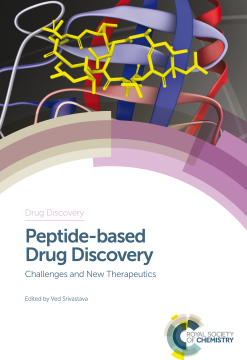
Additional Information
Book Details
Abstract
With potentially high specificity and low toxicity, biologicals offer promising alternatives to small-molecule drugs. Peptide therapeutics have again become the focus of innovative drug development efforts backed up by a resurgence of venture funds and small biotechnology companies.
What does it take to develop a peptide-based medicine? What are the key challenges and how are they overcome? What are emerging therapeutics for peptide modalities? This book answers these questions with a holistic story from molecules to medicine, combining the themes of design, synthesis and clinical applications of peptide-based therapeutics and biomarkers. Chapters are written and edited by leaders in the field from industry and academia and they cover the pharmacokinetics of peptide therapeutics, attributes necessary for commercially successful metabolic peptides, medicinal chemistry strategies for the design of peptidase-resistant peptide analogues, disease classes for which peptide therapeutic are most relevant, and regulatory issues and guidelines.
The critical themes covered provide essential background information on what it takes to develop peptide-based medicine from a chemistry perspective and views on the future of peptide drugs. This book will be a valuable resource not only as a reference book for the researcher engaged in academic and pharmaceutical setting, from basic research to manufacturing and from organic chemistry to biotechnology, but also a valuable resource to graduate students to understand discovery and development process for peptide-based medicine.
Ved Srivastava is Vice President of Chemistry and co-founder at Phoundry Pharmaceuticals, and prior to that he was head of Peptide Chemistry at GlaxoSmithKline and Vice President of research and production at New England Peptide. He spent several years with Amylin Pharmaceuticals where he focused on discovery and development of novel peptide hormones for diabetes, obesity and neuropsychiatric therapies. He has significantly contributed in the development of ByettaTM, SymlinTM and BydeureonTM for the treatment of diabetes. He also directed the research chemistry team of Psylin Neurosciences, Inc., a biotech company formed as a joint venture between Amylin Pharmaceuticals, Inc. and PshychoGenics, Inc. Ved has over 25 years of experience with expertise in drug discovery in the area metabolic diseases and inflammation with major emphasis in peptide medicinal chemistry and peptide delivery. He earned a Ph.D.in organic chemistry from the University of Lucknow, India and had subsequent postdoctoral appointments at the University of Georgia and the University of Colorado Medical School. Ved is energetically involved in the governance of the American Peptide Society.
Table of Contents
Section Title | Page | Action | Price |
---|---|---|---|
Cover | Cover | ||
Peptide-based Drug Discovery: Challenges and New Therapeutics | i | ||
Foreword 1 | vii | ||
Foreword 2 | ix | ||
Preface | xi | ||
Biography | xv | ||
Contents | xvii | ||
Chapter 1 - Renaissance in Peptide Drug Discovery: The Third Wave | 1 | ||
1.1 Peptide Science and Technology | 1 | ||
1.1.1 Past Milestones in Peptide Science and Technology | 2 | ||
1.1.2 Hierarchical Strategies to Transform Native Peptides into Drug Candidates | 5 | ||
1.2 Peptide Target Space and Druggability | 6 | ||
1.2.1 G Protein-Coupled Receptors: Class A and Class B | 6 | ||
1.2.1.1 Melanocortin Receptor Agonists/Antagonists | 7 | ||
1.2.1.2 GLP-1 Receptor Agonists/Antagonists | 8 | ||
1.2.2 Intracellular Protein–Protein Interaction Targets | 9 | ||
1.2.3 Exploring Peptide–Target Molecular Recognition | 10 | ||
1.3 Peptide Drug Design and Chemical Space | 11 | ||
1.3.1 Peptide ψ, ϕ and χ Space | 12 | ||
1.3.2 Peptide Backbone Modifications | 13 | ||
1.3.3 Peptide Secondary Structure Mimicry | 13 | ||
1.3.3.1 β- and γ-turn Peptidomimetics and Non-Peptide Templates | 14 | ||
1.3.3.2 β-strand Peptidomimetic and Non-Peptide Templates | 14 | ||
1.3.3.3 α-Helix Peptidomimetic and Non-Peptide Templates | 14 | ||
1.3.4 Peptide Macrocyclization Design and Diversity | 15 | ||
1.3.4.1 Stapled α-Helical Peptides | 16 | ||
1.3.4.2 Macrocyclic Peptides from Super-Diverse Libraries | 18 | ||
1.4 Peptide Cell Permeability and Drug Delivery | 19 | ||
1.5 Peptide Breakthrough Medicine and Disruptive Innovation | 22 | ||
Acknowledgements | 23 | ||
References | 23 | ||
Chapter 2 - Identification and Validation of Peptide Therapeutic Targets and Indications | 35 | ||
2.1 Introduction | 35 | ||
2.1.1 Background | 35 | ||
2.1.2 Advantages of Peptide Therapeutics | 36 | ||
2.1.2.1 Efficacy | 36 | ||
2.1.2.2 Safety | 37 | ||
2.1.3 Marketed Drugs | 38 | ||
2.1.4 Bioactive Peptides | 38 | ||
2.1.5 Drug Targets | 39 | ||
2.1.6 Peptide Targets | 39 | ||
2.1.6.1 G Protein-Coupled Receptors | 39 | ||
2.1.6.2 Catalytic Receptors | 42 | ||
2.1.7 Peptide Drugs | 43 | ||
2.2 Discovery of Utilities | 43 | ||
2.2.1 Background | 43 | ||
2.2.2 Discovery from Knowledge of Normal and Pathologic Physiology | 45 | ||
2.2.2.1 Inference of Evolved Purpose | 45 | ||
2.2.2.2 Inference of Function from Anatomy | 50 | ||
2.2.2.3 Deficiency States | 52 | ||
2.2.2.4 Inference from Gene Knockout and Other Techniques | 53 | ||
2.2.2.5 Functional Ablation via Antagonism/Neutralization | 55 | ||
2.2.2.6 Models Derived from Screening of Disease | 56 | ||
2.3 Biological Assays in Peptide Development | 56 | ||
2.3.1 Cell-Based and Molecular Systems | 56 | ||
2.3.2 Organ Systems | 57 | ||
2.3.3 Whole-Animal in vivo Screens | 58 | ||
2.3.4 High-Throughput in vivo Screens | 58 | ||
2.3.4.1 Simplifying Assumptions | 59 | ||
2.3.5 High-Content in vivo Screens | 60 | ||
2.3.6 High-Fidelity Disease Models | 61 | ||
2.3.7 Validation of a Target | 62 | ||
2.4 Summary | 62 | ||
References | 63 | ||
Chapter 3 - Peptide Biomarkers and Assay Development | 76 | ||
3.1 Naturally Occurring Peptide Ligands and Their\rApplications in Assay Development | 76 | ||
3.1.1 Peptide Hormones | 77 | ||
3.1.1.1 Somatostatin | 77 | ||
3.1.1.2 Growth Hormone-Releasing Peptides | 79 | ||
3.1.1.3 Enkephalin | 80 | ||
3.1.1.4 Melanocyte-Stimulating Hormone | 81 | ||
3.1.2 Cell-Penetrating Peptides | 82 | ||
3.1.2.1 Tat Peptides | 82 | ||
3.1.2.2 RGD Peptides | 82 | ||
3.1.3 Immunostimulatory Peptides | 83 | ||
3.1.3.1 MHC Peptides | 83 | ||
3.1.3.2 Native Immunostimulatory Peptides | 84 | ||
3.1.3.3 The Multiple Antigen Peptides | 85 | ||
3.2 Development of Peptide Biomarkers | 86 | ||
3.2.1 Chemical Synthesis | 86 | ||
3.2.1.1 One-Bead One-Compound Method | 86 | ||
3.2.1.2 One-Bead Two-Compound Method | 87 | ||
3.2.2 Semi-Synthesis of Peptide–Protein Bioconjugates | 90 | ||
3.2.2.1 Expressed Protein Ligation of Semi-Synthetic Polypeptides | 91 | ||
3.2.3 Biosynthesis of Peptide Biomarkers by Phage Display Biopanning | 92 | ||
3.3 Peptide Biomarkers in Assay Development | 94 | ||
3.3.1 Peptide Detection Assays of Biomarkers | 95 | ||
3.3.2 Peptidomimetic Assays in the Detection of Biomarkers | 97 | ||
3.3.3 Polypeptide Assays for the Detection of Biomarkers | 98 | ||
3.4 Pre-Clinical Applications of Peptide Biomarkers | 100 | ||
3.4.1 Cell- and in vivo-Based Studies of Peptide Ligands in Imaging and Cancer Diagnostics | 101 | ||
3.4.1.1 NIR–Peptide Conjugates | 101 | ||
3.4.1.2 Organic Fluorophore–Peptide Conjugates | 103 | ||
3.4.1.3 Quantum Dot-Peptide Conjugates | 103 | ||
3.4.1.4 Magnetic Nanoparticle–Peptide Conjugates | 104 | ||
3.4.2 Cell Based and in vivo Studies of Peptide Ligands as Therapeutics | 105 | ||
3.4.2.1 Photosensitizer–Peptide Conjugates | 105 | ||
3.4.2.2 Drug–Peptide Conjugates | 106 | ||
3.4.2.3 Killer Peptide Conjugates | 106 | ||
3.4.2.4 Peptide–Radionuclide Conjugates | 108 | ||
3.5 Conclusions and Future Outlook | 109 | ||
Acknowledgements | 109 | ||
References | 110 | ||
Chapter 4 - Peptide Library Technologies: Mixture-Based Library Generation and Screening | 116 | ||
4.1 Introduction | 116 | ||
4.2 Mixture-Based Peptide Libraries | 117 | ||
4.3 Mixture Library Synthesis | 117 | ||
4.4 Non-Peptide Libraries | 119 | ||
4.5 Mixture Library Screening and Deconvolution | 120 | ||
4.6 Mixture Activity Theory | 123 | ||
4.7 Opioid Ligands from Combinatorial Libraries | 124 | ||
4.8 Scaffold-Ranking Libraries | 125 | ||
4.9 Screening Mixture Libraries in vivo | 126 | ||
4.10 Computational Analysis | 127 | ||
4.11 Mixture Linkage | 127 | ||
4.12 Conclusion | 135 | ||
Acknowledgements | 135 | ||
References | 135 | ||
Chapter 5 - Macrocyclic Peptides for Intracellular Drug Targets | 141 | ||
5.1 Introduction and the Biology of Cellular Permeability | 141 | ||
5.2 Physical Basis of Passive Membrane Permeability for Cyclic Peptides | 144 | ||
5.3 Case Studies in Cyclic Peptide Cell Permeability Through Active Transport | 155 | ||
5.3.1 Cationic Peptides | 155 | ||
5.3.2 Transporter-Mediated Permeability | 162 | ||
5.3.3 Stapled Peptides | 164 | ||
5.4 Conclusion | 165 | ||
References | 165 | ||
Chapter 6 - Structural Design for Bioactive Peptides | 172 | ||
6.1 Introduction | 172 | ||
6.2 Design Strategies | 173 | ||
6.2.1 Chemical Optimization Strategies for Bioactive Peptides | 173 | ||
6.3 Selective Peptide-Based Therapeutics | 177 | ||
6.3.1 Angiotensin II Receptor Antagonist | 177 | ||
6.3.2 Antidiabetic Agents | 179 | ||
6.3.3 Anti-HIV Peptide | 180 | ||
6.3.4 Calcitonins | 180 | ||
6.3.5 Cardiovascular Agents | 180 | ||
6.3.6 Cholecystokinin Analogs | 181 | ||
6.3.7 Central Nervous System Agents | 181 | ||
6.3.8 Growth Hormone-Releasing Hormone and Analog | 181 | ||
6.3.9 Gonadotropin-Releasing Hormone and Analogs (Agonists) | 182 | ||
6.3.10 GnRH Antagonists | 183 | ||
6.3.11 Oxytocin, Its Antagonists and Analogs | 183 | ||
6.3.12 Secretin | 183 | ||
6.3.13 Somatostatin and Analogues (Agonists) | 185 | ||
6.3.14 Vasopressin Analogs | 187 | ||
6.3.15 Peptides with Other Chemical Modification | 187 | ||
6.4 Conclusion and Future Prospects | 188 | ||
Acknowledgements | 188 | ||
References | 188 | ||
Chapter 7 - Peptide Lead Optimization—Strategies and Tactics | 192 | ||
7.1 Introduction | 192 | ||
7.2 ADME Issues in Peptide Lead Optimization | 193 | ||
7.2.1 Metabolism | 193 | ||
7.2.2 Absorption and Distribution | 195 | ||
7.2.2.1 BBB Permeability | 195 | ||
7.2.3 Elimination | 198 | ||
7.3 Lead Peptide Optimization Strategies | 199 | ||
7.3.1 Classic Strategies | 199 | ||
7.3.1.1 Amino Acid Substitutions | 199 | ||
7.3.1.2 Truncation of Peptides | 201 | ||
7.3.1.3 Terminus Modification | 202 | ||
7.3.1.4 Peptide Bond Replacements | 202 | ||
7.3.1.5 Peptide Cyclization | 203 | ||
7.3.2 Recent Approaches to Peptide Design | 204 | ||
7.3.2.1 d-Peptides | 204 | ||
7.3.2.2 β-Amino Acids | 205 | ||
7.3.2.3 Cyclization Strategies | 205 | ||
7.3.2.3.1\rRing Closing Metathesis.Ring closing metathesis (RCM) has proven to be an extremely useful approach to cyclize peptides.71,75–79... | 205 | ||
7.3.2.3.2\rIntramolecular “Click” Chemistry.“Click” reactions yielding triazoles, which have been used extensively to conjugate various fun... | 207 | ||
7.3.2.3.3\rOther Cyclization Strategies.A variety of other cyclization strategies examined in peptides include novel dithioethers and diami... | 207 | ||
7.3.2.4 Macrocyclic Peptides | 210 | ||
7.3.2.4.1\rPolycyclic Peptides.There are a number of macrocyclic peptides that contain additional cyclic constraints.156 Many of these pept... | 213 | ||
7.4 Conclusions | 214 | ||
Acknowledgements | 214 | ||
References | 215 | ||
Chapter 8 - ADME Properties of Peptide Therapeutics in Drug Discovery and Development | 223 | ||
8.1 Introduction | 223 | ||
8.2 Absorption of Peptide Therapeutics | 224 | ||
8.2.1 Oral Absorption | 224 | ||
8.2.2 Subcutaneous Absorption | 225 | ||
8.2.3 Metabolism as a Barrier to Subcutaneous Absorption of Peptides | 228 | ||
8.2.4 Strategies for Understanding and Improving Subcutaneous Absorption in Lead Optimization | 231 | ||
8.3 Distribution of Peptide Therapeutics | 232 | ||
8.4 Elimination of Peptide Therapeutics | 235 | ||
8.4.1 Metabolism | 235 | ||
8.4.2 Renal Elimination | 237 | ||
8.4.3 Receptor-Mediated Endocytosis | 238 | ||
8.5 Strategies for Integrating Concepts of Peptide Elimination into the Design of Peptide Therapeutics | 239 | ||
8.5.1 Importance of Understanding Mechanism of Clearance | 239 | ||
8.5.2 Identifying Areas Susceptible to Metabolism | 240 | ||
8.5.2.1 Serum and Plasma | 240 | ||
8.5.2.2 Kidney Brush Border Membranes | 240 | ||
8.5.2.3 Purified and Recombinant Enzymes | 242 | ||
8.5.3 Strategies to Extend the Elimination Half-Life of Peptide Therapeutics | 244 | ||
8.5.3.1 Increasing Molecular Weight | 244 | ||
8.5.3.2 Protein Binding | 245 | ||
8.6 Predicting Human Pharmacokinetics from Non-Clinical Data | 246 | ||
8.7 Conclusions | 247 | ||
References | 248 | ||
Chapter 9 - Optimizing Peptides for Metabolic Diseases | 252 | ||
9.1 Introduction | 252 | ||
9.2 GLP-1 Analogs for Hyperglycemia | 254 | ||
9.2.1 Semaglutide | 256 | ||
9.2.2 Albiglutide | 258 | ||
9.2.3 Dulaglutide | 258 | ||
9.3 Structural Modifications to Increase Stability to DPPIV | 258 | ||
9.3.1 Use of β-Amino Acids | 259 | ||
9.3.2 P1′ Modifications | 260 | ||
9.3.3 N-Terminal Extension by a Single Amino Acid | 262 | ||
9.4 Conformationally Constrained GLP-1 Analogs | 262 | ||
9.5 Engineering Novel Constraints | 264 | ||
9.6 Short-Chain GLP-1 Analogs | 265 | ||
9.7 Design of Cross-Linked Oxyntomodulin Analogs | 267 | ||
9.8 Bifunctional PEGylated Exenatide-Amilinomimetic Hybrids | 268 | ||
9.9 Dual Agonists | 270 | ||
9.10 Teduglutide (GLP-2) Analog for Short Bowel Syndrome | 273 | ||
9.11 Conclusions | 275 | ||
Acknowledgements | 275 | ||
References | 276 | ||
Chapter 10 - Peptide Therapeutics: Oncology | 278 | ||
10.1 Introduction | 278 | ||
10.2 Discovery of Therapeutic Peptides Against Cancers | 279 | ||
10.2.1 Peptides Derived from Natural Sources | 279 | ||
10.2.1.1 Anticancer Peptides from Animals | 280 | ||
10.2.1.2 Anticancer Peptides from Plants | 281 | ||
10.2.1.3 Anticancer Peptides from Microbes | 285 | ||
10.2.2 Anticancer Peptides Developed from Combinatorial Peptide Libraries | 286 | ||
10.2.2.1 Anticancer Peptides from Phage-Display Peptide Libraries | 286 | ||
10.2.2.2 Anticancer Peptides from One-Bead One-Compound Combinatorial Peptide Libraries | 288 | ||
10.2.2.3 Ribosome-Mediated Synthesis of Natural Product-Like Cyclic Peptide Libraries | 289 | ||
10.2.3 De novo Design | 289 | ||
10.3 Optimization of Anticancer Peptides | 293 | ||
10.4 Examples of Peptide-Based Therapeutics Against Cancer | 295 | ||
10.4.1 Microtubule Inhibitors | 295 | ||
10.4.2 Cell Cycle Inhibitors | 295 | ||
10.4.3 Apoptotic Peptides (or Apoptosis-Inducing Peptides) | 296 | ||
10.4.4 Peptides Target Signal Transduction Pathways | 297 | ||
10.4.5 Immuno-Active Peptides | 297 | ||
10.4.5.1 Peptide Vaccines | 297 | ||
10.4.5.2 Immune Checkpoint Blockade Peptide Inhibitors | 300 | ||
10.4.6 Peptide Hormones | 300 | ||
10.5 Tumor-Targeting Peptides for Targeted Delivery of Anticancer Drugs | 301 | ||
10.5.1 Peptide–Drug Conjugates | 301 | ||
10.5.2 Peptide-Decorated Nanomedicine | 305 | ||
10.5.3 Self-Assembling PDCs | 306 | ||
10.6 Therapeutic Anticancer Peptides on the Market and in Clinical Trials | 307 | ||
10.6.1 Examples of Approved Peptide-Derived Drugs for Cancer Therapy | 307 | ||
10.6.2 Anticancer Peptides, PDCs and Peptidic Drugs in Clinical Development | 307 | ||
10.7 Conclusion and Perspectives | 310 | ||
Acknowledgements | 312 | ||
References | 312 | ||
Chapter 11 - Development of Peptide-Based Diagnostic and Therapeutic Agents in Oncology | 326 | ||
11.1 Introduction | 326 | ||
11.2 Cancer Imaging: Technology Systems and Imaging Agents | 328 | ||
11.2.1 Positron Emission Tomography and Single Photon Emission Computed Tomography | 329 | ||
11.2.2 Optical Imaging and Spectroscopy | 331 | ||
11.2.3 Knottin Peptides | 332 | ||
11.2.3.1 Chlorotoxin | 332 | ||
11.2.3.2 CTX Nanoparticles | 334 | ||
11.2.4 Affibodies | 334 | ||
11.2.5 Two-Helix Affibodies | 336 | ||
11.2.6 Fibronectin Domain | 337 | ||
11.2.7 DARPins | 338 | ||
11.2.8 Activatable Peptides | 338 | ||
11.3 Extracellular Targets: Combinatorial Libraries to Identify Targeting Peptide Probes | 340 | ||
11.3.1 Phage Display Libraries | 340 | ||
11.3.2 Chemical Libraries | 342 | ||
11.4 Intracellular Targets: Protein–Protein Interactions | 343 | ||
11.4.1 Cyclic Peptides | 344 | ||
11.4.2 Stapled Peptides | 346 | ||
11.4.3 Helix-Stabilizing Chemistry | 349 | ||
11.4.4 β-Hairpin Mimetics | 350 | ||
11.4.5 Bicyclic Peptides | 351 | ||
11.4.6 β-Peptides and Peptoids | 351 | ||
11.4.7 Grafted Bioactive Peptides on Mini-Protein Scaffolds | 352 | ||
11.5 Peptide Vaccines | 352 | ||
11.6 Conclusions | 355 | ||
References | 356 | ||
Chapter 12 - Designing an Effective Peptide Vaccine | 364 | ||
12.1 Introduction | 364 | ||
12.2 Key Concepts for Peptide Vaccine Design | 365 | ||
12.3 Prophylactic Peptide Vaccines Targeting Humoral Immunity | 366 | ||
12.3.1 Multivalent Display | 366 | ||
12.3.2 Conjugation Chemistry | 368 | ||
12.3.3 Vaccine Adjuvants | 368 | ||
12.3.4 Toll-Like Receptor Agonists | 369 | ||
12.3.5 Epitope Selection | 370 | ||
12.3.6 A Case Study: The Elusive Malaria Vaccine | 371 | ||
12.4 Therapeutic Peptide Vaccines Targeting Cellular Immunity | 373 | ||
12.4.1 Peptide Vaccines Against Viral Disease and Cancer | 373 | ||
12.4.1.1 Conjugates of Class I and Class II Epitope Peptides | 374 | ||
12.4.1.2 Peptide–TLR Agonist Conjugates | 374 | ||
12.4.1.3 Tripartite Peptide Vaccines | 374 | ||
12.4.1.4 Vaccines Based on Longer Tc Peptides | 375 | ||
12.4.1.5 A Renaissance of Peptide Cancer Vaccines | 375 | ||
12.4.2 Peptide Vaccines for Allergy and Autoimmune Disease | 377 | ||
12.4.2.1 Peptide Vaccines for Allergy | 378 | ||
12.4.2.2 Peptide Vaccines for Autoimmune Diseases | 379 | ||
12.4.2.3 Vaccines Based on Altered Peptide Ligands | 380 | ||
12.5 Conclusions | 380 | ||
References | 381 | ||
Chapter 13 - Peptide Therapeutics: Neuropeptides | 391 | ||
13.1 Introduction | 391 | ||
13.2 General Strategies for Enhancing Receptor Selectivity, Peptide Stability Against Biodegradation and Bioavailability | 392 | ||
13.2.1 Receptor Selectivity | 392 | ||
13.2.2 Stabilizing Against Proteolytic Degradation | 393 | ||
13.2.3 Bioavailability | 393 | ||
13.3 Receptor Types | 394 | ||
13.3.1 Bradykinin Receptors | 394 | ||
13.3.2 Melanocortin Receptors | 396 | ||
13.3.2.1 NMR Structure-Based Design | 396 | ||
13.3.2.2 Ligand–Receptor Interactions | 398 | ||
13.4 Developing Selective Ligands for the µ and δ Receptors | 400 | ||
13.4.1 Improving Agonist Selectivity and Activity | 400 | ||
13.4.2 Improving µ-Antagonist Selectivity and Potency | 401 | ||
13.4.3 Improving δ-Agonist Potency and Selectivity | 402 | ||
13.5 Selective Ligands for the KOR | 402 | ||
13.6 Conclusions | 405 | ||
References | 405 | ||
Chapter 14 - From Spider Toxins to Therapeutics—Developing Selective Nav1.7 Peptide Inhibitors for Pain | 411 | ||
14.1 Introduction | 411 | ||
14.2 General Considerations | 414 | ||
14.2.1 Nav1.7 Structural Biology | 414 | ||
14.2.2 Spider Venom Toxin Peptide Receptor Pharmacology | 415 | ||
14.2.3 ADME Properties | 416 | ||
14.3 Representative Examples | 417 | ||
14.3.1 ProTx-II | 417 | ||
14.3.1.1 Overview | 417 | ||
14.3.1.2 ProTx-II Receptor Pharmacology | 418 | ||
14.3.1.3 Purdue’s SAR Analysis of ProTx-II and PaTx-I | 419 | ||
14.3.1.4 Janssen’s Selectivity Optimization of the ProTx-II Scaffold | 420 | ||
14.3.1.5 ProTx-II in vivo Results | 420 | ||
14.3.2 HWTx-IV | 421 | ||
14.3.2.1 Overview | 421 | ||
14.3.2.2 Binding Sites of HWTx-IV | 421 | ||
14.3.2.3 HWTx-IV Potency/Selectivity Optimization | 422 | ||
14.3.2.3.1\rMedImmune/AstraZeneca’s Optimization Efforts.MedImmune and AstraZeneca reported on efforts to optimize the potency of HWTx-IV in... | 422 | ||
14.3.2.3.2\rJanssen’s Optimization Efforts.Concurrent to the above efforts, a research group from Janssen published patent applications113,1... | 423 | ||
14.3.2.4 HWTx-IV in vivo Results | 424 | ||
14.3.3 GpTx-1 | 424 | ||
14.3.3.1 Overview | 424 | ||
14.3.3.2 GpTx-1 Binding Model with Nav1.7 | 425 | ||
14.3.3.3 GpTx-1 Selectivity Optimization | 425 | ||
14.3.3.4 GpTx-1 Conjugation Efforts | 427 | ||
14.3.3.5 GpTx-1 in vivo Activities | 428 | ||
14.3.4 JzTx-V | 428 | ||
14.3.5 CcoTx-1 | 428 | ||
14.3.5.1 Overview | 428 | ||
14.3.5.2 CcoTx-1 Potency/Selectivity Optimization | 429 | ||
14.3.6 Discussion and Future Perspective | 431 | ||
Acknowledgements | 431 | ||
References | 431 | ||
Chapter 15 - Structure and Function of Stress-Responsive Peptides in Insects | 438 | ||
15.1 Introduction | 438 | ||
15.2 Discovery | 439 | ||
15.3 Structure | 440 | ||
15.3.1 Core Domain | 442 | ||
15.3.2 N-Terminal Extension | 443 | ||
15.3.3 C-Terminal Extension | 444 | ||
15.4 Biological Functions | 445 | ||
15.4.1 Plasmatocyte Spreading and Attachment | 445 | ||
15.4.2 Paralysis | 446 | ||
15.4.3 Wound Healing | 446 | ||
15.4.4 Phagocytosis | 446 | ||
15.4.5 Reactive Oxygen/Nitrogen Species and Nitric Oxide Synthase Production | 447 | ||
15.4.6 Induced AMP Synthesis | 447 | ||
15.4.7 Growth-Blocking Activity | 447 | ||
15.4.8 Mitogenic Activity | 448 | ||
15.5 Conclusion | 448 | ||
Acknowledgements | 449 | ||
References | 449 | ||
Chapter 16 - Technologies for Oral Delivery of Peptides | 452 | ||
16.1 Introduction | 452 | ||
16.2 Non-Invasive Routes for Peptide Delivery | 453 | ||
16.3 Oral Peptide Delivery: Advantages and Challenges | 455 | ||
16.4 Peptide Absorption by the GI Tract | 456 | ||
16.5 Considerations on Choice of Peptide for Oral Delivery | 457 | ||
16.5.1 Size | 457 | ||
16.5.2 Stability | 458 | ||
16.5.3 Intestinal Peptidases | 458 | ||
16.5.4 Aggregation | 458 | ||
16.6 Technologies for Enhancement of Oral Delivery | 459 | ||
16.6.1 Inhibiting Peptide Degradation | 459 | ||
16.6.1.1 pH Lowering | 459 | ||
16.6.1.2 Amino Acid Replacement | 459 | ||
16.6.1.3 Protease Inhibitors | 460 | ||
16.6.1.4 Covalent Modification of Peptides | 463 | ||
16.6.1.4.1\rFatty Acids.Chicken cystatin, a model protein inhibitor of cysteine proteinases, was acylated with fatty acyl residues of 6–18 c... | 463 | ||
16.6.1.4.2\rPolyethylene Glycol.Acylated peptides are more hydrophobic than the parent molecule and tend to aggregate with increased fatty a... | 464 | ||
16.6.1.4.3\rPEG Oligomers.As an alternative to fatty acids or PEG derivatization, comparatively small, amphiphilic monodisperse oligomers th... | 465 | ||
16.6.1.4.4\rTransporter Ligands.Peptides can also be made more protease resistant by conjugating them to ligands that will bind them to tran... | 466 | ||
16.6.1.5 Peptide Cyclization | 467 | ||
16.6.2 Permeation Enhancers | 467 | ||
16.7 Technologies in Pre-Clinical and Clinical Development | 473 | ||
16.7.1 Enteris Biopharma Peptelligence® Technology | 473 | ||
16.7.2 Chiasma Transient Permeability Enhancement Technology | 476 | ||
16.7.3 Oramed POD™ Technology | 477 | ||
16.7.4 Merrion Pharmaceuticals GIPET™ Technology | 477 | ||
16.7.5 Emisphere Eligen® Technology | 478 | ||
16.7.6 Diasome Pharmaceuticals Hepatocyte-Directed Vesicle Technology | 478 | ||
16.7.7 NOD Pharmaceuticals Insulin NOD Tech Nanoparticle Technology | 479 | ||
16.7.8 Technologies in Preclinical Testing | 479 | ||
16.7.8.1 Arisgen ArisCrown Technology | 479 | ||
16.7.8.2 NanoMega Medical Nanoparticulate System | 479 | ||
16.7.8.3 Rani Therapeutics Robotic Pill | 480 | ||
16.7.8.4 Aegis Therapeutics Intravail® Technology | 480 | ||
16.7.8.5 Miscellaneous Studies | 481 | ||
16.8 Currently Approved Oral Peptide Pharmaceuticals | 481 | ||
16.9 Future Developments | 482 | ||
Acknowledgements | 484 | ||
References | 484 | ||
Chapter 17 - Phylomer Libraries: A Rich Source of Peptide Hits in Phenotypic and Target-Directed Screens | 497 | ||
17.1 Composition and Construction of Phylomer\rLibraries | 497 | ||
17.1.1 Addressing the Challenges of Limited Structural Diversity in Random Peptide Libraries | 497 | ||
17.1.2 Characterisation of Phylomer Libraries: Genome Representation and Sequence Diversity | 499 | ||
17.1.3 Structural Diversity Within Phylomer Libraries | 503 | ||
17.2 Phylomer Libraries and Target-Directed Screening | 505 | ||
17.2.1 A Diversity of Targets Can Be Efficiently Hit with Phylomers | 505 | ||
17.2.2 Intracellular Target Screens Show High Functional Hit Rates and Enrichment for Biophysical Characteristics | 506 | ||
17.2.3 Quality of Primary Hits from Phylomer Libraries | 508 | ||
17.2.4 Structurally Related Families of Phylomer Sequences from Hits Selected on an Extracellular Target | 509 | ||
17.2.5 Targeting Peptides: Biopanning Phylomer Libraries Against Neurotoxic β-Amyloid Oligomers | 510 | ||
17.3 Phylomer Libraries as a Rich Source of Hits in Phenotypic Screening | 512 | ||
17.3.1 Phenotypic Screens of Mini-Libraries Identify Hits Targeting Various Pathways | 512 | ||
17.3.2 Phylomers as Target Validation Reagents | 514 | ||
17.4 Conclusions | 514 | ||
Acknowledgements | 515 | ||
References | 515 | ||
Chapter 18 - Solid-Phase Peptide Synthesis, the State of the Art: Challenges and Opportunities | 518 | ||
18.1 Introduction | 518 | ||
18.2 The Current Situation of the Synthetic Peptide Field | 520 | ||
18.3 Is It a Green Process | 520 | ||
18.4 The Solid Support | 521 | ||
18.5 Protecting-Group Strategies | 522 | ||
18.6 Handles/Functionalized Solid Support | 524 | ||
18.7 Coupling Reagents | 526 | ||
18.8 Integrated Strategies | 530 | ||
18.9 Cyclic Peptides | 534 | ||
18.9.1 Amides and Depsipeptides: Orthogonal Protecting Groups | 534 | ||
18.9.2 Disulfides | 537 | ||
18.9.3 Others | 539 | ||
18.10 Native Chemical Ligation | 540 | ||
18.11 Conclusions | 543 | ||
Acknowledgements | 543 | ||
References | 543 | ||
Subject Index | 551 |