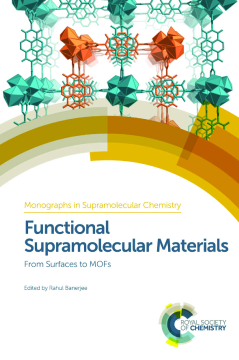
Additional Information
Book Details
Abstract
Supramolecular materials have a great number of applications due to the reversibility of their non-covalent molecular interactions, such as reversible hydrogen bonding, host–guest interactions and electrostatic interactions. This book provides a comprehensive source of information on the structure and function of organic and metal–organic supramolecular materials.
The chapters of this book provide an overview of supramolecular material assembly at various scales, including the formation of 2D polymers and molecular cages. The role of intermolecular interactions in solid and solution state self-assembly is discussed, as is the role of mechanochemistry on molecular and supramolecular architectures. Finally, novel applications of these materials in molecular recognition, catalysis, light harvesting and environmental remediation are covered.
Functional Supramolecular Materials will be of interest to graduate students and researchers in academia and industry in the fields of supramolecular chemistry and functional materials science.
Rahul Banerjee received his PhD degree from University of Hyderabad, Hyderabad in 2006 under the supervision of Prof. Gautam R. Desiraju. After postdoctoral work at UCLA with Prof. Omar M. Yaghi (2006-2008), he joined the National Chemical Laboratory, Pune, India in 2008 as a Scientist. His research interests include the study of structural chemistry with chemical synthesis to design new materials for hydrogen storage and carbon sequestration. Additionally, his group is also engaged in design and synthesis of lightweight materials for storage, capture and proton conduction. Dr Banerjee is an Editorial board member and associate editor of CrystEngComm. He has also served as a co-editor of Acta Crystallographica Section E in 2011-2012. Dr Banerjee has been invited to become a Fellow of the Royal Society of Chemistry in 2013 in recognition of his significant contribution to Chemical Science and, in particular, to his work on the board of CrystEngComm.
Table of Contents
Section Title | Page | Action | Price |
---|---|---|---|
Cover | Cover | ||
Preface | v | ||
Contents | vii | ||
Chapter 1 Surface Self-assembly of Functional Supramolecular Networks | 1 | ||
1.1 Introduction | 1 | ||
1.2 Two-dimensional Arrays Assembled Using Hydrogen-bonding | 3 | ||
1.3 Two-dimensional Arrays Assembled Using van der Waals Interactions | 19 | ||
1.4 Covalently Coupled Two-dimensional Arrays: Planar Covalent Organic Frameworks | 21 | ||
1.5 Complex Structures with Low Degrees of Symmetry | 26 | ||
1.6 Conclusions | 31 | ||
References | 32 | ||
Chapter 2 Designing Soft Supramolecular Materials Using Intermolecular Interactions | 37 | ||
2.1 Introduction | 37 | ||
2.2 Designing LMWGs | 38 | ||
2.2.1 Molecular Engineering Approach | 39 | ||
2.2.2 Crystal Engineering Approach | 46 | ||
2.3 Metallogels | 63 | ||
2.4 Summing Up and Future Direction | 65 | ||
Acknowledgments | 68 | ||
References | 69 | ||
Chapter 3 Anion Binding in Tripodal Receptors and Supramolecular Capsular Dimeric Assemblies | 75 | ||
3.1 Introduction | 75 | ||
3.2 Anion Recognition with Tripodal Amide Receptors | 76 | ||
3.3 Anion Recognition with Tripodal Urea Receptors | 86 | ||
3.4 Anion Recognition with Tripodal Amine Receptors | 99 | ||
3.5 Anion Recognition with Tripodal Guanidinium Receptor | 100 | ||
3.6 Anion Recognition with Tripodal Imidazole and Imidazolium Receptors | 101 | ||
3.7 Anion Recognition with Tripodal Pyridinium Receptors | 102 | ||
3.8 Steroid-based Tripodal Anion Receptors with Diversified Binding Motifs | 102 | ||
3.9 Anion Recognition Through Tripodal Halogen Bond Donors | 103 | ||
3.10 Concluding Remarks | 104 | ||
References | 105 | ||
Chapter 4 Functional Molecular and Supramolecular Materials for Electron Transfer Reactions and their Applications | 110 | ||
4.1 Introduction | 110 | ||
4.2 Tetracyanoquinodimethanes | 112 | ||
4.2.1 Introduction to Tetracyanoquinodimethanes | 112 | ||
4.2.2 TCNQ-based Radical Anion and Dianion | 113 | ||
4.3 Arylenediimides | 116 | ||
4.3.1 Introduction to NDIs | 118 | ||
4.3.2 ET Reactions of NDI | 119 | ||
4.3.3 Electron Delocalization/Stabilization by Noncovalent Means in NDI Radical Ions | 121 | ||
4.3.4 Photochemical Reactions and Photoexcitation of NDIs and NDI Radical Ions | 125 | ||
4.4 Perylenediimides | 128 | ||
4.4.1 Introduction to PDIs | 128 | ||
4.4.2 Electron Injection and Transport With PDIs | 130 | ||
4.4.3 ET Reactions of PDIs | 135 | ||
4.4.4 ET in PDI-based Supramolecular Systems | 141 | ||
4.5 Introduction to Corannulenes | 146 | ||
4.5.1 ET and Solid-state Characterization of Anionic Corannulenes | 147 | ||
4.6 Introduction to Bipyridinium Systems | 149 | ||
4.6.1 ET Reactions of Bipyridinium-based Systems | 149 | ||
4.6.2 Supramolecular Stabilization of the MV Radical Cation | 151 | ||
4.6.3 Multi-ET Reactions/Electron Reservoirs | 152 | ||
4.7 Applications | 155 | ||
4.7.1 Sensors | 155 | ||
4.7.2 Organic Battery | 163 | ||
4.8 Conclusion | 166 | ||
Abbreviations | 166 | ||
References | 167 | ||
Chapter 5 Influence of Supramolecular Interactions on the Solid-state Photoreactivity of Metal Complexes | 180 | ||
5.1 Introduction | 180 | ||
5.2 Metallophilic Interactions to Align Terminal Ligands Bearing Olefin Bonds | 181 | ||
5.2.1 Role of Argentophilic Interactions Influencing the Photoreactivity in Ag(I) Complexes | 182 | ||
5.2.2 Argentophilic Interactions versus Donor-Acceptor Interactions | 184 | ||
5.2.3 Intra versus Intermolecular Photoreaction: Influence of C-H…π Interactions | 185 | ||
5.3 Ligand Design in Controlling Photoreactivity of 1,2-Bi(4-pyridyl)ethylene (bpe) Pairs | 187 | ||
5.4 Diphosphine Ligands as Clipping Agents | 187 | ||
5.4.1 Photoreactive Gold(i) Macrocycles | 188 | ||
5.5 Photoreactive Organometallic Cages and Molecular Rectangles | 189 | ||
5.5.1 Organometallic Rectangles Containing Bisimidazolate Derivatives | 189 | ||
5.5.2 Photoreactive Organometallic Rectangles Containing Oxalate Ligands | 190 | ||
5.5.3 Unusual Formation of tpcb Isomer from the Crisscrossed Alignment of bpe in Photoreactive Organometallic Cages | 191 | ||
5.5.4 Solid-state and Solution Photoreactivity of an Organometallic Rectangle with Muconate Bridging | 191 | ||
5.5.5 Photoreactive NHC-based Metalla Macrocycles | 192 | ||
5.5.6 An Unusual Photodimerization of Coumarin Derivatives in Metalla Macrocycles | 192 | ||
5.6 Switching of Magnetic Properties due to [4+4] Cycloaddition Reaction in a Cu(ii) Complex of Anthracene Derivatives | 194 | ||
5.7 Unusual Fusion of Interlocked Cages by [2+2] Cycloaddition Reaction | 196 | ||
5.8 1D Coordination Polymers from the [2+2] Cycloaddition of Metal Complexes | 197 | ||
5.8.1 Historical Developments | 197 | ||
5.8.2 Polymerization of a Metal Complexes with bpe as a Monodentate Ligand | 198 | ||
5.8.3 Pedal Motion of Olefin Bonds by Grinding in a Zn(ii) Complex | 199 | ||
5.8.4 Photoreactivity in Similar Mn(ii) Complexes Containing Unsymmetrical Ligands | 199 | ||
5.9 Photoreactive Polymorphs of Co-crystals Containing a Metal Complex and an Organic Molecule Yielding Two Different Cyclobutane Isomers | 200 | ||
5.9.1 Photoreactivity in Similar Metal Complexes with Asymmetrical Bipyridyl Spacer Ligand | 202 | ||
5.10 Photoreactive Zwitter Ionic Metal Complexes Containing bpe-H+ Cationic Ligand | 202 | ||
5.11 Making the Terminal Ligands of the Metal Complexes Photoreactive | 204 | ||
5.11.1 Photoreactivity of 4-Strylpyridine Ligands in Metal Complexes | 204 | ||
5.11.2 Unusual Solid-state Intermolecular Phenyl-Olefin Dimerization in a Zn(ii) Complex | 206 | ||
5.12 Photosalient Behaviour of Photoreactive Metal Complexes | 207 | ||
5.13 Alignment of Pyridinium Cations in Metal Salts | 210 | ||
5.14 Conclusions and Summary | 211 | ||
Acknowledgments | 211 | ||
References | 211 | ||
Chapter 6 Metal-organic Frameworks with Tunable Electrical and Optical Properties | 217 | ||
6.1 Introduction | 217 | ||
6.2 Electrically Conducting MOFs | 219 | ||
6.2.1 MOFs with Intrinsic Electrical Conductivity | 220 | ||
6.2.2 Guest-induced Electrical Conductivity in Porous MOFs | 225 | ||
6.3 Optically-tunable Metal-organic Frameworks | 232 | ||
6.3.1 Electrochromic MOFs | 232 | ||
6.3.2 Photoluminescent MOFs | 234 | ||
6.3.3 Photovoltaic Application of Light-harvesting MOFs | 236 | ||
6.3.4 Photochromic MOFs | 236 | ||
6.3.5 Solvatochromic MOFs | 238 | ||
6.3.6 Chemical Sensors Based on Luminescent MOFs | 239 | ||
6.4 Concluding Remarks | 242 | ||
Acknowledgments | 242 | ||
References | 242 | ||
Chapter 7 Conducting Framework Materials | 247 | ||
7.1 Introduction | 247 | ||
7.1.1 Scope of this Chapter | 249 | ||
7.2 Mechanisms of Charge Transport | 249 | ||
7.3 Conducting Coordination Solids: Historical Foundations | 251 | ||
7.4 Conducting Framework Materials: Recent Experimental Milestones | 259 | ||
7.4.1 Exploiting Redox Matching: Donor-Acceptor and Mixed Valency | 260 | ||
7.4.2 Exploiting π-Interactions | 263 | ||
7.4.3 Other Examples: Conductivity Induced by External Stimuli | 268 | ||
7.5 Methods of Assessing Conductive Properties in Frameworks | 271 | ||
7.5.1 Contact Methods | 271 | ||
7.5.2 Contactless Methods | 272 | ||
7.6 Summary and Future Outlook | 273 | ||
References | 275 | ||
Chapter 8 Functional Metal-organic Frameworks for Enzyme/Protein Immobilization | 281 | ||
8.1 Introduction | 281 | ||
8.2 Enzyme Immobilization MOFs via Chemical Binding | 283 | ||
8.3 Enzyme Immobilization MOFs via Physical Adsorption | 285 | ||
8.4 Enzyme Immobilization MOFs via Encapsulation | 290 | ||
8.5 Conclusions | 294 | ||
References | 295 | ||
Chapter 9 Porous Metal Organic Polygons and Polyhedra - Intrinsic vs. Extrinsic Porosity | 297 | ||
9.1 Introduction | 297 | ||
9.1.1 Polygons | 301 | ||
9.1.2 Polyhedra | 305 | ||
9.2 Materials and Their Properties | 308 | ||
9.2.1 Copper Nanoball | 309 | ||
9.2.2 Tetrahedral MOPs | 312 | ||
9.3 Applications and Characterisations | 314 | ||
9.4 Conclusion | 319 | ||
References | 320 | ||
Chapter 10 Functional Metallo-supramolecular Polyhedral Capsules and Cages | 325 | ||
10.1 Introduction | 325 | ||
10.2 Design Principles | 326 | ||
10.3 Encapsulation | 328 | ||
10.4 Selectivity, Separations and Sequestration | 329 | ||
10.4.1 Anion Recognition | 330 | ||
10.4.2 Cation Binding | 348 | ||
10.4.3 Charge-neutral Guest Encapsulation | 352 | ||
10.5 Reactivity Modulation | 366 | ||
10.5.1 Catalytic Polyhedra | 367 | ||
10.5.2 Reaction Inhibition | 375 | ||
10.6 Conclusions and Outlook | 377 | ||
References | 378 | ||
Chapter 11 Designing Functional Porous Organic Frameworks for Gas Storage and Separation | 388 | ||
11.1 Introduction | 388 | ||
11.2 Synthesis of POFs | 389 | ||
11.2.1 General Strategy for Synthesis of Porous Organic Polymers (POPs) | 389 | ||
11.2.2 Different Approaches for Synthesis of HCPs | 392 | ||
11.3 Design and Synthesis of POFs for Hydrogen Storage | 394 | ||
11.4 Methane Storage by POFs | 399 | ||
11.5 Synthesis of Functionalized POFs for CO2 Capture | 401 | ||
11.6 Conclusion | 406 | ||
References | 407 | ||
Chapter 12 Gated and Stepwise Sorption Processes in Functional Metal-organic Frameworks | 412 | ||
12.1 Introduction | 412 | ||
12.2 Rigid and Flexible MOFs | 413 | ||
12.3 Sorption in Flexible MOFs: Gated and Stepwise Adsorption | 414 | ||
12.4 Structural Flexibility in a 1D Coordination Polymer | 415 | ||
12.5 Flexibility in 2D MOFs | 416 | ||
12.5.1 Stepwise Sorption in 2D MOFs | 417 | ||
12.5.2 Gated Sorption in 2D MOFs | 419 | ||
12.6 Flexibility in 3D MOFs | 424 | ||
12.6.1 Stepwise Sorption in 3D MOFs | 424 | ||
12.6.2 Gated Sorption in 3D MOFs | 435 | ||
12.7 Future Outlook | 446 | ||
Abbreviations | 447 | ||
Acknowledgments | 448 | ||
References | 448 | ||
Subject Index | 454 |