 Bio-ethanol | 309 | ||
21.4.2 Biodiesel | 311 | ||
21.4.3 Heat and bio-electricity | 313 | ||
21.5 COMPARISON WITH OTHER ENERGY CARRIERS | 313 | ||
21.6 THE WATER FOOTPRINT OF NEXT - GENERATION BIOFUELS | 314 | ||
21.7 DISCUSSION | 315 | ||
21.8 CONCLUSIONS | 316 | ||
REFERENCES | 316 | ||
Chapter 22 | 319 | ||
Water demand for the production of renewable energy from crops | 319 | ||
22.1 INTRODUCTION | 319 | ||
22.2 BIO-ENERGY GENERATION | 319 | ||
22.2.1 General considerations | 319 | ||
22.2.2 Current state of bio-energy production | 320 | ||
22.3 WATER DEMAND | 320 | ||
22.3.1 Water demand of energy crops | 320 | ||
22.3.2 Water demand for the substrate production of biogas plants | 322 | ||
22.3.3 Biogenous fuels | 324 | ||
22.4 CONCLUSIONS | 326 | ||
REFERENCES | 327 | ||
Chapter 23 | 329 | ||
Summary and concluding remarks – solving the water-energy nexus for tomorrow | 329 | ||
23.1 INTRODUCTION | 329 | ||
23.2 TAKING ADVANTAGE OF THE WATER-ENERGY NEXUS | 331 | ||
23.3 LOWERING THE ENERGY AND CARBON FOOTPRINT OF WASTEWATER TREATMENT | 332 | ||
23.4 ENERGY FOOTPRINT OF WATER REUSE | 333 | ||
23.5 COMBINING ENERGY-EFFICIENT REUSE WITH ALTERNATIVE WATER RESOURCES | 334 | ||
23.6 TAKING INTO ACCOUNT THE WATER FOOTPRINT OF ENERGY PRODUCTION | 335 | ||
23.7 CONCLUSIONS | 336 | ||
Index | 337 |