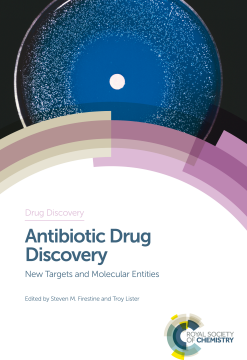
Additional Information
Book Details
Abstract
Recent years have seen a resurgence of antibiotic drug discovery. This book brings together the relevant information to assess the state-of-the-art. It identifies and elaborates the most recent and compelling strategies for antibiotic drug discovery with a primary focus on new targets, mechanisms and molecular entities. Addressing the need for continued investment in antibiotic drug development, the book provides a point of reference for the rapidly expanding infectious disease research community.
In addition to its attention on new targets, the book focusses on the medicinal chemistry and chemistry of the targets. Within this framework, chapters from leading researchers in academia and industry address findings in important areas such as biofilm production, narrow spectrum antibiotics and novel antibacterials from previously uncultured soil bacteria.
This book will be a useful resource for postgraduate students and researchers in medicinal chemistry wishing to understand the latest approaches to antibiotic drug discovery.
The book will be of interest to a large audience, not only to medicinal chemists in the field, to whom it can serve as a valuable point of reference, but to anyone wishing to follow the latest approaches in antibiotic drug design. The book is also valuable as a textbook for postgraduate students in medicinal chemistry: concepts are explained clearly and concisely throughout all chapters; sections are well organized and nicely illustrated.
Dr Donatella Tondi, Universit/ degli Studi di Modena e Reggio Emilia (Italy)
Steven M. Firestine is an Associate Professor of Medicinal Chemistry at the Eugene Applebaum College of Pharmacy and Health Sciences at Wayne State University. He received a B.S. with honors in chemistry from the University of Michigan and a PhD in Medicinal Chemistry from Purdue University. Dr. Firestine conducted postdoctoral research in the laboratory of Dr. Stephen J. Benkovic before joining the medicinal chemistry faculty at Duquesne University. In 2005 he moved to Wayne State University. Dr. Firestine maintains an active research program focused in the area of anti-infective drug design. To date, he has authored more than 40 articles in peer-reviewed research journals and presented more than 75 research abstracts and seminars. Dr. Firestine is an active reviewer for the NIH and the MRC. He serves on the editorial board of ACS Combinatorial Sciences and is a reviewer for numerous scientific journals.
Troy Lister, PhD, is Head of Chemistry at Spero Therapeutics in Cambridge, MA. Dr. Lister previously held roles as Team Leader of Infection Chemistry at AstraZeneca and Investigator III in Global Discovery Chemistry at the Novartis Institute for BioMedical Research. He has lead numerous discovery programs searching for novel agents targeting bacterial and viral pathogens including multidrug resistant Gram negative and Gram positive bacteria, hepatitis C virus, human rhinovirus, respiratory syncytial virus, ebola virus and human enterovirus. Dr. Lister has led programs from the earliest stages of target selection and high throughput screening, through lead identification and optimization, to candidate selection and IND enablement. He is an author of 15 peer review publications, has extensive experience in natural product chemistry and is a member of the American Chemical Society. Dr. Lister received his B.Tech, B.S. and PhD degrees from Flinders University in Adelaide, Australia, and completed a post-doctoral fellowship at The Scripps Research Institute in La Jolla, California with Professoer K.C. Nicolaou.
Table of Contents
Section Title | Page | Action | Price |
---|---|---|---|
Cover | Cover | ||
Antibiotic Drug Discovery: New Targets and Molecular Entities | i | ||
Preface | vii | ||
Contents | xi | ||
Chapter 1 - Treatment of Clostridium difficile Infections | 1 | ||
1.1 Background | 1 | ||
1.2 CDI Symptoms and Progression | 5 | ||
1.3 Relapse | 5 | ||
1.4 Diagnosis | 6 | ||
1.5 Prevention Measures—General Hospital Practice and Other Prevention Methods | 7 | ||
1.6 Current Treatment and Antibiotics | 9 | ||
1.6.1 Anti-Toxins | 10 | ||
1.6.2 Vaccines | 12 | ||
1.6.3 Anti-Germinants | 13 | ||
1.6.4 Fecal Transplantation | 13 | ||
1.6.5 Probiotics | 14 | ||
1.7 Conclusion | 15 | ||
References | 15 | ||
Chapter 2 - Targeting Purine Biosynthesis for Antibacterial Drug Design | 20 | ||
2.1 Introduction | 20 | ||
2.1.1 The De novo Purine Nucleotide Biosynthesis Pathway | 21 | ||
2.1.2 The Purine Conversion Pathways | 22 | ||
2.1.3 The Purine Salvage Pathways | 24 | ||
2.1.4 Availability of Purine Bases and Nucleosides | 25 | ||
2.1.5 The Complex Interplay Between Salvage Pathways and Precursor Availability | 25 | ||
2.2 The Essentiality of Enzymes of the Purine Biosynthetic Pathways | 26 | ||
2.2.1 Essentiality of Purine Nucleotide Biosynthesis for Growth in Rich Media | 26 | ||
2.2.2 Essentiality of Purine Nucleotide Biosynthesis During Infection | 27 | ||
2.2.3 Inhibitors May Not Have the Same Phenotypes as Gene Knockouts | 30 | ||
2.2.4 The Problem of Resistance | 30 | ||
2.3 Progress Targeting the Enzymes of De novo Purine Nucleotide Biosynthesis | 30 | ||
2.4 Progress Targeting Enzymes in the Purine Nucleotide Conversion Pathways | 31 | ||
2.4.1 IMPDH Structure and Mechanism | 32 | ||
2.4.2 Prokaryotic and Eukaryotic IMPDHs: A Comparative Analysis of Rational Drug Design | 34 | ||
2.4.3 Repurposing Cryptosporidium IMPDH Inhibitors as Antibiotics | 36 | ||
2.5 Conclusion | 38 | ||
Acknowledgements | 38 | ||
References | 39 | ||
Chapter 3 - Inhibitors of Biofilm Production | 43 | ||
3.1 Introduction | 43 | ||
3.2 Biofilms | 43 | ||
3.3 Strategies for Combating Biofilms | 44 | ||
3.3.1 Quorum Sensing Inhibitors | 44 | ||
3.3.2 Inhibitors of AHL Based Quorum Sensing as Biofilm Inhibitors | 45 | ||
3.3.3 Inhibitors of AIP-Based Quorum Sensing as Biofilm Inhibitors | 47 | ||
3.3.4 Inhibitors of AI-2 Based Quorum Sensing as Biofilm Inhibitors | 48 | ||
3.4 Inhibition of Bacterial Signaling Pathways | 49 | ||
3.4.1 Interference with c-di-GMP Signaling to Inhibit Biofilm Formation | 49 | ||
3.4.2 Inhibition of Indole Signaling Pathways to Prevent Biofilm Formation | 50 | ||
3.4.3 Inhibition of Two-Component Signal Transduction Systems (TCS) to Prevent Biofilm Formation | 52 | ||
3.4.4 Inhibition of Other Signaling Pathways to Prevent Biofilm Formation | 53 | ||
3.5 Identification of Natural Products and Analogues that Prevent Biofilm Formation | 54 | ||
3.5.1 Plant Natural Products and Analogues that Prevent Biofilm Formation | 54 | ||
3.5.2 Marine Natural Products and Analogues that Prevent Biofilm Formation | 55 | ||
3.5.3 Other Natural Products that Prevent Biofilm Formation | 58 | ||
3.6 Antimicrobial Peptides (AMPs) that Prevent Biofilm Formation | 58 | ||
3.7 Inhibition of Efflux to Prevent Biofilm Formation | 60 | ||
3.8 Matrix Degradation to Prevent Biofilm Formation | 60 | ||
3.9 Conclusions | 62 | ||
References | 62 | ||
Chapter 4 - Narrow Spectrum Antibacterial Agents | 76 | ||
4.1 Introduction | 76 | ||
4.2 Natural Products | 80 | ||
4.3 Synthetic and Target-Based Approaches | 86 | ||
4.4 Future Prospects | 93 | ||
4.5 Conclusions | 95 | ||
References | 95 | ||
Chapter 5 - The LPS Transport Pathway: A New Target for the Development of Gram-Negative Antibiotics | 103 | ||
5.1 Introduction | 103 | ||
5.2 Lipopolysaccharide (LPS) and the Gram-Negative Cell Envelope | 105 | ||
5.3 The LPS Biosynthesis Pathway | 106 | ||
5.3.1 Kdo2-Lipid A | 106 | ||
5.3.2 Core Polysaccharide | 109 | ||
5.3.3 O-Antigen | 111 | ||
5.4 LPS Modification and Its Role in Gram-Negative Bacterial Persistence | 111 | ||
5.5 LPS Transport: The Lpt Pathway | 112 | ||
5.5.1 Extraction of LPS from the IM | 112 | ||
5.5.2 Traversing the Periplasm: The LptA Protein Bridge | 113 | ||
5.5.3 LPS Insertion into the OM | 114 | ||
5.6 LPS Transport as a New Target for the Development of Gram-Negative Antibiotics | 115 | ||
5.7 Conclusions | 120 | ||
References | 120 | ||
Chapter 6 - The Discovery of Teixobactin | 127 | ||
6.1 Introduction | 127 | ||
6.2 Cultivating the Unculturable – The iChip | 128 | ||
6.3 Teixobactin – A Novel Antibiotic | 129 | ||
6.4 Structural Determination of Teixobactin | 130 | ||
6.5 Synthesis of Teixobactin and Analogues | 135 | ||
6.6 Conclusion | 139 | ||
References | 139 | ||
Chapter 7 - Emerging Targets in Anti-Tubercular Drug Design | 141 | ||
7.1 Introduction | 141 | ||
7.1.1 The Biology and Pathology of TB | 145 | ||
7.1.2 Current Drug Targets | 148 | ||
7.1.2.1 Cell Envelope | 149 | ||
7.1.2.2 Translation | 149 | ||
7.1.2.3 Transcription and DNA Replication | 150 | ||
7.1.3 Resistance Related to TB | 150 | ||
7.1.3.1 Efflux Pumps | 150 | ||
7.1.3.2 Gene Mutations | 151 | ||
7.1.3.2(a)\rMutations or Modifications of Drug Targets.Mutations: Anti-tubercular agents interrupt the cellular metabolism preventing requis... | 151 | ||
7.1.3.2(b)\rInability to Activate Prodrugs.Among the anti-tubercular agents, some prodrugs are found (e.g. INH, PZA, and ETH). These prodrug... | 151 | ||
7.1.3.3 Enzymatic Modifications and Inactivation of Drugs | 152 | ||
7.2 Discovery and Validation of Novel Drug Targets in TB | 153 | ||
7.2.1 Targets Involved in Mycobacterium tuberculosis Cell Envelope Biosynthesis | 153 | ||
7.2.1.1 Mycolic Acid Biosynthesis and Processing | 154 | ||
7.2.1.1(a)\rβ-Ketoacyl-ACP Synthases.There are three β-ketoacyl-ACP synthases in the Mtb FAS II system: FabH, KasA, and KasB (Figure 7.9). F... | 154 | ||
7.2.1.1(b)\rMycobacterial Membrane Protein Large 3 (MmpL3) (Cell Wall Mycolic Acid Transporter).The synthesized mycolic acids from the FAS I... | 157 | ||
7.2.1.1(c) Antigen 85 Complex.The Ag85 complex consists of three homo logous mycolyltransferase enzymes Ag85A/B/C, which catalyze the trans... | 159 | ||
7.2.1.2 Arabinogalactan Biosynthesis | 162 | ||
7.2.1.3 Peptidoglycan Biosynthesis and Maintenance | 163 | ||
7.2.2 Targets Involved in Mycobacterium tuberculosis General Metabolism | 164 | ||
7.2.2.1 ATP Homeostasis | 166 | ||
7.2.2.2 Targets in Amino Acid Biosynthetic Pathways | 167 | ||
7.2.2.2(a)\rTargets in the Shikimate Pathway.The shikimate pathway encompasses seven steps leading to chorismate, a precursor of folic acid,... | 167 | ||
7.2.2.2(b)\rTargets in the Branched-Chain Amino Acid Pathway.A second amino acid biosynthetic pathway to exploit is the branched-chain amino... | 169 | ||
7.2.2.2(c)\rTargets in the Biosynthesis of Lysine and Its Precursors.A third amino acid biosynthetic pathway, which has been investigated an... | 170 | ||
7.2.2.3 Cofactors Biosynthesis | 170 | ||
7.2.2.3(a)\rCoA Biosynthetic Pathway.CoA is an essential cosubstrate for many biosynthetic pathways, including fatty acid biosynthesis, DNA ... | 172 | ||
7.2.2.3(b)\rFolate Biosynthesis.Folate is biosynthesized from GTP (Figure 7.25), which is converted to dihydroneopterin triphosphate with th... | 172 | ||
7.2.2.3(c)\rBiosynthesis of Riboflavin (Vitamin B2).The de novo synthesis of flavin requires one molecule of GTP and two molecules of ribulo... | 174 | ||
7.2.2.3(d) Redox of NADH.The reduction and oxidation of NADH is important for respiration, particularly for Mtb in a non-replicating state... | 176 | ||
7.2.2.4 DNA Synthesis | 178 | ||
7.2.2.4(a)\rDeoxythymidine Triphosphate (dTTP) Generation.Thymidylate kinase (TMPK) catalyzes the transformation of deoxythymine monophospha... | 179 | ||
7.2.2.4(b)\rDNA Primase (DnaG).The bacterial primase DnaG is responsible for synthesizing small RNA oligonucleotides and is essential for ch... | 180 | ||
7.2.2.4(c)\rDNA Ligase.DNA ligase is a highly modular protein that has distinct domains and architecture, and is either ATP or NAD+ dependen... | 180 | ||
7.2.2.5 Other Pathways | 181 | ||
7.2.2.5(a)\rCytochrome Oxidases.A major drawback of the current anti-tubercular drug therapy is the duration of treatment, which is typicall... | 181 | ||
7.2.2.5(b)\rIsocitrate Lyase (ICL).One way to overcome Mtb persistence is to target a pathway that is required for a hostile environment. Wh... | 182 | ||
7.2.2.5(c)\rProtein Tyrosine Phosphatase B (PTPB).Many biological processes in mammalian cells are regulated by protein tyrosine phosphoryla... | 182 | ||
7.2.2.5(d)\rIron Acquisition Mechanism.Iron is a critical nutrient for most organisms due to its function as a cofactor for many enzymatic p... | 185 | ||
7.3 Synergistic Drug Combination Therapy | 186 | ||
7.3.1 Unrelated Compounds | 186 | ||
7.3.2 Inhibitors of Resistance Enzymes | 186 | ||
7.3.3 P2X7 Receptor Agonist | 187 | ||
7.4 Conclusion and Perspectives | 188 | ||
Acknowledgements | 188 | ||
References | 188 | ||
Chapter 8 - Antibacterial Leads Targeting Isoprenoid Biosynthesis | 204 | ||
8.1 Introduction | 204 | ||
8.2 Targeting the MVA Pathway | 207 | ||
8.2.1 Historic Compounds Inhibiting the MVA Pathway | 207 | ||
8.2.2 Screening for Inhibitors of the MVA Pathway in Bacteria | 208 | ||
8.2.3 Targeting the GHMP Kinase Family Members of the MVA Pathway | 209 | ||
8.3 Targeting the MEP Pathway | 211 | ||
8.3.1 Historic Compounds Inhibiting the MEP Pathway | 211 | ||
8.3.2 Inhibiting DXS | 213 | ||
8.3.3 Inhibiting IspC | 217 | ||
8.3.4 Inhibiting IspD | 226 | ||
8.3.5 Inhibiting IspE | 227 | ||
8.3.6 Inhibiting IspF | 231 | ||
8.3.7 Inhibiting IspG and IspH | 234 | ||
8.4 Alternate Targets Utilizing IPP Precursors | 236 | ||
8.4.1 Inhibiting IDI | 236 | ||
8.4.2 Inhibiting UppS | 238 | ||
8.4.3 Inhibiting Staphyloxanthin Biosynthesis | 243 | ||
8.5 Conclusions | 245 | ||
References | 246 | ||
Subject Index | 256 |