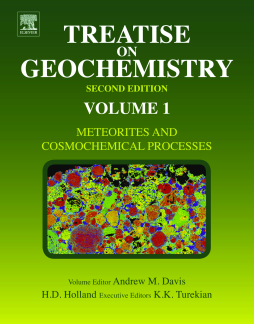
Additional Information
Book Details
Abstract
This extensively updated new edition of the widely acclaimed Treatise on Geochemistry has increased its coverage beyond the wide range of geochemical subject areas in the first edition, with five new volumes which include: the history of the atmosphere, geochemistry of mineral deposits, archaeology and anthropology, organic geochemistry and analytical geochemistry. In addition, the original Volume 1 on "Meteorites, Comets, and Planets" was expanded into two separate volumes dealing with meteorites and planets, respectively. These additions increased the number of volumes in the Treatise from 9 to 15 with the index/appendices volume remaining as the last volume (Volume 16). Each of the original volumes was scrutinized by the appropriate volume editors, with respect to necessary revisions as well as additions and deletions. As a result, 27% were republished without major changes, 66% were revised and 126 new chapters were added.
- In a many-faceted field such as Geochemistry, explaining and understanding how one sub-field relates to another is key. Instructors will find the complete overviews with extensive cross-referencing useful additions to their course packs and students will benefit from the contextual organization of the subject matter
- Six new volumes added and 66% updated from 1st edition. The Editors of this work have taken every measure to include the many suggestions received from readers and ensure comprehensiveness of coverage and added value in this 2nd edition
- The esteemed Board of Volume Editors and Editors-in-Chief worked cohesively to ensure a uniform and consistent approach to the content, which is an amazing accomplishment for a 15-volume work (16 volumes including index volume)!
"This landmark 10-volume publication is a comprehensive review of the many-faceted field of geochemistry. (...)
The Treatise will be an indispensable reference not only to academics but to contamination cleanup professionals, resource managers, and environmental regulators as well." --David W. Morganwalp, U.S. Geological Survey, Reston, VA, USA
Table of Contents
Section Title | Page | Action | Price |
---|---|---|---|
e9780080983004v1.pdf | 1 | ||
Front Cover | 1 | ||
Meteorites and Cosmochemical Processes | 4 | ||
Copyright | 5 | ||
In Memoriam | 7 | ||
Heinrich Dieter Holland (1927–2012) | 9 | ||
Karl Karekin Turekian (1927–2013) | 11 | ||
References | 13 | ||
Dedication | 15 | ||
Contents | 17 | ||
Executive Editors’ Foreword to the Second Edition | 19 | ||
Contributors | 23 | ||
Volume Editor’s Introduction | 25 | ||
References | 26 | ||
Chapter 1.1: Classification of Meteorites and Their Genetic Relationships | 27 | ||
1.1.1. Introduction | 28 | ||
1.1.2. Classification of Chondritic Meteorites | 29 | ||
1.1.2.1. Taxonomy | 29 | ||
1.1.2.2. Primary Classification Parameters | 31 | ||
1.1.2.2.1. Bulk chemical compositions | 31 | ||
1.1.2.2.2. Bulk oxygen isotopic compositions | 32 | ||
1.1.2.2.3. Bulk carbon and nitrogen abundances and isotopic compositions | 32 | ||
1.1.2.2.4. Bulk stable-isotope anomalies | 32 | ||
1.1.2.2.5. Oxidation state | 32 | ||
1.1.2.3. Secondary Classification Parameters | 33 | ||
1.1.2.3.1. Petrologic type | 33 | ||
1.1.2.3.2. Shock metamorphism stages | 35 | ||
1.1.2.3.3. Classification of breccias | 35 | ||
1.1.2.3.4. Degree of terrestrial weathering | 35 | ||
1.1.2.4. Mineralogical and Geochemical Characteristics of Chondrite Groups | 36 | ||
1.1.2.4.1. Carbonaceous chondrites | 36 | ||
1.1.2.4.1.1. CI (Ivuna-like) chondrites | 36 | ||
1.1.2.4.1.2. CM (Mighei-like) chondrites | 36 | ||
1.1.2.4.1.3. Ungrouped CM/CI-like chondrites | 38 | ||
1.1.2.4.1.4. CO (Ornans-like) chondrites | 38 | ||
1.1.2.4.1.5. Ungrouped CO/CM-like chondrites | 38 | ||
1.1.2.4.1.6. CR (Renazzo-like) chondrites | 40 | ||
1.1.2.4.1.7. Ungrouped CR-related chondrites | 40 | ||
1.1.2.4.1.8. CH (ALH 85085-like) chondrites | 40 | ||
1.1.2.4.1.9. CB (Bencubbin-like) chondrites | 40 | ||
1.1.2.4.1.10. CV (Vigarano-like) chondrites | 42 | ||
1.1.2.4.1.11. Ungrouped CV-like chondrites | 45 | ||
1.1.2.4.1.12. CK (Karoonda-like) chondrites | 46 | ||
1.1.2.4.2. Ordinary chondrites and related meteorites | 47 | ||
1.1.2.4.2.1. H, L, and LL chondrites | 47 | ||
1.1.2.4.2.2. Low-FeO ordinary chondrites | 48 | ||
1.1.2.4.2.3. Metal-rich chondrites | 48 | ||
1.1.2.4.3. Enstatite chondrites | 48 | ||
1.1.2.4.4. K (Kakangari-like) chondrites | 50 | ||
1.1.2.4.5. R (Rumuruti-like) chondrites | 51 | ||
1.1.3. Classification of Interplanetary Dust Particles (IDPs) | 51 | ||
1.1.4. Classification of Nonchondritic Meteorites | 51 | ||
1.1.4.1. Winonaites and the Silicate-Bearing IAB Irons | 52 | ||
1.1.4.2. Acapulcoites and Lodranites | 52 | ||
1.1.4.3. Ungrouped primitive achondrites | 55 | ||
1.1.4.3.1. Primitive enstatite achondrites | 55 | ||
1.1.4.3.2. Tafassasset | 56 | ||
1.1.4.4. Brachinites and Brachinite-Like Ultramafic Achondrites | 56 | ||
1.1.4.5. Ungrouped Achondrites | 58 | ||
1.1.4.5.1. Divnoe | 58 | ||
1.1.4.5.2. Ungrouped felsic achondrite | 58 | ||
1.1.4.6. Ureilites | 59 | ||
1.1.4.6.1. Almahata Sitta | 61 | ||
1.1.4.7. Angrites | 61 | ||
1.1.4.8. Aubrites | 62 | ||
1.1.4.9. HED Meteorites: Howardites, Eucrites, and Diogenites | 62 | ||
1.1.4.9.1. Eucrites | 62 | ||
1.1.4.9.2. Diogenites | 64 | ||
1.1.4.9.3. Howardites and other eucrite-diogenite breccias | 64 | ||
1.1.4.9.4. Anomalous eucrites | 64 | ||
1.1.4.9.4.1. Ungrouped eucrites | 66 | ||
1.1.4.9.4.2. Anomalous metal-rich eucrites | 66 | ||
1.1.4.9.4.3. Other anomalous eucrites | 66 | ||
1.1.4.10. Mesosiderites | 67 | ||
1.1.4.11. Pallasites | 68 | ||
1.1.4.11.1. Main-group pallasites | 68 | ||
1.1.4.11.2. Eagle Station pallasite grouplet | 69 | ||
1.1.4.11.3. Other pallasites | 69 | ||
1.1.4.12. Iron Meteorites | 69 | ||
1.1.4.12.1. Chemical groups | 70 | ||
1.1.4.12.2. Groups IAB and IIICD | 72 | ||
1.1.4.12.3. Group IIAB | 73 | ||
1.1.4.12.4. Group IIE | 74 | ||
1.1.4.12.5. Group IIIAB | 75 | ||
1.1.4.12.6. Group IVA | 75 | ||
1.1.4.12.7. Group IVB | 75 | ||
1.1.4.12.8. Ungrouped irons | 75 | ||
1.1.4.13. Martian Meteorites | 75 | ||
1.1.4.13.1. Shergottites | 75 | ||
1.1.4.14. Nakhlites (Clinopyroxenites/Wehrlites) | 78 | ||
1.1.4.14.1. Chassignites (Dunites) | 78 | ||
1.1.4.14.2. Allan Hills 84001 (Orthopyroxenite) | 78 | ||
1.1.4.15. Lunar Meteorites | 78 | ||
1.1.5. Genetic Relations Among Meteorite Groups | 78 | ||
Acknowledgments | 79 | ||
References | 79 | ||
Chapter 1.2: Chondrites and Their Components | 91 | ||
1.2.1. Introduction | 92 | ||
1.2.1.1. What Are Chondrites? | 92 | ||
1.2.1.2. Why Study Chondrites? | 92 | ||
1.2.1.3. Historical Views on Chondrite Origins | 93 | ||
1.2.1.4. Chondrites and the Solar Nebula | 94 | ||
1.2.2. Classification and Parent Bodies of Chondrites | 94 | ||
1.2.2.1. Chondrite Groups, Clans, and Parent Bodies | 94 | ||
1.2.2.2. Ordinary Chondrites | 96 | ||
1.2.2.3. Carbonaceous Chondrites | 96 | ||
1.2.2.4. Enstatite Chondrites | 96 | ||
1.2.3. Bulk Composition of Chondrites | 97 | ||
1.2.3.1. Cosmochemical Classification of Elements | 97 | ||
1.2.3.2. Chemical Compositions of Chondrites | 97 | ||
1.2.3.3. Isotopic Compositions of Chondrites | 98 | ||
1.2.3.4. Oxygen Isotopic Compositions | 99 | ||
1.2.4. Metamorphism, Alteration, and Impact Processing | 99 | ||
1.2.4.1. Petrologic Types | 100 | ||
1.2.4.1.1. Type 1-2 chondrites | 100 | ||
1.2.4.1.2. Type 3 chondrites | 101 | ||
1.2.4.1.3. Type 4-6 chondrites | 101 | ||
1.2.4.2. Thermal History and Modeling | 102 | ||
1.2.4.3. Impact Processing of Chondritic Asteroids | 102 | ||
1.2.5. Chondritic Components | 103 | ||
1.2.5.1. Calcium- and Aluminum-Rich Inclusions | 103 | ||
1.2.5.1.1. Comparison of CAIs from different chondrite groups | 103 | ||
1.2.5.1.2. Oxygen-isotope compositions of CAIs | 104 | ||
1.2.5.2. Forsterite-Rich Accretionary Rims around CAIs | 106 | ||
1.2.5.3. Amoeboid Olivine Aggregates | 108 | ||
1.2.5.3.1. Mineralogy and petrology of AOAs | 108 | ||
1.2.5.3.2. Trace elements and isotopic composition of AOAs• | 109 | ||
1.2.5.3.3. Origin of AOAs | 110 | ||
1.2.5.4. Aluminum-Rich Chondrules | 112 | ||
1.2.5.4.1. Isotopic and trace element studies of aluminum-rich chondrules | 113 | ||
1.2.5.4.2. Origin of aluminum-rich chondrules | 115 | ||
1.2.5.4.3. Relict CAIs and AOAs in ferromagnesian chondrules | 116 | ||
1.2.5.4.4. Relict chondrules in igneous CAIs and igneous CAIs overgrown by chondrule material | 116 | ||
1.2.5.5. Refractory Forsterite-Rich Objects | 119 | ||
1.2.5.6. Chondrules | 121 | ||
1.2.5.6.1. Chondrule textures, types, and thermal histories | 121 | ||
1.2.5.6.2. Chemical compositions of chondrules | 122 | ||
1.2.5.6.3. Oxygen-isotope compositions of chondrules | 122 | ||
1.2.5.6.4. Stable isotope anomalies and aluminum-magnesium isotope systematics of chondrules | 123 | ||
1.2.5.6.5. Noble gas abundances in chondrules | 123 | ||
1.2.5.6.6. Compound chondrules, relict grains, and chondrule rims | 124 | ||
1.2.5.6.7. Chondrule forming environment | 126 | ||
1.2.5.6.8. Chondrules that formed by condensation | 129 | ||
1.2.5.6.9. Chondrules that formed on asteroids | 130 | ||
1.2.5.7. Metal and Troilite | 132 | ||
1.2.5.7.1. CR chondrite metallic Fe,Ni | 133 | ||
1.2.5.7.2. CH and CB chondrite metallic Fe,Ni | 133 | ||
1.2.5.7.3. Troilite | 134 | ||
1.2.5.8. Matrix Material | 134 | ||
1.2.5.8.1. CI1 chondrites | 136 | ||
1.2.5.8.2. CM2 chondrite matrices | 136 | ||
1.2.5.8.3. CR2-3 chondrite matrices | 137 | ||
1.2.5.8.4. CO3 chondrite matrices | 137 | ||
1.2.5.8.5. CK3-6 chondrite matrices | 140 | ||
1.2.5.8.6. CV3 chondrite matrices | 140 | ||
1.2.5.8.7. Matrix of ungrouped C chondrites, Acfer 094, and Adelaide | 141 | ||
1.2.5.8.8. H-L-LL3 chondrite matrices | 142 | ||
1.2.5.8.9. EH3 and EL3 chondrite matrices | 142 | ||
1.2.5.8.10. K3 chondrite matrices (Kakangari and LEW 87232) | 142 | ||
1.2.5.8.11. Matrix-rich lithic clasts | 144 | ||
1.2.5.8.12. Origins of matrix phases | 145 | ||
1.2.6. Formation and Accretion of Chondritic Components | 148 | ||
1.2.7. Heating Mechanisms in the Early Solar System | 148 | ||
1.2.7.1. Nebular Shocks | 150 | ||
1.2.7.2. Jets and Outflows | 150 | ||
1.2.7.3. Impacts on Planetesimals | 150 | ||
Acknowledgments | 151 | ||
References | 151 | ||
Chapter 1.3: Calcium-Aluminum-Rich Inclusions in Chondritic Meteorites | 165 | ||
1.3.1. Introduction | 165 | ||
1.3.2. Changes in this Revision | 166 | ||
1.3.3. Some Essential Terminology: Structural Elements of a CAI | 166 | ||
1.3.3.1. The Generic CAI | 166 | ||
1.3.3.2. Comments on Primary and Secondary Mineralogy | 167 | ||
1.3.3.3. Rim Sequences | 167 | ||
1.3.3.4. Metal Grains and Fremdlinge | 168 | ||
1.3.4. Mineralogy and Mineral Chemistry | 168 | ||
1.3.4.1. Melilite | 170 | ||
1.3.4.2. Spinel | 171 | ||
1.3.4.3. Pyroxene | 171 | ||
1.3.4.4. Hibonite | 172 | ||
1.3.4.5. Perovskite | 173 | ||
1.3.4.6. Grossite (CaAl4O7) and Krotite/Dmitryivanovite (CaAl2O4) | 173 | ||
1.3.4.7. Anorthite | 173 | ||
1.3.5. Diversity and Major Element Bulk Chemistry | 173 | ||
1.3.6. Type C CAIs, Compound Objects, and the Chondrule-CAI Connection | 180 | ||
1.3.7. Fun CAIs and Hibonite Grains | 180 | ||
1.3.8. Distribution Among Chondrite Types | 182 | ||
1.3.8.1. CV3 Chondrites | 183 | ||
1.3.8.2. CO3 Chondrites | 183 | ||
1.3.8.3. CM Chondrites | 183 | ||
1.3.8.4. CR Clan Chondrites | 184 | ||
1.3.8.5. CAIs in Acfer 094 | 185 | ||
1.3.8.6. Ordinary Chondrites | 186 | ||
1.3.8.7. Enstatite Chondrites | 186 | ||
1.3.8.8. Stardust CAIs | 188 | ||
1.3.9. Ages | 188 | ||
1.3.10. Trace Elements | 188 | ||
1.3.11. Oxygen Isotopes | 190 | ||
1.3.11.1. General | 190 | ||
1.3.11.2. The Data | 190 | ||
1.3.11.3. Nebular and Intra-CAI heterogeneity: Progress on Two Great Debates | 193 | ||
1.3.12. Short-Lived Radionuclides in CAIs | 194 | ||
1.3.12.1. 26Al | 194 | ||
1.3.12.2. 41Ca | 198 | ||
1.3.12.3. 10Be | 198 | ||
1.3.13. CAIS, Chondrules, Condensation, and Melt Distillation | 198 | ||
1.3.14. Wark-Lovering Rim Sequences: One Terminal Event or Many? | 199 | ||
1.3.15. Conclusions and Reflections: Technology, the Big Picture, and the Convergence of Cosmochemistry and Astronomy | 199 | ||
Acknowledgments | 199 | ||
References | 200 | ||
Chapter 1.4: Presolar Grains | 207 | ||
1.4.1. Introduction | 207 | ||
1.4.2. Historical Background | 208 | ||
1.4.3. Types of Presolar Grains | 208 | ||
1.4.4. Analysis Techniques | 209 | ||
1.4.5. Astrophysical Implications of the Study of Presolar Grains | 210 | ||
1.4.6. Silicon Carbide | 210 | ||
1.4.6.1. Mainstream Grains | 213 | ||
1.4.6.2. Type Y and Z Grains | 216 | ||
1.4.6.3. Type AB Grains | 217 | ||
1.4.6.4. Type X Grains | 217 | ||
1.4.6.5. Nova Grains | 220 | ||
1.4.6.6. Type C Grains | 220 | ||
1.4.6.7. Abundances and Grain-Size Effect | 220 | ||
1.4.7. Silicon Nitride | 221 | ||
1.4.8. Graphite | 221 | ||
1.4.8.1. Physical Properties | 221 | ||
1.4.8.2. Isotopic Compositions | 222 | ||
1.4.9. Oxygen-Rich Grains | 225 | ||
1.4.9.1. Oxide Grains | 225 | ||
1.4.9.2. Silicate Grains | 228 | ||
1.4.10. Diamond | 230 | ||
1.4.11. Conclusion and Future Prospects | 231 | ||
Acknowledgments | 231 | ||
References | 231 | ||
Chapter 1.5: Structural and Isotopic Analysis of Organic Matter in Carbonaceous Chondrites | 241 | ||
1.5.1. Introduction | 241 | ||
1.5.2. Organic Material in Carbonaceous Chondrites | 242 | ||
1.5.3. Extractable Organic Matter | 242 | ||
1.5.3.1. Abundance and Distribution of Extractable Compounds | 242 | ||
1.5.3.1.1. Carboxylic acids | 242 | ||
1.5.3.1.2. Sulfonic and phosphonic acids | 243 | ||
1.5.3.1.3. Amino acids | 244 | ||
1.5.3.1.4. Aromatic hydrocarbons | 245 | ||
1.5.3.1.5. Heterocyclic compounds | 245 | ||
1.5.3.1.6. Aliphatic hydrocarbons | 246 | ||
1.5.3.1.7. Amines and amides | 246 | ||
1.5.3.1.8. Alcohols, aldehydes, ketones, and sugar-related compounds | 246 | ||
1.5.3.2. Stable-Isotopic Investigations of Classes of Organic Compounds | 247 | ||
1.5.3.3. Compound-Specific Isotopic Studies | 247 | ||
1.5.3.3.1. Carbon | 248 | ||
1.5.3.3.2. Nitrogen | 249 | ||
1.5.3.3.3. Hydrogen | 249 | ||
1.5.3.3.4. Sulfur | 249 | ||
1.5.4. Macromolecular Material | 251 | ||
1.5.4.1. Structural Studies Using Pyrolysis and Chemical Degradation | 251 | ||
1.5.4.2. NMR and Electron Spin Resonance Studies | 251 | ||
1.5.4.3. Stable-Isotopic Studies | 253 | ||
1.5.5. In Situ Examination of Meteoritic Organic Matter | 254 | ||
1.5.6. Environments of Formation | 255 | ||
References | 257 | ||
Chapter 1.6: Achondrites | 261 | ||
1.6.1. Introduction | 261 | ||
1.6.2. Primitive Achondrites | 262 | ||
1.6.2.1. Acapulcoite-Lodranite Clan | 262 | ||
1.6.2.2. Winonaite-IAB-Iron Silicate Inclusion Clan | 272 | ||
1.6.2.3. Zag (b) | 273 | ||
1.6.3. Differentiated Achondrites | 273 | ||
1.6.3.1. Angrites | 273 | ||
1.6.3.2. Aubrites | 276 | ||
1.6.3.3. Brachinites | 277 | ||
1.6.3.4. Howardite-Eucrite-Diogenite Clan | 279 | ||
1.6.3.5. Mesosiderite Silicates | 280 | ||
1.6.3.6. Ureilites | 282 | ||
1.6.3.7. Ibitira | 284 | ||
1.6.3.8. Itqiy | 284 | ||
1.6.3.9. Northwest Africa 011 | 285 | ||
1.6.4. Uncategorized Achondrites | 285 | ||
1.6.4.1. IIE Iron Meteorite Silicates | 285 | ||
1.6.5. Summary | 286 | ||
Acknowledgments | 286 | ||
References | 287 | ||
Chapter 1.7: Iron and Stony-Iron Meteorites | 293 | ||
1.7.1. Introduction | 293 | ||
1.7.2. Classification and Chemical Composition of Iron Meteorites | 294 | ||
1.7.2.1. Group IIAB Iron Meteorites | 295 | ||
1.7.2.2. Group IIIAB Iron Meteorites | 295 | ||
1.7.2.3. Group IVA Iron Meteorites | 295 | ||
1.7.2.4. Group IVB Iron Meteorites | 296 | ||
1.7.2.5. Silicate-Bearing IAB Complex and IIE Iron Meteorites | 296 | ||
1.7.2.6. Mesosiderites | 296 | ||
1.7.2.7. Ungrouped Iron Meteorites | 297 | ||
1.7.3. Accretion and Differences in Bulk Chemistry Between Groups of Iron Meteorites | 297 | ||
1.7.4. Heating and Differentiation | 298 | ||
1.7.5. Fractional Crystallization of Metal Cores | 300 | ||
1.7.5.1. Imperfect Mixing During Crystallization | 301 | ||
1.7.5.2. Late-Stage Crystallization and Immiscible Liquid | 301 | ||
1.7.5.3. The Missing Sulfur-Rich Meteorites | 302 | ||
1.7.6. Cooling Rates and Sizes of Parent Bodies | 303 | ||
1.7.7. Pallasites | 304 | ||
1.7.8. Parent Bodies of Iron and Stony-Iron Meteorites | 305 | ||
1.7.9. Future Research Directions | 306 | ||
References | 307 | ||
Chapter 1.8: Early Solar Nebula Grains - Interplanetary Dust Particles | 313 | ||
1.8.1. Introduction | 313 | ||
1.8.2. Particle Size, Morphology, Porosity, and Density | 315 | ||
1.8.3. Mineralogy | 315 | ||
1.8.3.1. CPIDPs | 316 | ||
1.8.3.2. Glass with Embedded Metal and Sulfides | 320 | ||
1.8.3.3. CSIDPs | 323 | ||
1.8.4. Optical Properties | 324 | ||
1.8.5. Compositions | 325 | ||
1.8.5.1. Major Elements | 325 | ||
1.8.5.2. Trace Elements | 327 | ||
1.8.5.3. Isotopes | 329 | ||
1.8.5.4. Noble Gases | 330 | ||
1.8.6. Conclusions | 330 | ||
Acknowledgments | 332 | ||
References | 332 | ||
Chapter 1.9: Nebular Versus Parent Body Processing | 335 | ||
1.9.1. Introduction | 335 | ||
1.9.2. Nebular or Asteroidal Processing: Some Criteria | 336 | ||
1.9.3. Aqueous Alteration | 336 | ||
1.9.3.1. CI Carbonaceous Chondrites | 336 | ||
1.9.3.1.1. Evidence for asteroidal alteration | 336 | ||
1.9.3.1.2. Timing of alteration | 337 | ||
1.9.3.1.3. Summary | 338 | ||
1.9.3.2. CM Carbonaceous Chondrites | 338 | ||
1.9.3.2.1. Timing of alteration | 339 | ||
1.9.3.2.2. Appraisal of the evidence for preaccretionary alteration | 340 | ||
1.9.3.2.3. Evidence for asteroidal alteration | 341 | ||
1.9.3.2.3.1. Veining in CM chondrites | 341 | ||
1.9.3.2.3.2. Fe-rich aureoles | 341 | ||
1.9.3.2.3.3. Bulk compositional homogeneity of CM chondrites | 341 | ||
1.9.3.2.3.4. Elemental exchange between chondrules and matrix during progressive alteration | 342 | ||
1.9.3.2.3.5. Homogeneity of chondrule alteration | 342 | ||
1.9.3.2.3.6. Presence of oriented aragonite clusters in CM chondrites | 342 | ||
1.9.3.2.3.7. Microchemical environments | 342 | ||
1.9.3.2.3.8. Oxygen isotopic compositions | 343 | ||
1.9.3.2.4. Summary | 343 | ||
1.9.3.3. CR Carbonaceous Chondrites | 343 | ||
1.9.3.4. CO Carbonaceous Chondrites | 344 | ||
1.9.3.5. CV Carbonaceous Chondrites | 345 | ||
1.9.3.5.1. Evidence for preaccretionary alteration | 346 | ||
1.9.3.5.2. Evidence for parent body alteration | 346 | ||
1.9.3.6. Unequilibrated Ordinary Chondrites | 346 | ||
1.9.4. Oxidation and Metasomatism | 347 | ||
1.9.4.1. CV Carbonaceous Chondrites | 347 | ||
1.9.4.1.1. Iron-alkali-halogen metasomatism | 347 | ||
1.9.4.1.2. Fayalitic rims on chondrules, CAIs, etc. | 348 | ||
1.9.4.1.3. Oxidation and sulfidization of opaque assemblages | 348 | ||
1.9.4.2. Dark Inclusions in CV Chondrites | 348 | ||
1.9.4.3. Nebular and Parent Body Alteration Models for CV Chondrites | 348 | ||
1.9.4.3.1. Nebular alteration | 348 | ||
1.9.4.3.2. Parent body alteration | 349 | ||
1.9.4.3.3. Problems with the nebular alteration model | 349 | ||
1.9.4.3.3.1. Origin of platy matrix olivine grains | 349 | ||
1.9.4.3.3.2. Presence of veins in dark inclusions | 349 | ||
1.9.4.3.3.3. Formation of calcium-iron pyroxenes | 349 | ||
1.9.4.3.3.4. Rims of calcium-iron pyroxene around dark inclusions | 350 | ||
1.9.4.3.3.5. Calcium-rich halos around altered CAIs | 350 | ||
1.9.4.3.3.6. Timing of alteration | 350 | ||
1.9.4.3.4. Problems with the asteroidal alteration model | 351 | ||
1.9.4.3.4.1. Oxygen isotopes | 351 | ||
1.9.4.3.4.2. Formation of platy olivines | 351 | ||
1.9.4.3.4.3. Timing of metasomatism and aqueous alteration | 351 | ||
1.9.4.4. CO Carbonaceous Chondrites | 352 | ||
1.9.4.4.1. Evidence for parent body alteration of CO chondrites | 353 | ||
1.9.4.4.2. Evidence for nebular alteration of CO chondrites | 354 | ||
1.9.4.5. Unequilibrated Ordinary Chondrites | 354 | ||
1.9.4.5.1. Carbide-magnetite assemblages | 354 | ||
1.9.5. Future Work | 355 | ||
Acknowledgments | 355 | ||
References | 355 | ||
Chapter 1.10: Condensation and Evaporation of Solar System Materials | 361 | ||
1.10.1. Introduction | 361 | ||
1.10.2. Theoretical Framework | 362 | ||
1.10.2.1. Thermodynamic Equilibrium | 363 | ||
1.10.2.1.1. Condensation of the major elements | 363 | ||
1.10.2.1.2. Condensation of trace elements | 364 | ||
1.10.2.1.3. High-temperature equilibrium isotopic fractionation | 365 | ||
1.10.2.2. Kinetic Effects | 365 | ||
1.10.3. Laboratory Experiments | 368 | ||
1.10.3.1. Evaporation of Metals and Simple Oxides | 368 | ||
1.10.3.2. Evaporation of Olivine | 369 | ||
1.10.3.3. Evaporation of CMAS Melts | 369 | ||
1.10.3.4. Evaporation of Chondritic Meteorites and Chondritic Melts | 373 | ||
1.10.3.5. Kinetic Isotope Fractionation Factors for Molten Oxides and Silicates | 373 | ||
1.10.4. Applications | 375 | ||
1.10.4.1. Bulk Compositions of Planets and Meteorite Parent Bodies | 375 | ||
1.10.4.2. Calcium- and Aluminum-Rich Inclusions | 376 | ||
1.10.4.2.1. Ultrarefractory inclusions | 376 | ||
1.10.4.2.2. Textural evidence | 377 | ||
1.10.4.2.3. Bulk elemental and isotopic compositions of type B CAIs | 377 | ||
1.10.4.2.4. Reheating mechanisms for the type B CAIs | 379 | ||
1.10.4.2.5. Isotopic fractionation of refractory elements in CAIs | 380 | ||
1.10.4.2.6. Refractory metal inclusions | 380 | ||
1.10.4.2.7. FUN CAIs | 381 | ||
1.10.4.2.8. Hibonites with cerium anomalies | 381 | ||
1.10.4.3. Chondrites | 381 | ||
1.10.4.3.1. Chondrules | 381 | ||
1.10.4.3.2. Metal Grains | 382 | ||
1.10.4.4. Deep-Sea Spherules | 382 | ||
1.10.5. Outlook | 382 | ||
Acknowledgments | 382 | ||
References | 382 | ||
Chapter 1.11: Short-Lived Radionuclides and Early Solar System Chronology | 387 | ||
1.11.1. Introduction | 387 | ||
1.11.1.1. Chondritic Meteorites as Probes of Early Solar System Evolution | 387 | ||
1.11.1.2. Short-Lived Radioactivity at the Origin of the Solar System | 388 | ||
1.11.1.3. A Brief History and the Scope of the Present Review | 388 | ||
1.11.2. Dating with Ancient Radioactivity | 390 | ||
1.11.3 ‘Absolute’ and ‘Relative’ Timescales | 391 | ||
1.11.3.1. An Absolute Timescale for Solar System Formation | 391 | ||
1.11.3.2. An Absolute Timescale for Chondrule Formation | 393 | ||
1.11.3.3. An Absolute Timescale for Early Differentiation of Planetesimals | 394 | ||
1.11.4. The Record of Short-Lived Radionuclides in Early Solar System Materials | 394 | ||
1.11.4.1. Beryllium-7 | 395 | ||
1.11.4.2. Calcium-41 | 395 | ||
1.11.4.3. Chlorine-36 | 396 | ||
1.11.4.4. Aluminum-26 | 396 | ||
1.11.4.4.1. Bulk and internal isochrons and the solar system initial 26Al/27Al | 396 | ||
1.11.4.4.2. Mass fractionation correction | 397 | ||
1.11.4.4.3. Magnesium isotopic evolution in the early solar system | 398 | ||
1.11.4.4.4. 26Al-26Mg systematics in CAIs | 399 | ||
1.11.4.4.5. 26Al-26Mg systematics in chondrules | 400 | ||
1.11.4.4.6. 26Al-26Mg systematics in achondrites | 401 | ||
1.11.4.5. Beryllium-10 | 401 | ||
1.11.4.6. Cesium-135 | 402 | ||
1.11.4.7. Iron-60 | 402 | ||
1.11.4.8. Manganese-53 | 404 | ||
1.11.4.9. Palladium-107 | 406 | ||
1.11.4.10. Hafnium-182 | 406 | ||
1.11.4.11. Curium-247 | 409 | ||
1.11.4.12. Iodine-129 | 409 | ||
1.11.4.13. Lead-205 | 409 | ||
1.11.4.14. Niobium-92 | 410 | ||
1.11.4.15. Samarium-146 | 410 | ||
1.11.4.16. Plutonium-244 | 410 | ||
1.11.5. Origins of the Short-Lived Nuclides | 410 | ||
1.11.5.1. Sources of Short-Lived Nuclides | 410 | ||
1.11.6. Short-Lived Nuclides as Chronometers | 411 | ||
1.11.6.1. Formation Timescales of Nebular Materials | 412 | ||
1.11.6.2. Timescales of Planetesimal Accretion and Early Chemical Differentiation | 413 | ||
1.11.7. Conclusions | 413 | ||
1.11.7.1. Implications for Solar Nebula Origin and Evolution | 414 | ||
1.11.7.2. Future Directions | 415 | ||
Acknowledgments | 415 | ||
References | 415 | ||
Chapter 1.12: Solar System Time Scales from Long-Lived Radioisotopes in Meteorites and Planetary Materials | 423 | ||
1.12.1. Introduction | 423 | ||
1.12.1.1. Basic Principles | 423 | ||
1.12.1.2. Application to Meteorites and Planetary Materials: A Historical Perspective and Some New Developments | 424 | ||
1.12.2. Chondrites and Their Components | 425 | ||
1.12.2.1. Formation Ages of Chondritic Components | 425 | ||
1.12.2.1.1. Calcium-aluminum-rich inclusions | 425 | ||
1.12.2.1.2. Chondrules | 427 | ||
1.12.2.2. Ages of Secondary Events Recorded in Chondrites | 427 | ||
1.12.2.2.1. Aqueous alteration | 427 | ||
1.12.2.2.2. Thermal metamorphism | 428 | ||
1.12.2.2.3. Shock metamorphism | 429 | ||
1.12.3. Differentiated Meteorites | 429 | ||
1.12.3.1. Primitive Achondrites: Timing of Incipient Differentiation on Planetesimals | 429 | ||
1.12.3.2. Basaltic and Other Achondrites: Timing of Asteroidal Differentiation and Cataclysm | 430 | ||
1.12.3.2.1. Crust formation time scales from chronology of achondrites and their components | 430 | ||
1.12.3.2.2. Global differentiation time scales based on whole-rock isochrons and initial 87S/86Sr | 432 | ||
1.12.3.2.3. Inner solar system bombardment history based on reset ages | 434 | ||
1.12.3.3. Iron Meteorites and Pallasites: Time Scales of Core Crystallization on Planetesimals | 434 | ||
1.12.4. Planetary Materials | 436 | ||
1.12.4.1. Timing of Lunar Differentiation and Cataclysm from Chronology of Lunar Samples | 437 | ||
1.12.4.1.1. Lunar differentiation history | 437 | ||
1.12.4.1.2. Lunar bombardment history | 438 | ||
1.12.4.2. Time Scales for the Evolution of Mars from Chronology of Martian Meteorites | 438 | ||
1.12.5. Conclusions | 439 | ||
1.12.5.1. A Timeline for Solar System Events | 439 | ||
1.12.5.2. Outlook and Future Prospects | 440 | ||
Acknowledgments | 440 | ||
References | 441 | ||
Chapter 1.13: Cosmic-Ray Exposure Ages of Meteorites | 445 | ||
1.13.1. Introduction | 446 | ||
1.13.2. Calculation of Exposure Ages | 447 | ||
1.13.2.1. Basic Equations | 447 | ||
1.13.2.2. Factors Influencing Production Rates | 447 | ||
1.13.2.3. Measurement Units and Quantities | 448 | ||
1.13.2.4. Determination of Production Rates | 448 | ||
1.13.2.4.1. Radionuclide production rates | 448 | ||
1.13.2.4.2. 26Al and 21Ne | 449 | ||
1.13.2.4.3. 83Kr/81Kr calibration | 449 | ||
1.13.2.5. Equations for Calculating One-Stage CRE Ages | 450 | ||
1.13.2.5.1. 21Ne-22Ne/21Ne ages | 450 | ||
1.13.2.5.2. 38Ar-22Ne/21Ne ages | 450 | ||
1.13.2.5.3. 3He ages | 451 | ||
1.13.2.5.4. 36Cl/ 36Ar ages | 451 | ||
1.13.2.5.5. 81Kr/Kr ages | 452 | ||
1.13.2.5.6. 40K/K ages of meteoritic metal | 452 | ||
1.13.2.6. The Importance of Half-Lives | 453 | ||
1.13.2.7. CRE Ages and Long-Lived Chronometers | 454 | ||
1.13.3. Carbonaceous Chondrites | 454 | ||
1.13.3.1. CI, CM, CO, CV, and CK Chondrites | 454 | ||
1.13.3.2. The CR Clan | 455 | ||
1.13.4. H Chondrites | 456 | ||
1.13.5. L Chondrites | 456 | ||
1.13.6. LL Chondrites | 457 | ||
1.13.7. E Chondrites | 457 | ||
1.13.8. R Chondrites | 458 | ||
1.13.9. Lodranites and Acapulcoites | 458 | ||
1.13.10. Lunar Meteorites | 459 | ||
1.13.10.1. Overview | 459 | ||
1.13.10.2. Construction of CRE Histories | 459 | ||
1.13.10.3. Production Rate of Lunar Meteorites | 461 | ||
1.13.11. Howardite-Eucrite-Diogenite (HED) Meteorites | 461 | ||
1.13.11.1. Eucrites | 461 | ||
1.13.11.2. Diogenites | 462 | ||
1.13.11.3. Howardites | 462 | ||
1.13.11.4. Kapoeta | 463 | ||
1.13.12. Angrites | 463 | ||
1.13.13. Ureilites | 463 | ||
1.13.14. Aubrites (Enstatite Achondrites) | 464 | ||
1.13.15. Brachinites | 465 | ||
1.13.16. Martian Meteorites | 465 | ||
1.13.17. Mesosiderites | 467 | ||
1.13.18. Pallasites | 468 | ||
1.13.19. Irons | 468 | ||
1.13.20. The Smallest Particles: Micrometeorites, Interplanetary Dust Particles, and Interstellar Grains | 470 | ||
1.13.20.1. Background | 470 | ||
1.13.20.2. Exposure Histories from Noble Gases | 470 | ||
1.13.20.3. Exposure Histories from 10Be and 26Al | 470 | ||
1.13.20.4. Interstellar (Presolar) Grains | 471 | ||
1.13.21. Conclusions | 472 | ||
Acknowledgments | 473 | ||
References | 473 | ||
e9780080983004v2 | 481 | ||
Front Cover | 481 | ||
Planets, Asteroids, Comets and the Solar System | 484 | ||
Copyright | 485 | ||
In Memoriam | 486 | ||
Heinrich Dieter Holland (1927–2012) | 488 | ||
Karl Karekin Turekian (1927–2013) | 490 | ||
References | 492 | ||
Dedication | 494 | ||
Contents | 496 | ||
Executive Editors’ Foreword to the Second Edition | 498 | ||
Contributors | 502 | ||
Volume editor’s Introduction | 504 | ||
References | 505 | ||
Chapter 2.1: Origin of the Elements | 506 | ||
2.1.1. Introduction | 506 | ||
2.1.2. Abundances and Nucleosynthesis | 507 | ||
2.1.3. IMS: Evolution and Nucleosynthesis | 508 | ||
2.1.3.1. Shell Helium Burning and 12C Production | 509 | ||
2.1.3.2. s-Process Synthesis in Red Giants | 509 | ||
2.1.4. Massive Star Evolution and Nucleosynthesis | 509 | ||
2.1.4.1. Nucleosynthesis in Massive Stars | 511 | ||
2.1.4.1.1. Hydrogen burning | 511 | ||
2.1.4.1.2. Helium burning and the s-process | 511 | ||
2.1.4.1.3. Hydrogen and helium shell burning | 512 | ||
2.1.4.1.4. Carbon burning | 512 | ||
2.1.4.1.5. Neon and oxygen burning | 512 | ||
2.1.4.1.6. Silicon burning | 512 | ||
2.1.4.1.7. Explosive nucleosynthesis | 512 | ||
2.1.4.1.8. The p-process | 513 | ||
2.1.4.1.9. The r-process | 514 | ||
2.1.5. Type Ia Supernovae: Progenitors and Nucleosynthesis | 514 | ||
2.1.6. Nucleosynthesis and Galactic Chemical Evolution | 515 | ||
References | 517 | ||
Chapter 2.2: Solar System Abu | 520 | ||
2.2.1. Abundances of the Elements in the Solar Nebula | 520 | ||
2.2.1.1. Historical Remarks | 520 | ||
2.2.1.2. Solar System Abundances of the Elements | 521 | ||
2.2.1.2.1. Is the solar nebula compositionally uniform? | 521 | ||
2.2.1.2.2. The composition of the solar photosphere | 522 | ||
2.2.1.3. Abundances of Elements in Meteorites | 524 | ||
2.2.1.3.1. Differentiated and undifferentiated meteorites | 524 | ||
2.2.1.3.2. Cosmochemical classification of elements | 524 | ||
2.2.1.4. CI Chondrites as the Standard for Solar Abundances | 526 | ||
2.2.1.4.1. Chemical variations among chondritic meteorites | 526 | ||
2.2.1.4.2. CI chondrites | 529 | ||
2.2.1.4.3. The CI chondrite abundance table | 530 | ||
2.2.1.5. Solar System Abundances of the Elements | 533 | ||
2.2.1.5.1. Comparison of meteorite and solar a& | 533 | ||
2.2.1.5.2. Solar system abundances versus mass number | 535 | ||
2.2.1.5.3. Other sources for solar system abundances | 535 | ||
2.2.2. The Abundances of the Elements in the ISM | 536 | ||
2.2.2.1. Introduction | 536 | ||
2.2.2.2. The Nature of the ISM | 536 | ||
2.2.2.3. The Chemical Composition of the ISM | 537 | ||
2.2.2.3.1. The composition of the interstellar gas and elemental depletions | 537 | ||
2.2.2.3.2. The composition of interstellar dust | 537 | ||
2.2.2.3.3. Interstellar oxygen problems | 538 | ||
2.2.3. Summary | 539 | ||
References | 539 | ||
Chapter 2.3: The Solar Nebula | 542 | ||
2.3.1. Introduction | 542 | ||
2.3.2. Formation of the Solar Nebula | 543 | ||
2.3.2.1. Observations of Precollapse Clouds | 543 | ||
2.3.2.1.1. Cloud properties | 543 | ||
2.3.2.1.1.1. Density profiles | 544 | ||
2.3.2.1.1.2. Cloud shapes | 544 | ||
2.3.2.1.1.3. Angular momentum distributions | 544 | ||
2.3.2.1.2. Onset of collapse phase | 544 | ||
2.3.2.1.2.1. Ambipolar diffusion and loss of magnetic support | 544 | ||
2.3.2.1.2.2. Shock-triggered collapse | 545 | ||
2.3.2.1.3. Outcome of collapse phase | 545 | ||
2.3.2.1.3.1. Fragmentation leading to ejection of single stars | 545 | ||
2.3.2.1.3.2. Collapse leading to single star formation | 546 | ||
2.3.2.1.4. Ubiquity of bipolar outflows from earliest phases | 546 | ||
2.3.2.1.5. The chemistry of infall and outflows | 546 | ||
2.3.3. Solar Nebula Structure and Evolution | 547 | ||
2.3.3.1. Search for Astrophysical Analogues for the Solar Nebula | 547 | ||
2.3.3.1.1. Optical and IR-wave observations | 547 | ||
2.3.3.1.1.1. Mass accretion rates – episodicity and outbursts | 547 | ||
2.3.3.1.1.2. Temperature profiles from spectral energy distributions | 548 | ||
2.3.3.1.2. Millimeter-wave observations | 548 | ||
2.3.3.1.2.1. Disk masses | 549 | ||
2.3.3.1.2.2. Keplerian rotation | 549 | ||
2.3.3.2. Angular Momentum Transport Mechanisms in Protoplanetary Disks | 549 | ||
2.3.3.2.1. Magnetorotational-driven turbulence | 549 | ||
2.3.3.2.1.1. Balbus–Hawley instability | 550 | ||
2.3.3.2.1.2. Ionization structure and layered accretion | 550 | ||
2.3.3.2.2. Gravitational torques in a marginally unstable disk | 550 | ||
2.3.3.2.2.1. Rapid mass and angular momentum transport | 551 | ||
2.3.3.2.2.2. Global process versus local viscosity | 551 | ||
2.3.3.3. Evolution of the Solar Nebula | 551 | ||
2.3.3.3.1. Viscous accretion disk models | 551 | ||
2.3.3.3.2. Turbulent and gas drag transport | 553 | ||
2.3.3.3.3. Volatility patterns and transport in inner solar system | 553 | ||
2.3.3.4. Clump Formation in a Marginally Gravitationally Unstable Disk | 554 | ||
2.3.3.4.1. Chondrule formation in nebular shock fronts | 554 | ||
2.3.3.4.2. Mixing processes in marginally gravitationally unstable disks | 554 | ||
2.3.4. Solar Nebula Removal | 555 | ||
2.3.4.1. Observational Constraints on Disk Lifetimes | 555 | ||
2.3.4.2. Removal Mechanisms | 555 | ||
2.3.4.2.1. Inward flow onto protosun | 555 | ||
2.3.4.2.2. UV photoevaporation by protosun | 555 | ||
2.3.4.2.3. UV photoevaporation in Orion-like regions | 556 | ||
2.3.4.2.4. Final scouring by widened stellar outflow | 556 | ||
2.3.5. Summary | 556 | ||
References | 556 | ||
Chapter 2.4: Planet Formation | 560 | ||
2.4.1. Introduction | 560 | ||
2.4.2. The Protoplanetary Nebula and the First Solids | 561 | ||
2.4.2.1. Protoplanetary Disks | 561 | ||
2.4.2.2. Disk Evolution | 561 | ||
2.4.2.3. The Solar Nebula | 562 | ||
2.4.3. Planetesimals and the First Solids | 562 | ||
2.4.3.1. Particle Motions | 562 | ||
2.4.3.2. Chondritic Meteorites and their Components | 563 | ||
2.4.3.3. Short-Lived Isotopes | 564 | ||
2.4.3.4. Planetesimal Formation | 564 | ||
2.4.4. Terrestrial Planet Formation | 565 | ||
2.4.4.1. Runaway and Oligarchic Growth | 565 | ||
2.4.4.2. Late-Stage Accretion and Giant Impacts | 566 | ||
2.4.4.3. Planetary Compositions and Core Formation | 566 | ||
2.4.4.4. Formation of the Moon | 567 | ||
2.4.4.5. Water and the Terrestrial Planets | 567 | ||
2.4.5. The Asteroid Belt | 568 | ||
2.4.5.1. Formation of the Asteroid Belt | 568 | ||
2.4.5.2. Asteroid Physical Evolution | 569 | ||
2.4.6. Giant-Planet Formation | 569 | ||
2.4.6.1. Giant-Planet Compositions | 569 | ||
2.4.6.2. Formation of Gas Giant Planets | 569 | ||
2.4.6.3. Formation of Uranus and Neptune | 570 | ||
2.4.6.4. Planetary Migration and Scattering | 570 | ||
2.4.6.5. Planetesimal-Driven Migration and the Nice Model | 571 | ||
References | 572 | ||
Chapter 2.5: The Geochemistry and Cosmochemistry of Impacts | 578 | ||
2.5.1. Introduction: The Use of Geochemistry in Impact Studies | 578 | ||
2.5.2. Background on Impact Craters and Processes | 579 | ||
2.5.3. Methods | 584 | ||
2.5.3.1. General Geochemistry: Major and Trace Elements | 584 | ||
2.5.3.1.1. Sample preparation | 585 | ||
2.5.3.2. Rb–Sr, Sm–Nd, and Pb Isotopes | 585 | ||
2.5.3.3. Siderophile Element Studies | 586 | ||
2.5.3.4. Osmium Isotopes | 588 | ||
2.5.3.5. Chromium Isotopes | 590 | ||
2.5.3.6. Tungsten Isotopes | 591 | ||
2.5.3.7. Stable Isotopes | 591 | ||
2.5.3.8. Other Methods | 592 | ||
2.5.3.8.1. Helium-3 | 592 | ||
2.5.3.8.2. Beryllium-10 | 593 | ||
2.5.3.8.3. Remote sensing | 593 | ||
2.5.3.8.4. Chromian spinels | 593 | ||
2.5.3.8.5. x-Ray absorption near-edge spectroscopy | 593 | ||
2.5.4. Examples | 595 | ||
2.5.4.1. Meteorite Craters: Source Rocks and Impactites | 595 | ||
2.5.4.2. Extraterrestrial Components in Impactites | 596 | ||
2.5.4.2.1. Vredefort impact structure (South Africa) | 599 | ||
2.5.4.2.2. Morokweng impact structure (South Africa) | 600 | ||
2.5.4.2.3. Bosumtwi crater, Ghana | 601 | ||
2.5.4.2.4. Other examples | 602 | ||
2.5.4.3. Tektites | 603 | ||
2.5.4.4. Libyan Desert Glass | 606 | ||
2.5.4.5. K–T Boundary | 607 | ||
2.5.4.6. Permian–Triassic Boundary | 609 | ||
2.5.4.7. Early Archean Spherule Layers | 610 | ||
2.5.4.8. The Earth's Earliest Impact History | 612 | ||
2.5.5. Summary | 614 | ||
Acknowledgments | 615 | ||
References | 615 | ||
Chapter 2.6: Mercury | 624 | ||
2.6.1. Introduction: The Importance of Mercury | 624 | ||
2.6.2. Pre-MESSENGER View of the Chemical Composition of Mercury | 624 | ||
2.6.3. Pre-MESSENGER Models for the Origin of Mercury | 625 | ||
2.6.3.1. Physical Models for Metal Enrichment in Mercury | 625 | ||
2.6.3.2. High-Temperature Evaporation and Condensation Models for Mercury | 625 | ||
2.6.3.3. Refractory-Volatile Mixtures | 626 | ||
2.6.3.4. Formation of Mercury from Known Chondritic Meteorites | 626 | ||
2.6.4. Results from the MESSENGER Mission | 626 | ||
2.6.5. Evaluating Models for the Origin of Mercury | 629 | ||
2.6.6. The Future for the Exploration of Mercury | 629 | ||
Acknowledgments | 630 | ||
References | 630 | ||
Chapter 2.7: Venus | 632 | ||
2.7.1. Brief History of Observations | 632 | ||
2.7.1.1. Pre-Twentieth Century | 632 | ||
2.7.1.2. The Twentieth Century to the Present Day | 632 | ||
2.7.2. Overview of Important Orbital Properties | 634 | ||
2.7.3. Atmosphere | 635 | ||
2.7.3.1. Composition | 635 | ||
2.7.3.1.1. Basic definitions and general remarks | 635 | ||
2.7.3.1.2. Carbon, sulfur, and halogen gases | 635 | ||
2.7.3.1.3. Water vapor | 639 | ||
2.7.3.1.4. Nitrogen and noble gases | 639 | ||
2.7.3.1.5. Isotopic composition | 640 | ||
2.7.3.2. Thermal Structure and Greenhouse Effect | 641 | ||
2.7.3.3. Clouds and Photochemical Cycles | 641 | ||
2.7.3.4. Atmospheric Dynamics | 643 | ||
2.7.3.5. Upper Atmosphere and Solar Wind Interactions | 643 | ||
2.7.4. Surface and Interior | 643 | ||
2.7.4.1. Geochemistry and Mineralogy | 643 | ||
2.7.4.2. Atmosphere–Surface Interactions | 645 | ||
2.7.4.2.1. Carbonate–silicate equilibria | 646 | ||
2.7.4.2.2. Equilibria involving HCl and HF | 646 | ||
2.7.4.2.3. Redox reactions involving Fe-bearing minerals | 647 | ||
2.7.4.2.4. Minerals present in low radar emissivity regions | 647 | ||
2.7.4.3. The Venus Sulfur Cycle and Climate Change | 647 | ||
2.7.4.4. Topography and Geology | 648 | ||
2.7.4.5. Interior | 649 | ||
2.7.5. Summary of Key Questions | 650 | ||
Acknowledgments | 651 | ||
References | 651 | ||
Chapter 2.8: The Origin and Earliest History of the Earth | 654 | ||
2.8.1. Introduction | 655 | ||
2.8.2. Observational Evidence and Theoretical Constraints Pertaining to the Nebular Environment from Which Earth Originated | 655 | ||
2.8.2.1. Circumstellar Disks and the Solar Nebula | 655 | ||
2.8.2.2. Earth-Like and Jupiter-Like Planets | 656 | ||
2.8.2.3. Longevity of the Solar Nebula | 657 | ||
2.8.2.4. Solar Mass Stars and Heating of the Inner Disk | 658 | ||
2.8.3. The Dynamics of Accretion of the Earth | 660 | ||
2.8.3.1. Introduction | 660 | ||
2.8.3.2. Starting Accretion: Settling and Sticking of Dust at 1AU | 660 | ||
2.8.3.3. Starting Accretion: Gravitational Instabilities | 661 | ||
2.8.3.4. Runaway Growth | 661 | ||
2.8.3.5. Migration | 661 | ||
2.8.3.5.1. The Nice model | 661 | ||
2.8.3.5.2. The Grand Tack hypothesis | 662 | ||
2.8.3.6. Larger Collisions | 662 | ||
2.8.4. Chemical and Isotopic Constraints on the Nature of the Components That Accreted to Form the Earth | 663 | ||
2.8.4.1. Chondrites and the Composition of the Disk from Which Earth Accreted | 663 | ||
2.8.4.2. Chondritic Component Models | 665 | ||
2.8.4.3. Nonchondritic Refractory Lithophile Elements | 666 | ||
2.8.4.4. Nonchondritic Moderately Volatile Lithophile Elements | 666 | ||
2.8.4.5. Oxygen Isotopic Models and Volatile Losses | 668 | ||
2.8.4.6. Compositional Models Based on Simple Theoretical Components | 669 | ||
2.8.4.7. The `Hot Nebula´ Model | 669 | ||
2.8.4.8. The `Hot Nebula´ Model and Heterogeneous Accretion | 671 | ||
2.8.4.9. Nonradiogenic Isotopic Differences Between Silicate Reservoirs and Chondrites | 672 | ||
2.8.4.9.1. Nucleosynthetic variations in nonvolatile elements | 672 | ||
2.8.4.9.2. Mass-dependent fractionations in strongly lithophile elements | 672 | ||
2.8.4.9.3. Mass-dependent fractionations in siderophile elements | 673 | ||
2.8.5. Core Formation | 674 | ||
2.8.5.1. Introduction | 674 | ||
2.8.5.2. Magma Oceans | 674 | ||
2.8.5.3. Early Core Growth | 675 | ||
2.8.5.4. Core Formation Mechanisms | 676 | ||
2.8.6. Lead and Tungsten Isotopes and the Timing, Rates, and Mechanisms of Accretion and Core Formation | 677 | ||
2.8.6.1. Introduction: Uses and Abuses of Isotopic Models | 677 | ||
2.8.6.2. Lead Isotopes | 679 | ||
2.8.6.3. Tungsten Isotopes | 680 | ||
2.8.7. Earth's Earliest Atmospheres and Hydrospheres | 685 | ||
2.8.7.1. Introduction | 685 | ||
2.8.7.2. Did Earth Once Have a Nebular Protoatmosphere? | 685 | ||
2.8.7.3. Earth's Degassed Protoatmosphere | 687 | ||
2.8.7.4. Loss of Earth's Earliest Atmosphere(s) | 689 | ||
2.8.8. The Formation of the Moon | 691 | ||
2.8.8.1. Background to Theories | 691 | ||
2.8.8.2. Isotopic Evidence That the Moon Formed Late | 692 | ||
2.8.8.3. Poorly Understood Facets of the Giant Impact Theory | 692 | ||
2.8.8.4. A More Exact Age of the Moon | 694 | ||
2.8.9. Mass Loss and Compositional Changes During Accretion | 695 | ||
2.8.10. The Late Veneer | 696 | ||
2.8.10.1. The Evidence for the Late Veneer | 696 | ||
2.8.10.2. A Volatile-Depleted Late Veneer | 698 | ||
2.8.10.3. Water and the Late Veneer | 698 | ||
2.8.10.4. Earth–Moon Isotopic Similarities and the Late Veneer | 699 | ||
2.8.11. Early Mantle and Crust | 700 | ||
2.8.11.1. Introduction | 700 | ||
2.8.11.2. Earth's Hadean Environment | 700 | ||
2.8.11.3. Earth’s Hadean Crust – The Zircon Archive | 701 | ||
2.8.11.4. Hafnium Isotopes | 702 | ||
2.8.11.5. Early Mantle Heterogeneity Recorded in the 146Sm–142Nd and 182Hf–182W Systems | 703 | ||
Acknowledgments | 704 | ||
References | 704 | ||
Chapter 2.9: The Moon | 718 | ||
2.9.1. Introduction: The Lunar Context | 718 | ||
2.9.2. The Lunar Geochemical Database | 719 | ||
2.9.2.1. Artificially Acquired Samples | 719 | ||
2.9.2.2. Lunar Meteorites | 719 | ||
2.9.2.3. Remote-Sensing Data | 720 | ||
2.9.3. Mare Volcanism | 721 | ||
2.9.3.1. Classification of Mare Rocks | 721 | ||
2.9.3.2. Chronology and Styles of Mare Volcanism | 723 | ||
2.9.3.3. Mare Basalt Trace Element and Isotopic Trends | 729 | ||
2.9.4. The Highland Crust: Impact Bombardment and Early Differentiation | 732 | ||
2.9.4.1. Polymict Breccias and the KREEP Component | 732 | ||
2.9.4.2. Bombardment History of the Moon | 734 | ||
2.9.4.3. Impactor Residues: Siderophile and Fragmental | 735 | ||
2.9.4.4. Pristine Highland Rocks: Distinctiveness of the Ferroan Anorthositic Suite | 736 | ||
2.9.4.5. The Magma Ocean Hypothesis | 740 | ||
2.9.4.6. Alternative Models | 742 | ||
2.9.5. Water in the Moon | 743 | ||
2.9.5.1. Traditional View of a Dry Moon | 743 | ||
2.9.5.2. Water in Pyroclastic Glasses | 744 | ||
2.9.5.3. Water in Apatite in Mare Basalts and KREEP-Related Samples | 744 | ||
2.9.5.4. Water in the Lunar Mantle | 745 | ||
2.9.5.5. Implications of Water in the Lunar Interior | 745 | ||
2.9.6. The Bulk Composition and Origin of the Moon | 746 | ||
Acknowledgments | 747 | ||
References | 747 | ||
Chapter 2.10: Mars | 756 | ||
2.10.1. Geochemical Exploration of Mars | 756 | ||
2.10.1.1. The Pace of Discovery | 756 | ||
2.10.1.2. Planetary Geology Overview | 757 | ||
2.10.2. Sources of Geochemical Data | 757 | ||
2.10.2.1. Geologic Context for Geochemical Data Sets | 757 | ||
2.10.2.2. Global Surface Chemistry from Orbiter Measurements | 759 | ||
2.10.2.3. In Situ Chemical Analyses of Rocks and Soils by Landers and Rovers | 760 | ||
2.10.2.4. Chemical Analyses of the Atmosphere | 761 | ||
2.10.2.5. Chemical Analyses of Martian Meteorites | 761 | ||
2.10.2.6. Geophysical Constraints on Chemistry of the Planet's Interior | 762 | ||
2.10.3. Geochemistry of Planetary Differentiation | 762 | ||
2.10.3.1. Bulk Planet Composition and Consistency with Geophysical Constraints | 762 | ||
2.10.3.2. Geochemical Models for the Mantle | 764 | ||
2.10.3.3. Geochemical Models for the Core | 765 | ||
2.10.3.4. Geochemistry of the Crust | 766 | ||
2.10.3.5. Radioisotope Ages of Planet Formation and Differentiation | 770 | ||
2.10.4. Geochemistry of Magmatic Processes | 771 | ||
2.10.4.1. Orbital Analyses of Volcanic Rock Compositions | 771 | ||
2.10.4.2. Gusev, the Best Studied Igneous Province on Mars | 772 | ||
2.10.4.3. Other Mars Igneous Rocks – Bounce Rock and Mars Pathfinder | 772 | ||
2.10.4.4. Geochemistry and Geochronology of Martian Meteorites | 773 | ||
2.10.4.5. Magma Petrogenesis | 778 | ||
2.10.5. Geochemistry of Sedimentary and Alteration Processes | 780 | ||
2.10.5.1. Some Sedimentological Context | 780 | ||
2.10.5.2. Low-Temperature Weathering and Alteration | 781 | ||
2.10.5.3. Geochemistry of Depositional Processes | 783 | ||
2.10.5.4. Chemical Diagenesis | 783 | ||
2.10.5.5. Hydrothermal Processes | 784 | ||
2.10.5.6. The Burns Formation | 785 | ||
2.10.5.7. Other Sediments and Sedimentary Rocks | 787 | ||
2.10.5.8. Aqueous Geochemistry of Martian Soils | 789 | ||
2.10.6. Organic Matter, Volatile Reservoirs, and Geochemical Cycles | 789 | ||
2.10.6.1. Organic Compounds | 789 | ||
2.10.6.2. Volatile Inventories and Reservoirs | 791 | ||
2.10.6.3. Outgassing and Atmospheric Loss | 792 | ||
2.10.6.4. Geochemical Cycles on Mars | 793 | ||
2.10.7. Geochemical Changes with Time and Comparison with Earth | 794 | ||
2.10.7.1. Magmatic Evolution | 794 | ||
2.10.7.2. Sedimentary Evolution | 794 | ||
2.10.7.3. Comparisons of Mars and Earth | 796 | ||
2.10.8. Major Unresolved Problems | 798 | ||
References | 798 | ||
Chapter 2.11: Giant Planets | 806 | ||
2.11.1. The Giant Planets in Relation to the Solar System | 806 | ||
2.11.1.1. Basic Physical and Orbital Parameters | 806 | ||
2.11.1.2. Discovery and Historical Investigation of the Giant Planets | 807 | ||
2.11.2. Essential Determinants of the Physical Properties of the Giant Planets | 808 | ||
2.11.2.1. How We Know the Giant Planets Contain Hydrogen and Helium | 808 | ||
2.11.2.2. The Equation of State of Hydrogen and Helium as a Determinant of the Structure | 809 | ||
2.11.2.3. The Thermal Infrared Emission of the Giant Planets and Implications for Evolution | 810 | ||
2.11.2.4. The Interior Structure of the Giant Planets | 812 | ||
2.11.2.5. Elemental and Isotopic Abundances | 812 | ||
2.11.2.6. Atmospheric Dynamics and Magnetic Fields | 813 | ||
2.11.3. Origin and Evolution of the Giant Planets | 814 | ||
2.11.3.1. Basic Model for the Formation of the Planets from a Disk of Gas and Dust | 814 | ||
2.11.3.2. Constraints from the Composition of the Giant Planets | 814 | ||
2.11.4. Extrasolar Giant Planets | 815 | ||
2.11.5. Major Unsolved Problems and Future Progress | 815 | ||
References | 816 | ||
Chapter 2.12: Major Satellites of the Giant Planets | 818 | ||
2.12.1. Introduction | 818 | ||
2.12.2. Cosmochemical Context | 819 | ||
2.12.3. Bulk Composition | 819 | ||
2.12.3.1. Bulk Density | 819 | ||
2.12.3.2. Sources of Data | 820 | ||
2.12.4. Surface Composition | 820 | ||
2.12.4.1. Spectral Reflectance | 820 | ||
2.12.4.2. Temperature and Atmospheres | 821 | ||
2.12.4.3. Radiation Effects | 821 | ||
2.12.5. The Jupiter System | 821 | ||
2.12.5.1. General | 821 | ||
2.12.5.2. Io | 822 | ||
2.12.5.2.1. Surface composition and volcanism | 822 | ||
2.12.5.2.2. Atmosphere and magnetospheric interactions | 823 | ||
2.12.5.3. Ganymede and Callisto | 824 | ||
2.12.5.3.1. Surface composition | 824 | ||
2.12.5.3.2. Atmospheres and magnetospheric interactions | 826 | ||
2.12.5.4. Europa | 826 | ||
2.12.5.4.1. Surface composition | 826 | ||
2.12.5.4.2. Atmosphere and magnetospheric interactions | 827 | ||
2.12.6. The Saturn System | 827 | ||
2.12.6.1. General | 827 | ||
2.12.6.2. Iapetus | 829 | ||
2.12.6.3. Titan | 829 | ||
2.12.6.4. Enceladus | 830 | ||
2.12.6.5. Magnetospheric Interactions | 831 | ||
2.12.7. The Uranus System | 831 | ||
2.12.7.1. General | 831 | ||
2.12.8. The Neptune System – Triton | 833 | ||
2.12.8.1. General | 833 | ||
2.12.8.2. Composition | 834 | ||
2.12.8.3. Atmosphere | 835 | ||
2.12.9. Major Issues and Future Directions | 835 | ||
References | 836 | ||
Chapter 2.13: Comets | 840 | ||
2.13.1. Introduction | 840 | ||
2.13.2. Comet and Asteroid Comparisons | 841 | ||
2.13.3. Comet Activity | 842 | ||
2.13.4. Comet Types – Orbital Distinction | 842 | ||
2.13.4.1. Major Comet Source Regions | 843 | ||
2.13.4.2. The Oort Cloud | 843 | ||
2.13.4.3. The Kuiper Belt | 844 | ||
2.13.4.3.1. KBO terminology | 844 | ||
2.13.4.3.2. KBO orbital distribution | 844 | ||
2.13.5. Physical Evolution of Comets | 845 | ||
2.13.5.1. Fragmentation | 845 | ||
2.13.5.2. Crust/Mantle Formation | 846 | ||
2.13.5.3. Strength and Structure of Cometary Materials | 849 | ||
2.13.6. Major Component Composition | 849 | ||
2.13.6.1. Water Ice | 849 | ||
2.13.6.2. CO and Very Volatile Compounds | 850 | ||
2.13.6.2.1. Full volatile composition | 851 | ||
2.13.6.3. Dust and Rocks | 852 | ||
2.13.6.3.1. Information from astronomical observations | 852 | ||
2.13.6.3.2. Information from collected samples | 853 | ||
2.13.6.3.2.1. Samples collected from active comets | 854 | ||
2.13.6.3.2.2. Meteoritic samples | 858 | ||
2.13.6.3.3. Information from in situ measurements at comet Halley | 860 | ||
2.13.7. Diversity Among Comets | 861 | ||
2.13.8. Conclusions | 863 | ||
References | 864 | ||
Chapter 2.14: Asteroids | 870 | ||
2.14.1. Introduction | 871 | ||
2.14.2. Background | 871 | ||
2.14.3. Remote Observations | 873 | ||
2.14.3.1. Reflectance Spectroscopy | 873 | ||
2.14.3.2. Mineral Spectroscopy | 874 | ||
2.14.3.3. Electronic Transitions | 874 | ||
2.14.3.4. Vibrational Bands | 875 | ||
2.14.3.5. Visual Albedo | 876 | ||
2.14.3.6. Metallic Iron | 877 | ||
2.14.3.7. Organics | 877 | ||
2.14.3.8. Space Weathering | 877 | ||
2.14.3.9. Determining Mineralogies | 878 | ||
2.14.4. Taxonomy | 879 | ||
2.14.4.1. A-Types | 881 | ||
2.14.4.2. C-Complex | 882 | ||
2.14.4.2.1. B-types | 882 | ||
2.14.4.2.2. C-types | 883 | ||
2.14.4.3. D-Types | 884 | ||
2.14.4.4. K-Types | 885 | ||
2.14.4.5. L-Types | 885 | ||
2.14.4.6. O-Types | 886 | ||
2.14.4.7. Q-Types | 886 | ||
2.14.4.8. R-Types | 886 | ||
2.14.4.9. S-Complex | 887 | ||
2.14.4.9.1. S-types | 887 | ||
2.14.4.9.2. Sa-types | 888 | ||
2.14.4.9.3. Sq-types | 888 | ||
2.14.4.9.4. Sr-types | 889 | ||
2.14.4.9.5. Sv-types | 889 | ||
2.14.4.10. T-Types | 889 | ||
2.14.4.11. V-Types | 889 | ||
2.14.4.12. X-Complex (E-Types, M-Types, and P-Types) | 891 | ||
2.14.4.12.1. E-types | 891 | ||
2.14.4.12.2. M-types | 892 | ||
2.14.4.12.3. P-types | 893 | ||
2.14.5. Spacecraft Missions | 893 | ||
2.14.5.1. Galileo | 893 | ||
2.14.5.2. Deep Space 1 | 894 | ||
2.14.5.3. NEAR Shoemaker | 894 | ||
2.14.5.4. Stardust | 896 | ||
2.14.5.5. Hayabusa | 896 | ||
2.14.5.6. Rosetta | 897 | ||
2.14.5.7. Dawn | 898 | ||
2.14.5.8. OSIRIS-REx | 898 | ||
2.14.5.9. 2008 TC3 and Almahata Sitta | 899 | ||
2.14.6. Interesting Groups of Asteroids | 899 | ||
2.14.6.1. Earth and Martian Trojans | 899 | ||
2.14.6.2. Near-Earth Asteroids | 899 | ||
2.14.6.3. Asteroid Families | 900 | ||
2.14.6.3.1. Inner belt (∼2.06-2.50AU) | 900 | ||
2.14.6.3.2. Middle belt (∼2.50-2.82AU) | 902 | ||
2.14.6.3.3. Outer belt (~2.82-3.28AU) | 903 | ||
2.14.6.3.4. Trojan region (~5.2AU) | 904 | ||
2.14.7. Taxonomic Distribution of Taxonomic Types | 904 | ||
2.14.7.1. Earlier Work | 904 | ||
2.14.7.2. Recent Work | 905 | ||
2.14.7.3. Formation of Material in the Solar Nebula | 905 | ||
2.14.7.4. Origin of the Taxonomic Distribution | 906 | ||
2.14.8. Conclusions and Future Work | 907 | ||
Acknowledgments | 908 | ||
References | 909 | ||
e9780080983004v3 | 921 | ||
e9780080983004v4 | 1525 | ||
e9780080983004v5 | 2358 | ||
Cover | 2358 | ||
The Atmosphere | 2361 | ||
Copyright | 2362 | ||
In Memoriam | 2363 | ||
Heinrich Dieter Holland (1927–2012) | 2365 | ||
Karl Karekin Turekian (1927–2013) | 2367 | ||
References | 2369 | ||
Dedication | 2371 | ||
Contents | 2373 | ||
Executive Editors’ Foreword to the Second Edition | 2375 | ||
Contributors | 2379 | ||
Volume Editor’s Introduction | 2381 | ||
Chapter 5.1: Ozone, Hydroxyl Radical, and Oxidative Capacity | 2385 | ||
5.1.1. Introduction | 2385 | ||
5.1.2. Evolution of Oxidizing Capability | 2387 | ||
5.1.2.1. Prebiotic Atmosphere | 2387 | ||
5.1.2.2. Preindustrial Atmosphere | 2387 | ||
5.1.3. Fundamental Reactions | 2388 | ||
5.1.3.1. Troposphere | 2388 | ||
5.1.3.2. Stratosphere | 2390 | ||
5.1.4. Meteorological Influences | 2391 | ||
5.1.5. Human Influences | 2391 | ||
5.1.5.1. Industrial Revolution | 2391 | ||
5.1.5.2. Future Projections | 2392 | ||
5.1.6. Measuring Oxidation Rates | 2393 | ||
5.1.6.1. Direct Measurement | 2393 | ||
5.1.6.2. Indirect Measurement | 2395 | ||
5.1.7. Atmospheric Models and Observations | 2399 | ||
5.1.8. Conclusions | 2400 | ||
References | 2400 | ||
Chapter 5.2: Tropospheric Halogen Chemistry | 2403 | ||
5.2.1. Introduction | 2403 | ||
5.2.2. Main Reaction Mechanisms | 2405 | ||
5.2.3. Tropospheric Ozone Depletion at Polar Sunrise | 2410 | ||
5.2.3.1. Main Features of Polar ODEs | 2411 | ||
5.2.3.2. Satellite Observations | 2413 | ||
5.2.3.3. Sources of Active Bromine | 2414 | ||
5.2.3.4. Chlorine Chemistry in ODEs and Br-Cl Interactions | 2416 | ||
5.2.3.5. The Role of Iodine in ODEs | 2417 | ||
5.2.3.6. Halogen-Mercury Interactions | 2418 | ||
5.2.3.7. Model Studies | 2418 | ||
5.2.4. Marine Boundary Layer | 2419 | ||
5.2.4.1. Sea Salt Aerosol | 2419 | ||
5.2.4.2. Reactive Chlorine | 2422 | ||
5.2.4.3. Reactive Bromine | 2423 | ||
5.2.4.3.1. Field observations | 2423 | ||
5.2.4.3.2. Model results | 2425 | ||
5.2.4.4. Reactive Iodine | 2427 | ||
5.2.4.5. Surface-Segregation Effects | 2432 | ||
5.2.4.6. Halogen-Sulfur Interactions | 2433 | ||
5.2.5. Salt Lakes | 2435 | ||
5.2.6. Volcanoes | 2436 | ||
5.2.7. Free Troposphere | 2438 | ||
5.2.8. Additional Sources of Reactive Halogens | 2441 | ||
5.2.8.1. Industry and Fossil Fuel Burning | 2441 | ||
5.2.8.2. Biomass Burning and Dust Plumes | 2441 | ||
5.2.8.3. Organic Halogen Compounds | 2442 | ||
5.2.8.4. Inventories | 2443 | ||
5.2.9. Summary | 2443 | ||
Acknowledgments | 2444 | ||
References | 2444 | ||
Chapter 5.3: Global Methane Biogeochemistry | 2455 | ||
5.3.1. Introduction | 2455 | ||
5.3.2. Global Methane Budget | 2456 | ||
5.3.2.1. Global Methane Increase | 2456 | ||
5.3.2.2. Methane Budget with Constraints | 2458 | ||
5.3.2.3. Gross Methane Budget | 2459 | ||
5.3.2.4. Atmospheric Models | 2459 | ||
5.3.2.5. Stable Isotopes | 2460 | ||
5.3.3. Terrestrial Studies | 2462 | ||
5.3.3.1. Flux Time Series | 2462 | ||
5.3.3.2. Flux Transects | 2462 | ||
5.3.3.3. Process-Level Studies | 2462 | ||
5.3.3.3.1. Vegetation removal experiments | 2462 | ||
5.3.3.3.2. Methane flux: net ecosystem exchange relationship | 2463 | ||
5.3.3.4. Scaling Up | 2463 | ||
5.3.3.5. Wetland Soil Models | 2463 | ||
5.3.3.6. Animals and Landfills | 2464 | ||
5.3.3.7. Microbial Soil Oxidation | 2464 | ||
5.3.3.7.1. Moist soils | 2464 | ||
5.3.3.7.2. Waterlogged soils | 2466 | ||
5.3.3.7.3. Nitrogen fertilization and disturbance | 2466 | ||
5.3.3.7.4. Effects of drying on paddy soils | 2466 | ||
5.3.3.8. New Techniques | 2466 | ||
5.3.4. Marine Studies | 2467 | ||
5.3.4.1. Ocean Methane Source | 2467 | ||
5.3.4.2. Aerobic Methane Oxidation | 2467 | ||
5.3.4.3. Anaerobic Methane Oxidation | 2468 | ||
5.3.4.4. Methane Clathrate Hydrates | 2469 | ||
5.3.4.4.1. Methane hydrate reservoir | 2469 | ||
5.3.4.4.2. Methane hydrate decomposition rates | 2469 | ||
5.3.4.4.3. How can we estimate the rate of CH4 clathrate decomposition? | 2469 | ||
5.3.4.4.4. Natural 14C-CH4 measurements | 2470 | ||
5.3.4.5. Other Ocean Methane Sources | 2470 | ||
5.3.5. Ice Cores | 2472 | ||
5.3.6. Future Work | 2473 | ||
Acknowledgments | 2473 | ||
References | 2474 | ||
Chapter 5.4: Tropospheric Aerosols | 2479 | ||
5.4.1. Introduction | 2480 | ||
5.4.1.1. Overview | 2480 | ||
5.4.1.2. Sources | 2482 | ||
5.4.1.3. Atmospheric Residence, Transport, and Removal | 2483 | ||
5.4.1.4. Primary versus Secondary Particles and Particle Nucleation | 2483 | ||
5.4.1.5. Spatial Homogeneity | 2483 | ||
5.4.2. Aerosol Properties | 2485 | ||
5.4.2.1. Number and Mass Distributions | 2485 | ||
5.4.2.2. Composition | 2489 | ||
5.4.2.3. Optical Properties | 2489 | ||
5.4.3. Measurement of Aerosol Properties | 2490 | ||
5.4.3.1. Particle Sizes, Size Distributions, and Number and Mass Concentrations | 2490 | ||
5.4.3.2. Composition | 2493 | ||
5.4.3.2.1. Bulk composition | 2493 | ||
5.4.3.2.2. Off-line measurements of single-particle compositions | 2495 | ||
5.4.3.2.3. On-line measurements | 2497 | ||
5.4.3.3. Hygroscopicity | 2498 | ||
5.4.3.4. Optical Properties | 2501 | ||
5.4.4. Spatial and Temporal Variation of Tropospheric Aerosols | 2501 | ||
5.4.4.1. Remote Sensing of Aerosol Using Passive Light Sources | 2501 | ||
5.4.4.2. Remote Sensing of Aerosol Using an Active Light Source | 2503 | ||
5.4.5. Aerosol Processes | 2506 | ||
5.4.5.1. Emissions | 2506 | ||
5.4.5.2. Gas-to-Particle Conversions and other Atmospheric Reactions | 2507 | ||
5.4.5.3. Long-Range Transport | 2509 | ||
5.4.5.4. Removal of Particles from the Atmosphere | 2509 | ||
5.4.6. Representation of Aerosol Processes in Chemical Transport and Transformation Models | 2509 | ||
5.4.7. Aerosol Influences on Climate and Climate Change | 2512 | ||
5.4.7.1. Background | 2512 | ||
5.4.7.2. Direct Aerosol Shortwave Radiative Forcing | 2513 | ||
5.4.7.3. Clouds and Indirect Effects | 2515 | ||
5.4.7.4. Aerosol Forcing Relative to other Forcings of Climate Change over the Industrial Period | 2516 | ||
5.4.8. Final Thoughts | 2517 | ||
Acknowledgments | 2517 | ||
References | 2518 | ||
Chapter 5.5: Biomass Burning: The Cycling of Gases and Particulates from the Biosphere to the Atmosphere | 2523 | ||
5.5.1. Introduction: Biomass Burning, Geochemical Cycling, and Global Change | 2523 | ||
5.5.2. Global Impacts of Biomass Burning | 2524 | ||
5.5.3. Enhanced Biogenic Soil Emissions of Nitrogen and Carbon Gases: A Postfire Effect | 2525 | ||
5.5.4. The Geographical Distribution of Biomass Burning | 2525 | ||
5.5.5. Biomass Burning in the Boreal Forests | 2526 | ||
5.5.6. Estimates of Global Burning and Global Gaseous and Particulate Emissions | 2528 | ||
5.5.7. Calculation of Gaseous and Particulate Emissions from Fires | 2528 | ||
5.5.8. Biomass Burning and Atmospheric Nitrogen and Oxygen | 2529 | ||
5.5.9. Atmospheric Chemistry Resulting from Gaseous Emissions from the Fires | 2529 | ||
5.5.9.1. Chemistry of the Hydroxyl Radical (OH) in the Troposphere | 2529 | ||
5.5.9.2. Production of O3 in the Troposphere | 2530 | ||
5.5.9.2.1. CO oxidation chain | 2530 | ||
5.5.9.2.2. Methane oxidation chain | 2530 | ||
5.5.9.3. Chemistry of Nitrogen Oxides in the Troposphere | 2530 | ||
5.5.9.4. Chemistry of the Stratosphere | 2530 | ||
5.5.10. A Case Study of Biomass Burning: The 1997 Wildfires in Southeast Asia | 2530 | ||
5.5.11. Results of Calculations: Gaseous and Particulate Emissions from the Fires in Kalimantan and Sumatra, Indonesia, Augus | 2531 | ||
5.5.12. The Impact of the Southeastern Asia Fires on the Composition and Chemistry of the Atmosphere | 2532 | ||
5.5.12.1. Modeling O3 and CO over Indonesia | 2532 | ||
5.5.12.2. Measurements over Indonesia | 2533 | ||
5.5.12.3. Measurements between Singapore and Japan | 2533 | ||
5.5.12.4. Measurements over Hawaii | 2533 | ||
References | 2533 | ||
Chapter 5.6: Mass-Independent Isotopic Composition of Terrestrial and Extraterrestrial Materials | 2535 | ||
5.6.1. General Introduction | 2535 | ||
5.6.2. Applications of Mass-Independent Isotopic Effects | 2536 | ||
5.6.3. Isotopic Anomalies in Extraterrestrial Atmospheres a | 2541 | ||
5.6.3.1. Physical Chemistry of Mass-Independent Isotope Effects | 2542 | ||
5.6.4. Atmospheric Observations of Mass-Independent Isotopic Compositions | 2544 | ||
5.6.4.1. Stratospheric and Tropospheric Ozone | 2544 | ||
5.6.4.2. Stratospheric Carbon Dioxide | 2545 | ||
5.6.5. Atmospheric Aerosol Sulfate: Present Earth's Atmosphere | 2546 | ||
5.6.6. Mass-Independent Oxygen Isotopic Composition of Paleosulfates | 2547 | ||
5.6.7. Atmospheric Mass-Independent Molecular Oxygen | 2549 | ||
5.6.8. The Atmospheric Aerosol Nitrate and the Nitrogen Cycle | 2549 | ||
5.6.9. Mass-Independent Oxygen Isotopic Compositions in Solids to Reflect Atmospheric Change: Earth and Mars | 2550 | ||
5.6.10. Sulfur in the Earth's Earliest Atmosphere: The Rise of Oxygen | 2553 | ||
5.6.11. Sulfur Isotopic Fractionation Processes in other Solar System Objects | 2555 | ||
5.6.12. Concluding Comments | 2555 | ||
Acknowledgments | 2556 | ||
References | 2556 | ||
Chapter 5.7: The Stable Isotopic Composition of Atmospheric CO2 | 2563 | ||
5.7.1. Introduction | 2563 | ||
5.7.2. Methodology and Terminology | 2564 | ||
5.7.3. delta13C in Atmospheric CO2 | 2566 | ||
5.7.3.1. Fossil Fuel Input | 2569 | ||
5.7.3.2. Exchange with the Ocean | 2570 | ||
5.7.3.3. Ocean Biology | 2570 | ||
5.7.3.4. Atmosphere-Ocean Disequilibrium | 2571 | ||
5.7.3.5. Photosynthetic CO2 Uptake on Land | 2572 | ||
5.7.3.6. CO2 Release in Respiration | 2575 | ||
5.7.3.7. The Land Disequilibrium | 2576 | ||
5.7.3.8. Ecosystem Discrimination | 2576 | ||
5.7.3.9. Incorporating Isotopes in Flux Measurements | 2579 | ||
5.7.4. delta18O in CO2 | 2579 | ||
5.7.4.1. The Soil Component | 2581 | ||
5.7.4.2. The Leaf Component | 2582 | ||
5.7.4.3. The Minor Components | 2584 | ||
5.7.4.3.1. The ocean component | 2584 | ||
5.7.4.3.2. Anthropogenic emissions | 2584 | ||
5.7.4.3.3. Troposphere-stratosphere exchange | 2585 | ||
5.7.4.4. Spatial and Temporal Patterns | 2585 | ||
5.7.5. Clumped Isotopes | 2587 | ||
5.7.5.1. Clumped Isotopes in Atmospheric CO2 | 2588 | ||
5.7.5.1.1. Delta47 in combustion and background air | 2588 | ||
5.7.5.1.2. Seasonal variations in Delta47 | 2589 | ||
5.7.5.1.3. Delta47 in stratospheric CO2 | 2589 | ||
5.7.6. Concluding Remarks | 2589 | ||
Acknowledgments | 2590 | ||
References | 2590 | ||
Chapter 5.8: Water Stable Isotopes: Atmospheric Composition and Applications in Polar Ice Core Studies | 2597 | ||
5.8.1. Introduction | 2598 | ||
5.8.2. Present-Day Observations | 2601 | ||
5.8.2.1. Deuterium and Oxygen-18 in Precipitation | 2601 | ||
5.8.2.2. Oxygen-17 and 17O-Excess | 2602 | ||
5.8.2.3. Water Isotopes in the Atmosphere | 2603 | ||
5.8.3. Physics of Water Isotopes | 2605 | ||
5.8.3.1. Fractionation Processes | 2605 | ||
5.8.3.2. Growth of Individual Elements | 2606 | ||
5.8.3.3. Isotopic Processes in Clouds | 2607 | ||
5.8.4. Modeling the Water Isotope Atmospheric Cycle | 2609 | ||
5.8.4.1. Rayleigh-Type Models | 2609 | ||
5.8.4.2. Isotopic Models of Higher Complexity | 2610 | ||
5.8.4.3. Isotope Modeling with GCMs | 2610 | ||
5.8.4.3.1. Early IGCMs | 2611 | ||
5.8.4.3.2. Recent simulations | 2612 | ||
5.8.4.3.3. Nudged and regional isotopic models | 2615 | ||
5.8.5. Ice Core Isotopic Records | 2617 | ||
5.8.6. The Conventional Approach for Interpreting Water Isotopes in Ice Cores | 2621 | ||
5.8.7. Alternative Estimates of Temperature Changes in Greenland and Antarctica | 2622 | ||
5.8.7.1. Greenland | 2623 | ||
5.8.7.2. Antarctica | 2624 | ||
5.8.8. What Do People Learn from GCMs? | 2625 | ||
5.8.8.1. Influence of the Seasonality of Precipitation | 2625 | ||
5.8.8.2. Estimating the Temporal Slope from IGCMs | 2626 | ||
5.8.9. Influence of the Oceanic Source of Polar Precipitation | 2629 | ||
5.8.10. Conclusion | 2632 | ||
Acknowledgments | 2633 | ||
References | 2633 | ||
Chapter 5.9: Radiocarbon | 2641 | ||
5.9.1. Introduction | 2641 | ||
5.9.2. Production and Distribution of 14C | 2641 | ||
5.9.3. Measurements of Radiocarbon | 2641 | ||
5.9.4. Timescale Calibration | 2642 | ||
5.9.4.1. Calibration Based on Tree Rings | 2642 | ||
5.9.4.2. Calibration Based on Corals | 2645 | ||
5.9.4.3. Other Calibration Schemes | 2645 | ||
5.9.4.4. Cause of the Long-Term 14C Decline | 2645 | ||
5.9.4.5. Change in Ocean Operation | 2646 | ||
5.9.5. Radiocarbon and Solar Irradiance | 2647 | ||
5.9.6. The `Bomb´ 14C Transient | 2649 | ||
5.9.6.1. Radiocarbon as a Tracer for Ocean Uptake of Fossil Fuel CO2 | 2650 | ||
5.9.6.2. Ocean Uptake of 14CO2 and CO2 | 2650 | ||
5.9.6.3. Terrestrial Uptake of 14CO2 and CO2 | 2652 | ||
5.9.7. Future Applications | 2653 | ||
References | 2653 | ||
Chapter 5.10: Natural Radionuclides in the Atmosphere | 2657 | ||
5.10.1. Introduction | 2657 | ||
5.10.2. Radon and Its Daughters | 2657 | ||
5.10.2.1. Flux of Radon from Soils to the Atmosphere | 2657 | ||
5.10.2.2. Flux of Radon from the Oceans | 2660 | ||
5.10.2.3. Distribution of Radon in the Atmosphere | 2660 | ||
5.10.2.4. Short-Lived Daughters of 222Rn in the Atmosphere | 2661 | ||
5.10.2.5. 210Pb and Its Progeny | 2661 | ||
5.10.2.5.1. Distribution of 210Pb in the atmosphere | 2661 | ||
5.10.2.5.2. Flux of 210Pb to the Earth's surface | 2662 | ||
5.10.2.5.3. Residence time of 210Pb and associated species in the atmosphere | 2663 | ||
5.10.2.5.4. Use of 210Pb as surrogate for other atmospheric components | 2665 | ||
5.10.3. Cosmogenic Nuclides | 2667 | ||
5.10.3.1. Atmospheric Production of Cosmogenic Nuclides | 2667 | ||
5.10.3.2. 7Be and 10Be | 2668 | ||
5.10.3.3. 35S and the Kinetics of SO2 Oxidation and Deposition | 2668 | ||
5.10.3.4. Phosphorus Isotopes | 2670 | ||
5.10.4. Coupled Lead-210 and Beryllium-7 | 2670 | ||
5.10.4.1. Temporal and Spatial Variation | 2670 | ||
5.10.4.2. Application of the Coupled 7Be-210Pb System to Sources of Atmospheric Species | 2671 | ||
References | 2672 | ||
Chapter 5.11: Carbonaceous Particles: Source-Based Characterization of Their Formation, Composition, and Structures | 2675 | ||
5.11.1. Introduction | 2676 | ||
5.11.1.1. Classifying Types of Carbonaceous Particles by Source | 2676 | ||
5.11.1.2. Four Categories of Carbonaceous Particles | 2678 | ||
5.11.2. Carbonaceous Particles from Fossil Fuel Combustion | 2681 | ||
5.11.2.1. FFC Carbonaceous Particle Formation | 2682 | ||
5.11.2.2. FFC Carbonaceous Particle Chemical Composition | 2683 | ||
5.11.2.3. FFC Carbonaceous Particle Structure | 2684 | ||
5.11.3. Biofuel and Biomass Burning Carbonaceous Particles | 2684 | ||
5.11.3.1. BBB Carbonaceous Particle Formation | 2684 | ||
5.11.3.2. BBB Carbonaceous Particle Chemical Composition | 2685 | ||
5.11.3.3. BBB Carbonaceous Particle Structure | 2686 | ||
5.11.4. Carbonaceous Particles from Biogenic Vapor Fluxes | 2687 | ||
5.11.4.1. BVF Carbonaceous Particle Formation | 2687 | ||
5.11.4.2. BVF Carbonaceous Particle Chemical Composition | 2687 | ||
5.11.4.3. BVF Carbonaceous Particle Structure | 2689 | ||
5.11.5. Carbonaceous Particles from Mechanically Lofted Biological Componen | 2689 | ||
5.11.5.1. MBC Carbonaceous Particle Formation | 2689 | ||
5.11.5.2. MBC Carbonaceous Particle Chemical Composition | 2690 | ||
5.11.5.3. MBC Carbonaceous Particle Structures | 2691 | ||
5.11.6. Impacts of Carbonaceous Particle on the Earth System | 2691 | ||
5.11.6.1. Physical Properties of Carbonaceous Particles | 2691 | ||
5.11.6.2. Global and Regional Modeling of Current and Future Carbonaceous Particles | 2692 | ||
Appendix A. Measurement Techniques for Carbonaceous Particles | 2692 | ||
Acknowledgments | 2694 | ||
References | 2695 | ||
Chapter 5.12: Ocean-Derived Aerosol and Its Climate Impacts | 2701 | ||
5.12.1. Introduction | 2701 | ||
5.12.2. Ocean-Derived Aerosol Production Mechanisms | 2701 | ||
5.12.3. Radiative Effects of Ocean-Derived Aerosol | 2703 | ||
5.12.3.1. Aerosol Direct Effects | 2703 | ||
5.12.3.2. Aerosol-Cloud Interactions | 2703 | ||
5.12.4. Sources and Composition of Ocean-Derived CCN | 2704 | ||
5.12.4.1. The Dimethylsulfide Source of Ocean-Derived CCN | 2704 | ||
5.12.4.1.1. Production of DMS-derived CCN | 2705 | ||
5.12.4.2. The Sea Spray Source of Ocean-Derived CCN | 2706 | ||
5.12.4.2.1. Sea salt aerosol | 2706 | ||
5.12.4.2.2. Organic aerosol | 2706 | ||
5.12.5. The MBL CCN Budget | 2709 | ||
5.12.5.1. Production Fluxes of Sea Spray Aerosol | 2709 | ||
5.12.6. The CLAW Hypothesis | 2711 | ||
5.12.7. Concluding Comments | 2712 | ||
Acknowledgments | 2712 | ||
References | 2712 | ||
Chapter 5.13: Aerosol Hygroscopicity: Particle Water Content and Its Role in Atmospheric Processes | 2715 | ||
5.13.1. Introduction | 2716 | ||
5.13.2. Methods for the Measurement of Aerosol Water Contents | 2717 | ||
5.13.2.1. Instruments Operating Below Water Saturation | 2717 | ||
5.13.2.1.1. Approaches using microscopy | 2718 | ||
5.13.2.1.2. Electrodynamic balance | 2719 | ||
5.13.2.1.3. Humidified tandem differential mobility analyzers | 2719 | ||
5.13.2.1.4. Optical humidified/dry measurements (f(RH)) | 2720 | ||
5.13.2.2. Instruments Operating Above Water Saturation | 2722 | ||
5.13.2.3. Water Uptake by Adsorption | 2723 | ||
5.13.3. Parameterizations of Aerosol Hygroscopicity | 2724 | ||
5.13.3.1. Diameter Growth Factor (g(RH)) Fits | 2724 | ||
5.13.3.2. The Hygroscopicity Parameter, kappa | 2725 | ||
5.13.3.3. Fits for f(RH) | 2726 | ||
5.13.3.4. Fits for Adsorbed Water Contents | 2726 | ||
5.13.4. Laboratory Measurements for Selected Aerosol Types | 2727 | ||
5.13.4.1. Measurements of Aerosol Water Contents | 2727 | ||
5.13.4.1.1. Inorganic ionic species | 2727 | ||
5.13.4.1.2. Organic species | 2728 | ||
5.13.4.1.3. Multicomponent particles | 2729 | ||
5.13.4.1.4. Dust | 2730 | ||
5.13.4.1.5. Soot particles | 2730 | ||
5.13.4.1.6. Biological particles | 2733 | ||
5.13.4.2. Optical Measurements | 2733 | ||
5.13.5. Observations of Aerosol Water Content and Atmospheric Implications | 2734 | ||
5.13.5.1. g(RH) Observations | 2734 | ||
5.13.5.2. CCN-Derived kappa Observations | 2736 | ||
5.13.5.3. f(RH) Observations | 2736 | ||
5.13.5.4. Atmospheric Implications | 2738 | ||
Acknowledgments | 2739 | ||
References | 2739 | ||
Chapter 5.14: The Stable Isotopic Composition of Atmospheric O2 | 2747 | ||
5.14.1. Introduction | 2747 | ||
5.14.2. Methodology and Terminology | 2748 | ||
5.14.2.1. Abundance and Fractionation of Oxygen Isotopes | 2748 | ||
5.14.2.1.1. Isotopic fractionation | 2748 | ||
5.14.2.2. Relationships between Fractionations of 17O/16O and 18O/16O | 2749 | ||
5.14.3. 18O/16O Ratios in Atmospheric O2 | 2750 | ||
5.14.3.1. The Dole Effect and Its Magnitude | 2750 | ||
5.14.3.2. Processes Influencing the Dole Effect | 2750 | ||
5.14.3.2.1. Biological O2 consumption | 2750 | ||
5.14.3.2.2. Photosynthetic O2 production | 2753 | ||
5.14.3.2.3. Hydrologic processes | 2755 | ||
5.14.3.2.4. Stratospheric photochemical reactions | 2756 | ||
5.14.3.3. Global Budgets of Processes Influencing the Dole Effect | 2757 | ||
5.14.3.4. Temporal Variations in the Dole Effect | 2758 | ||
5.14.4. Oxygen-17 and Oxygen-18 in Atmospheric O2 | 2760 | ||
5.14.4.1. Mass-Independent Fractionation and Biological Normalization | 2760 | ||
5.14.4.2. Temporal Variations in 17O/16O and 18O/16O | 2762 | ||
References | 2764 | ||
Chapter 5.15: Studies of Recent Changes in Atmo | 2769 | ||
5.15.1. Introduction | 2769 | ||
5.15.2. Overview of the Large-Scale Varia | 2770 | ||
5.15.2.1. Long-Term Trends | 2770 | ||
5.15.2.2. Seasonal Cycles | 2772 | ||
5.15.2.3. Atmospheric Potential Oxygen | 2773 | ||
5.15.3. Measurement Methods | 2773 | ||
5.15.4. O2-Based Global Carbon Budgets | 2774 | ||
5.15.4.1. Budget Equations | 2774 | ||
5.15.4.2. Updated Budget for Recent Deca | 2775 | ||
5.15.4.3. Updated Budgets Discussion | 2776 | ||
5.15.4.4. Oxidative Ratios of the Long-T | 2777 | ||
5.15.4.5. Nitrogen Cycle Perturbations | 2778 | ||
5.15.4.6. Ocean O2 and N2 Outgassing | 2779 | ||
5.15.5. Seasonal Cycles in APO | 2780 | ||
5.15.6. Interannual Variability in APO | 2781 | ||
5.15.7. Interhemispheric Gradient in O2/N2 and APO | 2782 | ||
5.15.8. Diurnal and Other Shorter-Term | 2784 | ||
5.15.9. Future Outlook | 2785 | ||
Acknowledgments | 2786 | ||
References | 2786 | ||
Chapter 5.16: Fluorine-Containing Greenhouse Gases | 2789 | ||
5.16.1. Introduction | 2789 | ||
5.16.2. Global Observations | 2789 | ||
5.16.2.1. Surface-Based Observational Networks | 2789 | ||
5.16.2.2. Instruments and Calibration | 2791 | ||
5.16.2.3. Observed Mole Fractions and Trends | 2792 | ||
5.16.2.3.1. Trends in Montreal Protocol gases and their replacements | 2792 | ||
5.16.2.3.2. Trends in Kyoto Protocol gases | 2792 | ||
5.16.3. Global Cycles | 2793 | ||
5.16.3.1. Anthropogenic Sources, Atmospheric Circulation and Atmospheric Chemistry | 2793 | ||
5.16.3.2. Flux Estimation Techniques Using Models and Observations | 2795 | ||
5.16.3.3. Source, Sink, and Lifetime Estimates | 2796 | ||
5.16.4. Environmental Impacts, Current Trends and Emission Policies | 2797 | ||
5.16.4.1. Climate Forcing and the Montreal and Kyoto Protocols | 2797 | ||
5.16.4.2. Ozone Destruction and Montreal Protocol | 2798 | ||
5.16.5. Verification of Future National Emission Reports Using Observations | 2798 | ||
5.16.6. Conclusions | 2799 | ||
Acknowledgments | 2799 | ||
References | 2799 | ||
e9780080983004v6 | 2803 | ||
e9780080983004v7 | 3238 | ||
Front Cover | 3238 | ||
Surface and Ground Water, Weathering and Soils | 3241 | ||
Copyright | 3242 | ||
In Memoriam | 3243 | ||
Heinrich Dieter Holland (1927–2012) | 3245 | ||
Karl Karekin Turekian (1927–2013) | 3247 | ||
References | 3249 | ||
Dedication | 3251 | ||
Contents | 3253 | ||
Executive Editors’ Foreword to the Second Edition | 3255 | ||
Contributors | 3259 | ||
Volume Editor’s Introduction | 3261 | ||
Chapter 7.1: Soil Formation | 3263 | ||
7.1.1. Introduction | 3263 | ||
7.1.2. What Is Soil? | 3263 | ||
7.1.3. Geographical Access to Soil Data | 3264 | ||
7.1.4. Conceptual Partitioning of the Earth Surface | 3265 | ||
7.1.4.1. Stable Landforms | 3265 | ||
7.1.4.2. Erosional Landscapes | 3271 | ||
7.1.5. The Human Dimension of Soil Formation | 3274 | ||
7.1.5.1. Accelerated Soil Formation Under Agriculture | 3277 | ||
7.1.6. Soil Geochemistry in Deserts | 3280 | ||
7.1.7. Soil Formation on Mars | 3283 | ||
7.1.8. Concluding Remarks | 3287 | ||
References | 3287 | ||
Chapter 7.2: Modeling Low-Temperature Geochemical Processes | 3289 | ||
7.2.1. Introduction | 3289 | ||
7.2.1.1. What Is a Model? | 3290 | ||
7.2.2. Modeling Concepts and Definitions | 3291 | ||
7.2.2.1. Modeling Concepts | 3291 | ||
7.2.2.2. Modeling Definitions | 3291 | ||
7.2.2.3. Inverse Modeling, Mass Balancing, and Mole Balancing | 3291 | ||
7.2.3. Solving the Chemical Equilibrium Problem | 3293 | ||
7.2.4. Historical Background to Geochemical Modeling | 3294 | ||
7.2.5. The Problem of Activity Coefficients | 3295 | ||
7.2.5.1. Activity Coefficients | 3295 | ||
7.2.5.2. Saturation Indices | 3296 | ||
7.2.6. Geochemical Databases | 3296 | ||
7.2.6.1. Thermodynamic Databases | 3297 | ||
7.2.6.2. Kinetic Databases | 3298 | ||
7.2.6.3. Electrolyte Databases | 3298 | ||
7.2.7. Geochemical Codes | 3298 | ||
7.2.7.1. USGS Codes | 3298 | ||
7.2.7.2. LLNL/LBNL Codes | 3299 | ||
7.2.7.3. Miami Code | 3299 | ||
7.2.7.4. The Geochemist's Workbench™ | 3300 | ||
7.2.7.5. REDEQL–MINTEQ Codes | 3300 | ||
7.2.7.6. Waterloo Codes | 3301 | ||
7.2.7.7. Harvie–Møller–Weare Code | 3301 | ||
7.2.7.8. FREZCHEM Code | 3301 | ||
7.2.7.9. Windermere Humic Aqueous Model Codes | 3301 | ||
7.2.7.10. Additional Codes | 3302 | ||
7.2.8. Water–Rock Interactions | 3303 | ||
7.2.8.1. Aqueous Speciation | 3303 | ||
7.2.8.2. Sorption Reactions | 3304 | ||
7.2.8.3. Aqueous Redox Kinetics and Microbial Growth | 3304 | ||
7.2.8.4. Model Simulations of Mineral Reactions | 3306 | ||
7.2.8.4.1. Which minerals reach solubility equilibrium? | 3306 | ||
7.2.8.4.2. Calcite solubility | 3308 | ||
7.2.8.4.3. Pyrite oxidation | 3308 | ||
7.2.8.4.4. Pyrite oxidation with calcite dissolution and neutralization | 3310 | ||
7.2.8.4.5. Seawater–groundwater mixing | 3312 | ||
7.2.8.4.6. Madison regional limestone aquifer | 3312 | ||
7.2.8.5. Reactive-Transport Modeling in Streams | 3315 | ||
7.2.8.6. Reactive-Transport Modeling in Groundwater | 3317 | ||
7.2.8.7. Geochemical Modeling of Catchments | 3317 | ||
7.2.8.8. Evaporation of Seawater | 3318 | ||
7.2.8.9. Reliability of Geochemical Model Simulations | 3318 | ||
7.2.9. Final Comments | 3320 | ||
Acknowledgments | 3322 | ||
References | 3322 | ||
Chapter 7.3: Reaction Kinetics of Primary Rock-Forming Minerals under Ambient Conditions | 3331 | ||
7.3.1. Introduction | 3331 | ||
7.3.2. Experimental Techniques for Dissolution Measurements | 3334 | ||
7.3.2.1. Chemical Reactors | 3334 | ||
7.3.2.2. Interpretation and Extrapolation of Rate Data | 3335 | ||
7.3.3. Mechanisms of Dissolution | 3338 | ||
7.3.3.1. Models for Dissolution Based on Surface Topography | 3338 | ||
7.3.3.2. Carbonate Dissolution Mechanism | 3338 | ||
7.3.3.3. Silicate and Oxide Dissolution Mechanisms | 3339 | ||
7.3.3.3.1. Nonstoichiometric dissolution | 3339 | ||
7.3.3.3.2. Surface complexation models | 3341 | ||
7.3.3.3.3. Oelkers–Schott model | 3344 | ||
7.3.3.3.4. Mechanism of dissolution of redox-sensitive silicates | 3345 | ||
7.3.4. Surface Area | 3345 | ||
7.3.4.1. BET and Geometric Surface Area | 3345 | ||
7.3.4.2. Reactive Surface Area | 3347 | ||
7.3.5. Rate Constants as a Function of Mineral Composition | 3348 | ||
7.3.5.1. Silica | 3349 | ||
7.3.5.2. Feldspars | 3349 | ||
7.3.5.3. Nonframework Silicates | 3349 | ||
7.3.5.4. Carbonates | 3351 | ||
7.3.6. Temperature Dependence | 3352 | ||
7.3.6.1. Activation Energy | 3352 | ||
7.3.6.2. Temperature and the Effect of Solution Chemistry | 3354 | ||
7.3.7. Chemistry of Dissolving Solutions | 3354 | ||
7.3.7.1. Inorganic Species | 3354 | ||
7.3.7.2. Dissolved Carbon Dioxide | 3357 | ||
7.3.7.3. Ligand Effects | 3358 | ||
7.3.7.3.1. Models of ligand-promoted dissolution | 3358 | ||
7.3.7.3.2. Ligand-promoted dissolution of individual minerals | 3359 | ||
7.3.7.3.3. Complex ligands | 3360 | ||
7.3.8. Chemical Affinity | 3360 | ||
7.3.8.1. Rate Laws Linear in ∆G near Equilibrium | 3360 | ||
7.3.8.2. Rate Laws Nonlinear in ∆G near Equilibrium | 3361 | ||
7.3.8.3. Models Based on Surface Topography | 3363 | ||
7.3.9. Duration of Dissolution | 3365 | ||
7.3.10. Conclusion | 3366 | ||
Acknowledgments | 3367 | ||
References | 3367 | ||
Chapter 7.4: Natural Weathering Rates of Silicate Minerals | 3377 | ||
7.4.1. Introduction | 3378 | ||
7.4.2. Defining Natural Weathering Rates | 3379 | ||
7.4.3. Mass Changes Related to Chemical Weathering | 3380 | ||
7.4.3.1. Bulk Compositional Changes in Regoliths | 3381 | ||
7.4.3.1.1. Weathering indices | 3381 | ||
7.4.3.1.2. Mass transfer | 3383 | ||
7.4.3.1.3. Profile categories | 3385 | ||
7.4.3.1.4. Time evolution of profiles | 3386 | ||
7.4.3.1.5. Utilization of soil chronosequences | 3387 | ||
7.4.3.1.6. Steady-state denudation and weathering profile development | 3389 | ||
7.4.3.2. Changes Based on Solute Compositions | 3390 | ||
7.4.3.2.1. Characterization of fluid transport | 3390 | ||
7.4.3.2.2. Weathering based on solutes in soils | 3391 | ||
7.4.3.2.3. Weathering based on solutes in groundwater | 3392 | ||
7.4.3.2.4. Weathering based on surface water solutes | 3393 | ||
7.4.4. Normalization of Weathering to Regolith Surface Area | 3393 | ||
7.4.4.1. Definitions of Natural Surface Areas | 3394 | ||
7.4.4.2. Measurements of Specific Surface Areas | 3396 | ||
7.4.4.3. Surface Roughness | 3397 | ||
7.4.5. Tabulations of Weathering Rates of Some Common Silicate Minerals | 3397 | ||
7.4.5.1. Elemental Weathering Fluxes | 3397 | ||
7.4.5.2. Mineral Weathering Fluxes | 3397 | ||
7.4.5.3. Specific Mineral Weathering Rates | 3398 | ||
7.4.6. Time as a Factor in Natural Weathering | 3398 | ||
7.4.6.1. Comparison of Contemporary and Geologic Rates | 3398 | ||
7.4.7. Factors Influencing Natural Weathering Rates | 3402 | ||
7.4.7.1. Mineral Weatherability | 3402 | ||
7.4.7.2. Solute Chemistry and Saturation States | 3403 | ||
7.4.7.3. Coupling the Effect of Hydrology and Chemical Weathering | 3405 | ||
7.4.7.3.1. Low permeability | 3406 | ||
7.4.7.3.2. High permeability | 3408 | ||
7.4.7.4. Role of Climate on Chemical Weathering | 3409 | ||
7.4.7.4.1. Temperature | 3409 | ||
7.4.7.4.2. Precipitation and recharge | 3410 | ||
7.4.7.4.3. Coupling climate effects | 3410 | ||
7.4.7.5. Role of Physical Weathering | 3410 | ||
7.4.8. Summary | 3412 | ||
References | 3413 | ||
Chapter 7.5: Geochemical Weathering in Glacial and Proglacial Environments | 3419 | ||
7.5.1. Introduction | 3419 | ||
7.5.2. Basic Glaciology and Glacier Hydrology | 3420 | ||
7.5.2.1. Glaciers, Ice Caps, and Ice Sheets | 3421 | ||
7.5.2.1.1. Cold or warm ice at the bed? | 3422 | ||
7.5.2.1.2. Sources of water and flow paths | 3422 | ||
7.5.2.1.3. Rock:water ratios and rock-water contact times | 3423 | ||
7.5.2.2. The Proglacial Zone | 3423 | ||
7.5.2.2.1. Broad definition – zone of ice advance and retreat | 3423 | ||
7.5.2.2.2. Permafrost | 3424 | ||
7.5.2.2.3. Seasonal freezing | 3424 | ||
7.5.2.2.4. Evapoconcentration | 3424 | ||
7.5.3. Composition of Glacial Runoff | 3424 | ||
7.5.3.1. General Features in Comparison with Global Riverine Runoff | 3424 | ||
7.5.3.2. Relation to Lithology | 3425 | ||
7.5.3.3. Chemical Erosion Rates | 3425 | ||
7.5.3.4. pH, pCO2 and pO2 | 3425 | ||
7.5.3.5. 87Sr/86Sr Ratios | 3425 | ||
7.5.3.6. Ge/Si Ratios | 3425 | ||
7.5.3.7. δ18O, δ13CDIC, δ34S, and δ18O–SO4 | 3427 | ||
7.5.3.8. Nutrients | 3428 | ||
7.5.4. Geochemical Weathering Reactions in Glaciated Terrain | 3428 | ||
7.5.4.1. Trace Reactive Bedrock Components are Solubilized | 3428 | ||
7.5.4.2. Carbonate and Silicate Hydrolysis | 3428 | ||
7.5.4.3. Cation Exchange | 3428 | ||
7.5.4.4. Carbonate and Silicate Dissolution – Sources of CO2 and Strong Acids | 3428 | ||
7.5.4.5. Sulfide Oxidation – Using O2 and Fe(III) | 3428 | ||
7.5.4.6. Oxidation of Bedrock Organic Matter, Sulfate Reduction, and Onward to Methanogenesis&DEL i | 3429 | ||
7.5.4.7. Nutrients from Glacial Flour | 3429 | ||
7.5.4.8. Other Lithologies | 3430 | ||
7.5.4.9. Little Necessity for Atmospheric CO2 | 3430 | ||
7.5.5. Geochemical Weathering Reactions in the Proglacial Zone | 3430 | ||
7.5.5.1. Similarities with Subglacial Environments | 3430 | ||
7.5.5.2. Evapoconcentration and Freeze Concentration | 3430 | ||
7.5.5.3. Ingress of Water from Channels into the Proglacial Zone | 3430 | ||
7.5.5.4. Enhancement of Glacial Solute Fluxes | 3431 | ||
7.5.6. Composition of Subglacial Waters Beneath Antarctica | 3431 | ||
7.5.7. Concluding Remarks | 3433 | ||
References | 3433 | ||
Chapter 7.6: Chemical Weathering Rates, CO2 Consumption, and Control Parameters Deduced from the Chemical Composition of Rivers | 3437 | ||
7.6.1. Introduction | 3437 | ||
7.6.2. Definition of Chemical Weathering | 3439 | ||
7.6.3. Calculation of CWRs from Field Data | 3439 | ||
7.6.3.1. The Vegetation Compartment | 3441 | ||
7.6.3.2. Role of Atmospheric Deposition | 3441 | ||
7.6.4. Parameters Controlling CWRs | 3442 | ||
7.6.5. Control Parameters Deduced from the Chemical Composition of Rivers | 3443 | ||
7.6.5.1. Field Studies on Small Watersheds | 3443 | ||
7.6.5.1.1. Effect of lithology | 3443 | ||
7.6.5.1.2. Effect of climate (temperature, runoff) | 3446 | ||
7.6.5.1.3. The role of mechanical erosion | 3447 | ||
7.6.5.1.4. Role of organic matter | 3448 | ||
7.6.5.2. Field Studies on Large Watersheds | 3450 | ||
7.6.5.2.1. The direct method | 3450 | ||
7.6.5.2.2. The inversion method | 3451 | ||
7.6.6. Conclusion | 3452 | ||
References | 3453 | ||
Chapter 7.7: Trace Elements in River Waters | 3457 | ||
7.7.1. Introduction | 3457 | ||
7.7.2. Natural Abundances of Trace Elements in River Water | 3458 | ||
7.7.2.1. Range of Concentrations of Trace Elements in River Waters | 3469 | ||
7.7.2.2. Crustal Concentrations versus Dissolved Concentrations in Rivers | 3469 | ||
7.7.2.3. Correlations between Elements | 3471 | ||
7.7.2.4. Temporal Variability | 3472 | ||
7.7.2.5. Conservative Behavior of Trace Elements in River Systems | 3473 | ||
7.7.2.6. Transport of Elements | 3473 | ||
7.7.3. Sources of Trace Elements in Aquatic Systems | 3474 | ||
7.7.3.1. Rock Weathering | 3474 | ||
7.7.3.2. Atmosphere | 3476 | ||
7.7.3.3. Other Anthropogenic Contributions | 3476 | ||
7.7.4. Aqueous Speciation | 3477 | ||
7.7.5. The ``Colloidal World´´ | 3479 | ||
7.7.5.1. Nature of the Colloids | 3479 | ||
7.7.5.2. Ultrafiltration of Colloids and Speciation of Trace Elements in Organic-rich Rivers | 3480 | ||
7.7.5.3. The Nonorganic Colloidal Pool | 3482 | ||
7.7.5.4. Fractionation of REEs in Rivers | 3483 | ||
7.7.5.5. Colloid Dynamics | 3485 | ||
7.7.6. Interaction of Trace Elements with Solid Phases | 3486 | ||
7.7.6.1. Equilibrium Solubility of Trace Elements | 3486 | ||
7.7.6.2. Reactions on Surfaces | 3487 | ||
7.7.6.3. Experimental Adsorption Studies | 3487 | ||
7.7.6.4. Adsorption on Hydrous Oxides in River Systems | 3488 | ||
7.7.6.5. The Sorption of REEs: Competition between Aqueous and Surface Complexation | 3489 | ||
7.7.6.6. Importance of Adsorption Processes in Large River Systems | 3490 | ||
7.7.6.7. Anion Adsorption in Aquatic Systems | 3491 | ||
7.7.6.8. Adsorption and Organic Matter | 3491 | ||
7.7.6.9. Particle Dynamics | 3492 | ||
7.7.7. Conclusion | 3493 | ||
Acknowledgments | 3494 | ||
References | 3494 | ||
Chapter 7.8: Dissolved Organic Matter in Freshwaters | 3499 | ||
7.8.1. Introduction | 3499 | ||
7.8.1.1. Terminology | 3499 | ||
7.8.1.2. Analytical Measurements | 3500 | ||
7.8.1.3. The Major Areas of Research Interest | 3500 | ||
7.8.2. Inventories and Fluxes | 3501 | ||
7.8.2.1. Estimates of Carbon Fluxes – 1970s | 3501 | ||
7.8.2.2. Estimates of Carbon Fluxes – 1980s | 3502 | ||
7.8.2.3. Estimates of Carbon Fluxes – 1990s and 2000s | 3502 | ||
7.8.3. Chemical and Biological Interactions | 3503 | ||
7.8.3.1. DOM and the Acid–Base Chemistry of Freshwaters | 3503 | ||
7.8.3.2. DOM and UV–Visible Radiation | 3504 | ||
7.8.3.3. DOM and Chemical Speciation of Trace Metal Cations | 3505 | ||
7.8.3.4. DOM and Biological Activity of Trace Metal Cations | 3506 | ||
7.8.3.5. Bioavailability of DOM | 3506 | ||
7.8.3.6. DOM and Disinfection By-Products | 3507 | ||
7.8.4. Chemical Properties | 3507 | ||
7.8.4.1. Shapiro's Yellow Organic Acids | 3508 | ||
7.8.4.2. Isolation and Fractionation of DOM | 3508 | ||
7.8.4.2.1. Isolation and fractionation by XAD resins | 3508 | ||
7.8.4.2.2. Isolation and fractionation by membranes | 3510 | ||
7.8.4.3. Average Molecular Weights | 3511 | ||
7.8.4.3.1. Molecular weight distributions and averages | 3511 | ||
7.8.4.3.2. Colligative and noncolligative methods | 3512 | ||
7.8.4.3.3. Statistical summary of average molecular weights | 3512 | ||
7.8.4.3.4. Mass spectrometric analysis of molecular weight distributions | 3514 | ||
7.8.4.4. Elemental Composition | 3514 | ||
7.8.4.4.1. Mass percentages of carbon, hydrogen, nitrogen, oxygen, and sulfur | 3514 | ||
7.8.4.4.2. Atomic ratios | 3516 | ||
7.8.4.4.3. Average oxidation state of organic carbon | 3517 | ||
7.8.4.4.4. Unsaturation and aromaticity | 3517 | ||
7.8.4.5. Acidic Functional Groups | 3517 | ||
7.8.4.5.1. Indirect titration methods | 3517 | ||
7.8.4.5.2. Direct titration methods | 3519 | ||
7.8.4.5.3. Carboxyl and phenolic contents of DOM | 3519 | ||
7.8.4.6. Carbon Distribution from 13C NMR Spectrometry | 3520 | ||
7.8.4.6.1. Processing source data | 3520 | ||
7.8.4.6.2. Statistical summary of 13C NMR results | 3520 | ||
7.8.4.7. Biomolecules in DOM | 3520 | ||
7.8.4.7.1. Amino acids | 3522 | ||
7.8.4.7.2. Sugars | 3522 | ||
7.8.4.7.3. Lignin-derived phenols | 3524 | ||
7.8.4.8. Overall Summary of the Chemical Properties of DOM | 3526 | ||
7.8.5. Summary and Conclusions | 3528 | ||
References | 3529 | ||
Chapter 7.9: Environmental Isotope Applications in Hydrologic Studies | 3535 | ||
7.9.1. Introduction | 3536 | ||
7.9.1.1. Environmental Isotopes as Tracers | 3536 | ||
7.9.1.2. Isotope Fundamentals | 3537 | ||
7.9.1.2.1. Basic principles | 3537 | ||
7.9.1.2.2. Isotope fractionation | 3537 | ||
7.9.1.2.3. Rayleigh fractionation | 3538 | ||
7.9.1.2.4. Terminology | 3538 | ||
7.9.1.3. Causes of Isotopic Variation | 3539 | ||
7.9.1.3.1. Isotope fractionations during phase changes | 3539 | ||
7.9.1.3.2. Mixing of waters and/or solutes | 3539 | ||
7.9.1.3.3. Geochemical and biological reactions | 3540 | ||
7.9.2. Water Sources, Ages, and Cycling | 3541 | ||
7.9.2.1. Deuterium and Oxygen-18 | 3541 | ||
7.9.2.1.1. Basic principles | 3541 | ||
7.9.2.1.2. Precipitation | 3541 | ||
7.9.2.1.3. Shallow groundwaters | 3542 | ||
7.9.2.1.4. Deep groundwaters and paleorecharge | 3543 | ||
7.9.2.1.5. Surface waters | 3543 | ||
7.9.2.2. Tritium | 3545 | ||
7.9.2.2.1. Basic principles | 3545 | ||
7.9.2.2.2. Applications to hydrologic studies | 3545 | ||
7.9.2.3. Determination of Runoff Mechanisms | 3546 | ||
7.9.2.3.1. Mixing models | 3546 | ||
7.9.2.3.2. Isotope hydrograph separation | 3546 | ||
7.9.2.3.2.1. Two-component mixing models | 3547 | ||
7.9.2.3.2.2. Three-component mixing models | 3547 | ||
7.9.2.3.3. Temporal and spatial variability in end-members | 3549 | ||
7.9.2.3.3.1. The ‘event’ water component | 3549 | ||
7.9.2.3.3.2. The ‘pre-event’ groundwater component | 3550 | ||
7.9.2.3.3.3. The soil water component | 3550 | ||
7.9.2.4. Estimation of MRT | 3551 | ||
7.9.3. Solute Isotope Hydrology and Biogeochemistry | 3552 | ||
7.9.3.1. Carbon | 3552 | ||
7.9.3.1.1. Carbon isotope fundamentals | 3553 | ||
7.9.3.1.2. δ13C of soil CO2 and DIC | 3553 | ||
7.9.3.1.3. Tracing sources of carbonate alkalinity in rivers | 3554 | ||
7.9.3.1.4. Tracing sources of carbonate alkalinity in catchments | 3556 | ||
7.9.3.1.5. Carbon-14 | 3557 | ||
7.9.3.1.6. Sources of DOC | 3557 | ||
7.9.3.2. Nitrogen | 3558 | ||
7.9.3.2.1. Nitrogen isotope fundamentals | 3558 | ||
7.9.3.2.2. Oxygen isotope fundamentals | 3559 | ||
7.9.3.2.3. Tracing atmospheric sources of nitrate | 3559 | ||
7.9.3.2.3.1. Using δ15N | 3559 | ||
7.9.3.2.3.2. Using δ18O | 3561 | ||
7.9.3.2.3.3. Using δ17O (or ∆17O) | 3561 | ||
7.9.3.2.4. Fertilizer and animal waste sources of N | 3562 | ||
7.9.3.2.5. Soil sources of N | 3563 | ||
7.9.3.2.6. Processes affecting the δ15N of nitrate | 3563 | ||
7.9.3.2.6.1. N-fixation | 3563 | ||
7.9.3.2.6.2. Assimilation | 3563 | ||
7.9.3.2.6.3. Mineralization | 3564 | ||
7.9.3.2.6.4. Nitrification | 3564 | ||
7.9.3.2.6.5. Volatilization | 3564 | ||
7.9.3.2.6.6. Denitrification | 3564 | ||
7.9.3.2.7. Processes affecting the δ18O of nitrate | 3565 | ||
7.9.3.2.7.1. Assimilation | 3565 | ||
7.9.3.2.7.2. Nitrification | 3565 | ||
7.9.3.2.7.3. Denitrification | 3566 | ||
7.9.3.2.8. Nitrate isotope applications to hydrologic studies | 3566 | ||
7.9.3.2.9. Small catchment studies | 3567 | ||
7.9.3.2.10. Large river studies | 3568 | ||
7.9.3.2.11. Subsurface waters | 3568 | ||
7.9.3.3. Sulfur | 3569 | ||
7.9.3.3.1. Sulfur isotope fundamentals | 3570 | ||
7.9.3.3.2. Oxygen isotopes of sulfate | 3571 | ||
7.9.3.3.3. Tracing atmospheric deposition of sulfate | 3571 | ||
7.9.3.3.3.1. Using δ34S and δ18O | 3571 | ||
7.9.3.3.3.2. Using δ17O (or ∆17O) | 3572 | ||
7.9.3.3.3.3. Using Sulfur-35 | 3572 | ||
7.9.3.3.4. Sulfate in catchment surface waters | 3573 | ||
7.9.3.3.5. Sulfate in groundwater | 3573 | ||
7.9.3.4. Phosphorus | 3574 | ||
7.9.3.4.1. Analytical methods | 3574 | ||
7.9.3.4.2. Factors controlling δ18OPO4 in aquatic ecosystems | 3575 | ||
7.9.3.4.3. Oxygen isotopic compositions of phosphate sources | 3575 | ||
7.9.3.4.3.1. δ18O of phosphate fertilizers | 3575 | ||
7.9.3.4.3.2. δ18O of WWTP phosphate | 3575 | ||
7.9.3.4.3.3. δ18O of soil phosphate | 3576 | ||
7.9.3.4.3.4. δ18O of organic phosphate | 3576 | ||
7.9.3.4.4. Application studies in aquatic ecosystems | 3576 | ||
7.9.4. Use of a Multi-Isotope Approach | 3577 | ||
7.9.4.1. Determination of Flowpaths and Reaction Paths | 3577 | ||
7.9.4.2. Multi-Isotope Studies of Hot Spots and Hot Moments | 3578 | ||
7.9.4.2.1. Isoscapes of nitrate isotope data | 3578 | ||
7.9.4.2.2. Organic matter isotopes | 3579 | ||
7.9.5. Summary and Conclusions | 3579 | ||
Acknowledgments | 3580 | ||
References | 3580 | ||
Chapter 7.10: Metal Stable Isotopes in Weathering and Hydrology | 3591 | ||
7.10.1. Introduction | 3591 | ||
7.10.2. Essential Background Information | 3592 | ||
7.10.2.1. Metal Stable Isotope Fractionation Processes | 3592 | ||
7.10.2.2. Instrumentation | 3593 | ||
7.10.2.2.1. Data: Acquisition, reduction, and accuracy | 3594 | ||
7.10.3. Li, Mg, Ca, and Fe Stable Isotope Signals in the Environment | 3595 | ||
7.10.3.1. Lithium | 3596 | ||
7.10.3.2. Magnesium | 3598 | ||
7.10.3.3. Calcium | 3602 | ||
7.10.3.4. Iron | 3605 | ||
7.10.4. Frontier Metal Stable Isotope Systems | 3609 | ||
7.10.4.1. Strontium | 3610 | ||
7.10.4.2. Copper | 3610 | ||
7.10.4.3. Zinc | 3611 | ||
7.10.4.4. Chromium | 3613 | ||
7.10.5. Directions Forward | 3614 | ||
7.10.5.1. Other Potential Metal Stable Isotope Tracers: The Logical Next Steps | 3615 | ||
7.10.5.2. Getting the Handle on Isotope Fractionation Factors | 3616 | ||
7.10.5.3. Developing Robust Multitracer Approaches | 3616 | ||
References | 3617 | ||
Chapter 7.11: Groundwater Dating and Residence-Time Measurements | 3623 | ||
7.11.1. Introduction | 3624 | ||
7.11.2. Nature of Groundwater Flow Systems | 3624 | ||
7.11.2.1. Driving Forces | 3624 | ||
7.11.2.2. Topographic Control on Flow | 3624 | ||
7.11.2.3. Hydraulic Conductivity and Its Variability | 3625 | ||
7.11.2.4. Scales of Flow Systems | 3625 | ||
7.11.2.4.1. Vadose-zone scale | 3625 | ||
7.11.2.4.2. Local scale | 3625 | ||
7.11.2.4.3. Aquifer scale | 3625 | ||
7.11.2.4.4. Regional scale | 3626 | ||
7.11.2.5. Sources of Solutes at Various Scales | 3626 | ||
7.11.2.5.1. Meteoric (recharge) | 3626 | ||
7.11.2.5.2. Weathering | 3627 | ||
7.11.2.5.3. Diagenetic | 3627 | ||
7.11.2.5.4. Connate | 3627 | ||
7.11.2.5.5. `Basement waters´ | 3627 | ||
7.11.3. Solute Transport in Subsurface Water | 3627 | ||
7.11.3.1. Fundamental Transport Processes | 3628 | ||
7.11.3.1.1. Advection | 3628 | ||
7.11.3.1.2. Diffusion | 3628 | ||
7.11.3.1.3. Dispersion | 3628 | ||
7.11.3.2. Advection–Dispersion Equation | 3628 | ||
7.11.3.3. Interaction between Hydrogeological Heterogeneity and Transport | 3629 | ||
7.11.3.3.1. Small-scale transport and effective dispersion | 3629 | ||
7.11.3.3.2. Large-scale transport and mixing | 3629 | ||
7.11.3.4. Groundwater Dating and the Concept of `Groundwater Age´ | 3629 | ||
7.11.4. Summary of Groundwater Age Tracers | 3630 | ||
7.11.4.1. Introduction | 3630 | ||
7.11.4.2. Radionuclides for Age Tracing of Subsurface Water | 3630 | ||
7.11.4.2.1. Argon-37 | 3630 | ||
7.11.4.2.2. Sulfur-35 | 3631 | ||
7.11.4.2.3. Krypton-85 | 3631 | ||
7.11.4.2.4. Tritium | 3631 | ||
7.11.4.2.5. Silicon-32 | 3633 | ||
7.11.4.2.6. Argon-39 | 3633 | ||
7.11.4.2.7. Carbon-14 | 3633 | ||
7.11.4.2.8. Krypton-81 | 3634 | ||
7.11.4.2.9. Chlorine-36 | 3634 | ||
7.11.4.2.10. Iodine-129 | 3635 | ||
7.11.4.3. Stable, Transient Tracers | 3635 | ||
7.11.4.3.1. Chlorofluorocarbons and sulfur hexafluoride | 3635 | ||
7.11.4.3.2. Atmospheric noble gases and stable isotopes | 3635 | ||
7.11.4.3.3. Nonatmospheric noble gases | 3636 | ||
7.11.5. Lessons from Applying Geochemical Age Tracers to Subsurface Flow and Transport | 3637 | ||
7.11.5.1. Introduction | 3637 | ||
7.11.5.2. Approaches | 3638 | ||
7.11.5.2.1. Direct groundwater age estimation | 3638 | ||
7.11.5.2.2. Modeling techniques: analytical versus numerical | 3638 | ||
7.11.5.2.3. Identification of sources and sinks of a particular tracer | 3639 | ||
7.11.5.2.4. Defining boundary conditions (modeling approach) | 3639 | ||
7.11.6. Tracers at the Regional Scale | 3639 | ||
7.11.6.1. Introduction | 3639 | ||
7.11.6.2. Examples of Applications | 3640 | ||
7.11.6.2.1. Noble-gas isotopes – Paris Basin | 3640 | ||
7.11.6.2.2. Great Artesian Basin – noble-gas isotopes, 36Cl, and 81Kr | 3642 | ||
7.11.6.2.3. Implications at the regional scale | 3644 | ||
7.11.7. Tracers at the Aquifer Scale | 3645 | ||
7.11.7.1. Introduction | 3645 | ||
7.11.7.2. Carrizo Aquifer | 3646 | ||
7.11.7.3. Implications at the Aquifer Scale | 3648 | ||
7.11.8. Tracers at the Local Scale | 3649 | ||
7.11.8.1. Introduction | 3649 | ||
7.11.8.2. 3H/3He, CFC-11, CFC-12, 85Kr – Delmarva Peninsula | 3650 | ||
7.11.8.3. Implications at the Local Scale | 3653 | ||
7.11.9. Tracers in Vadose Zones | 3653 | ||
7.11.10. Conclusions | 3657 | ||
References | 3657 | ||
Chapter 7.12: Cosmogenic Nuclides in Weathering and Erosion | 3663 | ||
7.12.1. Introduction | 3664 | ||
7.12.1.1. Definitions and Nomenclature | 3664 | ||
7.12.1.2. Quantifying Weathering and Erosion | 3664 | ||
7.12.1.2.1. Quantifying physical erosion rates | 3665 | ||
7.12.1.2.2. Quantifying chemical erosion rates | 3665 | ||
7.12.1.2.3. The advent of cosmogenic nuclide methods | 3665 | ||
7.12.2. Cosmogenic Nuclide Systematics at Earth's Surface | 3666 | ||
7.12.2.1. Cosmic Rays | 3666 | ||
7.12.2.2. Cosmogenic Nuclide Production | 3666 | ||
7.12.2.3. Cosmogenic Nuclide Production Profiles | 3667 | ||
7.12.3. Using Cosmogenic Nuclides to Determine Rates of Surface Lowering and Denudation | 3668 | ||
7.12.3.1. No Erosion (Surface Exposure Dating) | 3668 | ||
7.12.3.1.1. Theory | 3668 | ||
7.12.3.1.2. Examples | 3669 | ||
7.12.3.1.2.1. Dating strath terraces | 3669 | ||
7.12.3.1.2.2. Dating glacial moraines | 3669 | ||
7.12.3.2. Rock Erosion | 3670 | ||
7.12.3.2.1. Theory | 3670 | ||
7.12.3.2.1.1. Steady erosion | 3670 | ||
7.12.3.2.1.2. Complications due to unsteady denudation | 3671 | ||
7.12.3.2.2. Examples | 3671 | ||
7.12.3.2.2.1. Denudation of bedrock surfaces | 3671 | ||
7.12.3.2.2.1. Rock erosion under soil cover | 3672 | ||
7.12.3.3. Cosmogenic Nuclides in Vertically Mixed Soils | 3673 | ||
7.12.3.3.1. Theory | 3673 | ||
7.12.3.3.1.1. Exposure dating of vertically mixed sediment | 3674 | ||
7.12.3.3.1.2. Denudation rates in vertically mixed soils | 3674 | ||
7.12.3.3.1.3. Complications due to saprolite weathering and quartz enrichment | 3675 | ||
7.12.3.3.2. Examples | 3676 | ||
7.12.3.3.2.1. Exposure dating marine terraces | 3676 | ||
7.12.3.4. Spatially Averaged Denudation Rates | 3677 | ||
7.12.3.4.1. Theory | 3677 | ||
7.12.3.4.1.1. Complications | 3678 | ||
7.12.3.4.2. Examples | 3678 | ||
7.12.3.4.2.1. Test of the method: comparing cosmogenic rates to absolute erosion rates | 3678 | ||
7.12.3.4.2.2. Transient versus dynamic equilibrium landscapes | 3679 | ||
7.12.4. Chemical Erosion Inferred from Cosmogenic Nuclides | 3681 | ||
7.12.4.1. Chemical Erosion from Physically Stable Soils | 3681 | ||
7.12.4.1.1. Theory | 3681 | ||
7.12.4.1.1.1. Complications | 3682 | ||
7.12.4.1.2. Example | 3683 | ||
7.12.4.1.2.1. Chemical erosion rates of glacial moraines | 3683 | ||
7.12.4.2. Chemical Erosion Rates of Soil Along a Physically Eroding Slope | 3684 | ||
7.12.4.2.1. Theory | 3684 | ||
7.12.4.1.2.1.1. Complications | 3684 | ||
7.12.4.2.2. Example | 3685 | ||
7.12.4.2.2.1. Chemical erosion rates on a hillslope in Australia | 3685 | ||
7.12.4.3. Chemical Erosion Rates of Soils and Landscapes | 3686 | ||
7.12.4.3.1. Theory | 3686 | ||
7.12.4.3.1.1. Complications | 3688 | ||
7.12.4.3.2. Examples | 3689 | ||
7.12.4.3.2.1. Test of the mass balance approach | 3689 | ||
7.12.4.3.2.2. Chemical erosion as a function of altitude | 3689 | ||
7.12.4.3.2.3. Quantifying how chemical and physical erosion interrelate | 3690 | ||
7.12.4.3.2.4. Climatic effects on chemical erosion rates | 3691 | ||
7.12.5. Summary | 3693 | ||
References | 3695 | ||
Chapter 7.13: Geochemistry of Saline Lakes | 3699 | ||
7.13.1. Introduction | 3699 | ||
7.13.2. Origin and Occurrence | 3699 | ||
7.13.3. Environmental Context | 3701 | ||
7.13.3.1. Hydrology | 3701 | ||
7.13.3.2. Climate | 3702 | ||
7.13.3.3. Geology | 3703 | ||
7.13.4. Compositional Controls | 3704 | ||
7.13.4.1. Major Solutes | 3704 | ||
7.13.4.2. Stable Isotopes | 3705 | ||
7.13.5. Evaporative Brine Evolution | 3706 | ||
7.13.6. Examples of Saline Lake Systems | 3712 | ||
7.13.6.1. Chloride – Great Salt Lake | 3718 | ||
7.13.6.2. Carbonate – East Africa | 3719 | ||
7.13.6.3. Sulfate – Northern Great Plains, North America | 3720 | ||
7.13.6.4. Mixed Anions – Death Valley | 3721 | ||
7.13.6.5. Acid Lakes – Australia | 3723 | ||
7.13.7. Economic Minerals in Saline Lakes | 3724 | ||
7.13.8. Summary | 3724 | ||
References | 3725 | ||
Chapter 7.14: Deep Fluids in Sedimentary Basins | 3733 | ||
7.14.1. Introduction | 3734 | ||
7.14.2. Field and Laboratory Methods | 3735 | ||
7.14.2.1. Water from Gas Wells | 3736 | ||
7.14.2.2. Information from Wire-Line Logs | 3737 | ||
7.14.3. Chemical Composition of Subsurface Waters | 3737 | ||
7.14.3.1. Water Salinity | 3737 | ||
7.14.3.1.1. Origin of elevated salinities | 3737 | ||
7.14.3.1.1.1. Subaerial evaporation of seawater | 3737 | ||
7.14.3.1.1.2. Subsurface dissolution of evaporites | 3737 | ||
7.14.3.1.1.3. Marine aerosols: brines from rainwater | 3737 | ||
7.14.3.1.1.4. Freezing | 3737 | ||
7.14.3.1.1.5. Boiling | 3738 | ||
7.14.3.1.2. Controls on salinity | 3739 | ||
7.14.3.1.3. The role of formation waters in crustal cycling | 3739 | ||
7.14.3.2. Major Cations | 3739 | ||
7.14.3.2.1. Dissolved aluminum | 3741 | ||
7.14.3.2.2. Water pH | 3741 | ||
7.14.3.2.3. Dissolved silica | 3743 | ||
7.14.3.2.4. Boron | 3743 | ||
7.14.3.3. Water–Rock Reactions Controlling Cation Concentrations | 3744 | ||
7.14.3.3.1. Cation geothermometry | 3744 | ||
7.14.3.4. Major Anions | 3745 | ||
7.14.3.4.1. Chloride and bromide | 3745 | ||
7.14.3.4.2. Iodide | 3746 | ||
7.14.3.4.3. Fluoride | 3746 | ||
7.14.3.4.4. Inorganic carbon species | 3747 | ||
7.14.3.4.5. Sulfate | 3747 | ||
7.14.3.5. Reactive Organic Species | 3747 | ||
7.14.3.5.1. Monocarboxylic acid anions | 3747 | ||
7.14.3.5.2. Dicarboxylic acid anions | 3748 | ||
7.14.3.5.3. Other reactive organic species | 3748 | ||
7.14.3.5.4. Origin of major reactive species | 3749 | ||
7.14.4. Isotopic Composition of Water | 3749 | ||
7.14.4.1. Formation Waters Derived from Holocene Meteoric Water | 3750 | ||
7.14.4.2. Formation Waters from `Old´ Meteoric Water | 3750 | ||
7.14.4.3. Formation Waters of Connate Marine Origin | 3750 | ||
7.14.4.4. Bittern Connate Water in Evaporites | 3752 | ||
7.14.4.5. Brines from Mixing of Different Waters | 3752 | ||
7.14.4.6. Do the Compositions of Basinal Brines Reflect Secular Variations in Seawater Chemistry? | 3753 | ||
7.14.5. Isotopic Composition of Solutes | 3754 | ||
7.14.5.1. Boron Isotopes | 3754 | ||
7.14.5.2. Lithium Isotopes | 3754 | ||
7.14.5.3. Carbon Isotopes | 3755 | ||
7.14.5.4. Sulfur Isotopes | 3755 | ||
7.14.5.5. Chlorine Isotopes | 3756 | ||
7.14.5.6. Bromine Isotopes | 3756 | ||
7.14.5.7. Strontium Isotopes | 3756 | ||
7.14.5.8. Radioactive Isotopes and Age Dating | 3756 | ||
7.14.6. Basinal Brines as Ore-Forming Fluids | 3757 | ||
7.14.6.1. Metal-Rich Brines | 3757 | ||
7.14.6.2. Solubilization of Heavy Metals | 3757 | ||
7.14.6.3. Bisulfide Complexing | 3757 | ||
7.14.6.4. Organic Complexing | 3758 | ||
7.14.6.5. Chloride Complexing | 3758 | ||
7.14.6.6. Geochemical Modeling of Ore Fluids | 3758 | ||
7.14.6.7. Fluoride | 3759 | ||
7.14.6.8. Barium and Strontium | 3759 | ||
7.14.7. Dissolved Gases | 3759 | ||
7.14.7.1. Methane | 3759 | ||
7.14.7.2. Carbon Dioxide | 3760 | ||
7.14.7.2.1. Storage of CO2 in sedimentary basins | 3760 | ||
7.14.7.2.2. Storage of CO2 in the Frio Formation | 3761 | ||
7.14.7.2.3. Potential impacts and risks of geologic storage of CO2 | 3764 | ||
7.14.7.3. Hydrogen Sulfide | 3767 | ||
7.14.7.4. Noble Gases | 3767 | ||
7.14.8. The Influence of Geologic Membranes | 3768 | ||
7.14.9. Summary and Conclusions | 3769 | ||
Acknowledgments | 3769 | ||
References | 3770 | ||
Chapter 7.15: Deep Fluids in the Continents | 3779 | ||
7.15.1. Introduction | 3780 | ||
7.15.1.1. The Crystalline Rock Environment | 3780 | ||
7.15.1.1.1. The rocks | 3780 | ||
7.15.1.1.2. Structural and geomechanical characteristics | 3780 | ||
7.15.1.1.3. Hydrogeology | 3781 | ||
7.15.2. Field Sampling Methods | 3782 | ||
7.15.3. Chemistry and Isotopic Composition of Groundwaters from Crystalline Environments | 3783 | ||
7.15.3.1. Trends in Major Ions and the Stable Isotopes of Water | 3783 | ||
7.15.3.1.1. Bicarbonate and depth | 3783 | ||
7.15.3.1.2. Sulfate and depth | 3783 | ||
7.15.3.1.3. Chloride and depth | 3789 | ||
7.15.3.1.4. Bromide and depth | 3789 | ||
7.15.3.1.5. Fluoride | 3789 | ||
7.15.3.1.6. Strontium and depth | 3789 | ||
7.15.3.1.7. Oxygen-18 and depth | 3789 | ||
7.15.3.1.8. Oxygen-18 and deuterium | 3789 | ||
7.15.3.2. Strontium Isotopes | 3790 | ||
7.15.3.3. Boron Isotopes | 3792 | ||
7.15.3.4. Stable Chlorine Isotopes (37/35) | 3792 | ||
7.15.3.5. Stable Bromine Isotopes (81/79) | 3793 | ||
7.15.4. Gases from Crystalline Environments | 3793 | ||
7.15.5. The Origin and Evolution of Fluids in Crystalline Environments | 3794 | ||
7.15.5.1. The Origin of Salinity: The Influence of the Rock | 3795 | ||
7.15.5.1.1. Halogen-bearing mineral and fluid inclusions | 3795 | ||
7.15.5.1.2. The role of water/rock interaction | 3796 | ||
7.15.5.1.3. Mineral/fluid reactions | 3797 | ||
7.15.5.1.4. The role of strontium isotopes and water/rock interaction | 3798 | ||
7.15.5.2. The Origin of Salinity: Fluids from External Sources | 3800 | ||
7.15.5.2.1. Sources and concentration mechanisms for fluids from external sources | 3800 | ||
7.15.5.2.1.1. Evaporation of surface waters | 3800 | ||
7.15.5.2.1.2. Freezing of surface and subsurface waters | 3801 | ||
7.15.5.2.2. Emplacement of fluids into crystalline rocks | 3801 | ||
7.15.5.2.3. Major ions and stable isotopes | 3802 | ||
7.15.5.2.4. Evidence for external sources from B, Sr, and Li isotopes | 3802 | ||
7.15.5.3. Examples of Multiple Sources of Salinity | 3803 | ||
7.15.5.3.1. Potential mixing of freeze-out fluids, shield brines, and the influence of gas hydr | 3803 | ||
7.15.5.3.2. Stable chlorine isotope data from the Canadian and Fennoscandian Shields | 3804 | ||
7.15.5.3.3. Interpreting Ca/Na versus Br/Cl trends for data from crystalline environments | 3806 | ||
7.15.6. Examples from Research Sites Found in Crystalline Environments | 3807 | ||
7.15.6.1. Groundwater Composition and Saline Fluids in the Lac du Bonnet Granite Batholith, Manitoba, Canada | 3808 | ||
7.15.6.2. Groundwater Composition at the Palmottu Site in Finland | 3809 | ||
7.15.6.3. Groundwater Composition and Hydrogeochemical Aspects of the Äspö Site, Sweden | 3811 | ||
7.15.6.4. The KTB (Continental Deep Drilling Project of Germany) | 3812 | ||
7.15.6.5. Hydrogeological and Hydrogeochemical Investigations in the Outokumpu Deep Drill Hole in Finlan | 3813 | ||
7.15.6.6. The Hydrogeology and Geochemistry of the Kola Superdeep Well | 3815 | ||
7.15.7. Summary and Conclusions | 3816 | ||
References | 3816 | ||
e9780080983004v8 | 3825 | ||
Front Cover | 3825 | ||
The Oceans and Marine Geochemistry | 3828 | ||
Copyright | 3829 | ||
In Memoriam | 3830 | ||
Heinrich Dieter Holland (1927–2012) | 3832 | ||
Karl Karekin Turekian (1927–2013) | 3834 | ||
References | 3836 | ||
Dedication | 3838 | ||
Contents | 3840 | ||
Executive Editors’ Foreword to the Second Edition | 3842 | ||
Contributors | 3846 | ||
Volume Editor’s Introduction | 3848 | ||
References | 3850 | ||
Chapter 8.1: Physico-Chemical Controls on Seawater | 3852 | ||
8.1.1. Composition of Seawater | 3852 | ||
8.1.2. Thermodynamic Properties of Seawater | 3853 | ||
8.1.3. Thermodynamic Equilibria in Seawater | 3855 | ||
8.1.3.1. The Carbonate System in Seawater | 3855 | ||
8.1.3.2. Other Ionic Equilibrium Processes | 3856 | ||
8.1.4. Kinetic Processes in Seawater | 3857 | ||
8.1.5. Modeling the Ionic Interactions in Natural Waters | 3857 | ||
8.1.5.1. Estimating Activity Coefficients | 3857 | ||
8.1.5.2. Estimating Physical-Chemical Properties | 3858 | ||
8.1.6. Effect of Ocean Acidification | 3859 | ||
Acknowledgments | 3865 | ||
References | 3865 | ||
Chapter 8.2: Controls of Trace Metals in Seawater | 3870 | ||
8.2.1. Introduction | 3870 | ||
8.2.1.1. Concentrations | 3871 | ||
8.2.1.2. Distributions | 3874 | ||
8.2.1.2.1. Conservative-type distributions | 3874 | ||
8.2.1.2.2. Nutrient-type distributions | 3875 | ||
8.2.1.2.3. Scavenged-type distributions | 3876 | ||
8.2.1.2.4. Hybrid distributions | 3876 | ||
8.2.1.2.5. Mixed distributions | 3877 | ||
8.2.1.2.6. Data emerging from the GEOTRACES program | 3878 | ||
8.2.2. External Inputs of Trace Metals to the Oceans | 3880 | ||
8.2.2.1. Rivers | 3880 | ||
8.2.2.2. Benthic Inputs | 3881 | ||
8.2.2.3. Atmosphere | 3881 | ||
8.2.2.4. Hydrothermal | 3884 | ||
8.2.3. Removal Processes | 3886 | ||
8.2.3.1. Active Biological Uptake in the Surface Waters | 3886 | ||
8.2.3.1.1. Lessons from laboratory studies | 3886 | ||
8.2.3.1.2. Non-Redfieldian assimilation | 3887 | ||
8.2.3.2. Passive Scavenging | 3888 | ||
8.2.3.2.1. Adsorption/desorption processes | 3888 | ||
8.2.3.2.2. Lessons from radionuclides | 3888 | ||
8.2.4. Internal Recycling | 3890 | ||
8.2.4.1. Recycling within the Water Column | 3890 | ||
8.2.4.2. Recycling at the Sediment/Seawater Interface | 3890 | ||
8.2.5. Complexation with Organic Ligands | 3891 | ||
8.2.5.1. Copper | 3892 | ||
8.2.5.2. Iron | 3893 | ||
8.2.5.3. Zinc | 3894 | ||
8.2.5.4. Summary of Speciation | 3894 | ||
8.2.6. Future Directions | 3895 | ||
8.2.6.1. The Use of Trace Element Isotopes | 3895 | ||
8.2.6.2. Ocean Acidification | 3895 | ||
References | 3896 | ||
Relevant Websites | 3902 | ||
Chapter 8.3: Air-Sea Exchange of Marine Trace Gases | 3904 | ||
8.3.1. Introduction | 3905 | ||
8.3.2. Gas Exchange Processes and Parameterizations | 3905 | ||
8.3.2.1. Gas Flux Theory | 3905 | ||
8.3.2.1.1. Mass boundary layers at the interface | 3905 | ||
8.3.2.1.2. Turbulent forcing: wind speed-based parameterizations of transfer velocity | 3906 | ||
8.3.2.1.3. Interfacial models and the Schmidt number exponent on the water side | 3907 | ||
8.3.2.1.4. Air-side transfer | 3908 | ||
8.3.2.2. Processes Driving Gas Exchange | 3908 | ||
8.3.2.2.1. The effect of wind | 3908 | ||
8.3.2.2.2. The effect of waves | 3908 | ||
8.3.2.2.3. The effect of bubbles and sea spray | 3909 | ||
8.3.2.2.4. Heat and water fluxes | 3910 | ||
8.3.2.2.5. The effect of surface films | 3911 | ||
8.3.2.2.6. Chemical enhancement | 3912 | ||
8.3.2.2.7. Biological effects | 3912 | ||
8.3.2.3. Wind Speed Parameterizations | 3913 | ||
8.3.2.3.1. Global 14C constraint | 3913 | ||
8.3.2.3.2. Local-scale natural tracer experiments | 3914 | ||
8.3.2.3.2.1. Radon-deficit technique | 3914 | ||
8.3.2.3.2.2. Noble gas technique | 3914 | ||
8.3.2.3.2.3. Biogenic gas mass balance | 3915 | ||
8.3.2.3.3. Deliberate tracer experiments | 3915 | ||
8.3.2.3.4. Direct flux measurements | 3916 | ||
8.3.2.3.5. NOAA/COARE | 3917 | ||
8.3.2.3.6. Reconciling observations | 3918 | ||
8.3.2.3.7. Remote sensing of kw | 3919 | ||
8.3.2.3.8. Air-side transfer velocity | 3920 | ||
8.3.2.4. Estimating Trace Gas Fluxes in Biogeochemical Studies | 3920 | ||
8.3.2.4.1. Gas-specific effects on transfer | 3920 | ||
8.3.2.4.2. Selection of wind speed parameterization | 3921 | ||
8.3.2.4.3. Averaging and interpolation/extrapolation | 3922 | ||
8.3.2.4.4. Uncertainty in estimates | 3923 | ||
8.3.2.5. Future Developments | 3923 | ||
8.3.3. The Cycling of Trace Gases Across the Air-Sea Interface | 3923 | ||
8.3.3.1. Greenhouse Gases | 3924 | ||
8.3.3.1.1. Carbon dioxide | 3924 | ||
8.3.3.1.2. Methane | 3925 | ||
8.3.3.1.3. Nitrous oxide | 3925 | ||
8.3.3.1.4. Ozone | 3925 | ||
8.3.3.1.5. Carbon monoxide | 3926 | ||
8.3.3.2. Nitrogen-Containing Gases | 3926 | ||
8.3.3.2.1. Ammonia and methylamines | 3926 | ||
8.3.3.2.2. Alkyl nitrates | 3926 | ||
8.3.3.2.3. Hydrogen cyanide and methyl cyanide | 3927 | ||
8.3.3.3. Sulfur-Containing Gases | 3927 | ||
8.3.3.3.1. Dimethyl sulfide | 3927 | ||
8.3.3.3.2. Methyl mercaptan | 3930 | ||
8.3.3.3.3. Carbonyl sulfide | 3930 | ||
8.3.3.3.4. Carbon disulfide | 3930 | ||
8.3.3.3.5. Hydrogen sulfide | 3930 | ||
8.3.3.3.6. Sulfur dioxide | 3930 | ||
8.3.3.4. Nonmethane Hydrocarbons | 3930 | ||
8.3.3.5. Oxygenated Volatile Organic Compounds | 3931 | ||
8.3.3.6. Organohalogens | 3931 | ||
8.3.3.6.1. The mono-organohalogens: methyl, ethyl, and propyl iodide&INS id= | 3931 | ||
8.3.3.6.2. The di-organohalogens: diiodomethane, chloroiodomethane, bromoiodomethane,& | 3933 | ||
8.3.3.6.3. The tri-organohalogens: bromoform and chloroform | 3933 | ||
8.3.3.7. Miscellaneous Gases | 3934 | ||
8.3.3.7.1. Chlorofluorocarbons | 3934 | ||
8.3.3.7.2. Synthetic organic compounds | 3934 | ||
8.3.3.7.3. Mercury | 3934 | ||
8.3.3.7.4. Selenium and polonium | 3934 | ||
8.3.3.7.5. Hydrogen | 3935 | ||
8.3.4. Effects of Climate Change on Marine Trace Gases | 3935 | ||
8.3.4.1. Effect on Air-Sea Gas Transfer | 3935 | ||
8.3.4.2. Effect on Dissolved Gas Concentrations | 3935 | ||
8.3.4.2.1. Carbon dioxide | 3935 | ||
8.3.4.2.2. Dimethyl sulfide | 3935 | ||
8.3.4.2.3. Other gases | 3935 | ||
References | 3936 | ||
Chapter 8.4: The Biological Pump | 3944 | ||
List of Symbols | 3945 | ||
8.4.1. Introduction | 3945 | ||
8.4.2. Description of the Biological Pump | 3946 | ||
8.4.2.1. Photosynthesis and the Incorporation of Nutrients and Trace Elements into Biogenic Materials | 3947 | ||
8.4.2.1.1. Levels of primary production | 3948 | ||
8.4.2.1.2. Patterns in time and space | 3948 | ||
8.4.2.1.3. Nutrient limitation | 3949 | ||
8.4.2.2. Formation of Sinking Particles | 3949 | ||
8.4.2.2.1. Marine snow | 3949 | ||
8.4.2.2.2. Aggregation and exopolymers | 3949 | ||
8.4.2.2.3. Role of zooplankton | 3950 | ||
8.4.2.2.3.1. Mucus feeding structures | 3950 | ||
8.4.2.2.3.2. Fecal pellets | 3950 | ||
8.4.2.3. Transport of Material to Depth | 3950 | ||
8.4.2.3.1. The flux of POC | 3950 | ||
8.4.2.3.1.1. Controls on particle sinking velocities | 3951 | ||
8.4.2.3.2. Diel vertical migration of zooplankton | 3951 | ||
8.4.2.4. Particle Decomposition, Fragmentation, and Repackaging | 3951 | ||
8.4.2.4.1. Impacts of zooplankton | 3953 | ||
8.4.2.4.2. Impact of bacteria | 3953 | ||
8.4.2.5. Sedimentation and Burial | 3954 | ||
8.4.2.6. Dissolved Organic Matter, the Microbial Loop, and the Microbial Carbon Pump | 3954 | ||
8.4.2.7. New, Export, and Regenerated Production | 3955 | ||
8.4.2.8. The Ballast Hypothesis | 3955 | ||
8.4.3. Impact of the Biological Pum | 3955 | ||
8.4.3.1. Carbon | 3955 | ||
8.4.3.2. Nitrogen | 3956 | ||
8.4.3.2.1. Nitrogen fixation versus denitrification | 3956 | ||
8.4.3.3. Phosphorus | 3957 | ||
8.4.3.4. Silicon | 3957 | ||
8.4.3.4.1. The impact of the appearance of the diatoms on the marine silica cycle | 3957 | ||
8.4.3.4.2. Excessive pumping of silicon | 3958 | ||
8.4.4. Quantifying the Biological Pump | 3958 | ||
8.4.4.1. Measurement of New Production and Export Production | 3959 | ||
8.4.4.2. Measurement of Particle Flux | 3959 | ||
8.4.4.2.1. Sediment traps | 3959 | ||
8.4.4.2.2. Particle-reactive nuclides | 3960 | ||
8.4.4.2.3. Oxygen utilization rates | 3960 | ||
8.4.5. The Efficiency of the Biological Pump | 3961 | ||
8.4.5.1. Definition of the Efficiency of the Biological Pump | 3961 | ||
8.4.5.2. Geographic Variability in the Efficiency of the Biological Pump | 3961 | ||
8.4.5.3. Altering the Efficiency of the Biological Pump | 3962 | ||
8.4.5.3.1. Fertilization with micronutrients in HNLC areas | 3962 | ||
8.4.5.3.2. Translocation of nutrients | 3963 | ||
8.4.5.3.3. Changes in community composition | 3963 | ||
8.4.5.3.4. Effects of the calcium carbonate counter-pump | 3963 | ||
8.4.5.3.5. Enhancing particle transport | 3964 | ||
8.4.5.3.6. Carbon overconsumption | 3964 | ||
8.4.5.3.7. The C:N:P ratios of sinking material | 3964 | ||
8.4.6. The Biological Pump in the Immediate Future | 3965 | ||
References | 3966 | ||
Chapter 8.5: Marine Bioinorganic Chemistry: The Role of Trace Metals in the Oceanic Cycles of Major Nutrients | 3974 | ||
8.5.1. Introduction: The Scope of Marine Bioinorganic Chemistry | 3974 | ||
8.5.2. Trace Metals in Marine Microorganisms | 3975 | ||
8.5.2.1. Concentrations | 3975 | ||
8.5.2.2. Uptake | 3977 | ||
8.5.2.3. Trace Element Storage | 3982 | ||
8.5.3. The Biochemical Functions of Trace Elements in the Uptake and Transformations of Nutrients | 3982 | ||
8.5.3.1. Trace Metals and the Marine Carbon Cycle | 3983 | ||
8.5.3.1.1. Light reaction of photosynthesis | 3983 | ||
8.5.3.1.2. Dark reaction of photosynthesis | 3983 | ||
8.5.3.1.3. Carbon concentrating mechanisms | 3984 | ||
8.5.3.1.4. Respiration | 3986 | ||
8.5.3.2. Trace Metals and the Nitrogen Cycle | 3986 | ||
8.5.3.2.1. Acquisition of fixed nitrogen by phytoplankton | 3986 | ||
8.5.3.2.2. N2 fixation and the nitrogen cycle | 3987 | ||
8.5.3.3. Phosphorus Uptake | 3987 | ||
8.5.3.4. Silicon Uptake | 3988 | ||
8.5.4. Effects of Trace Metals on Marine Biogeochemical Cycles | 3988 | ||
8.5.4.1. Iron | 3988 | ||
8.5.4.1.1. Iron and growth rates | 3988 | ||
8.5.4.1.2. Iron uptake | 3990 | ||
8.5.4.1.3. Iron and electron transfer | 3990 | ||
8.5.4.1.4. Iron and nitrogen acquisition | 3990 | ||
8.5.4.2. Manganese | 3990 | ||
8.5.4.3. Zinc, Cobalt, and Cadmium | 3991 | ||
8.5.4.4. Copper | 3994 | ||
8.5.4.5. Nickel | 3996 | ||
8.5.5. Epilogue | 3997 | ||
8.5.5.1. Paleoceanographic Aspects | 3997 | ||
8.5.5.2. A View to the Future | 3997 | ||
Acknowledgments | 3998 | ||
References | 3998 | ||
Chapter 8.6: Organic Matter in the Contemporary Ocean | 4002 | ||
8.6.1. Introduction | 4002 | ||
8.6.2. Reservoirs and Fluxes | 4003 | ||
8.6.2.1. Reservoirs | 4003 | ||
8.6.2.2. Fluxes | 4004 | ||
8.6.2.2.1. Terrigenous OM fluxes to the oceans | 4004 | ||
8.6.2.2.1.1. Riverine fluxes | 4004 | ||
8.6.2.2.1.2. Eolian fluxes | 4005 | ||
8.6.2.2.2. Water-column fluxes and the burial of OC in sedimen | 4005 | ||
8.6.3. The Nature and Fate of TOC Delivered to the Oceans | 4005 | ||
8.6.3.1. Background | 4005 | ||
8.6.3.2. Terrestrial OM in River Systems | 4006 | ||
8.6.3.3. Quantitative Importance of TOC in Marine Sediments | 4008 | ||
8.6.3.3.1. Black carbon | 4010 | ||
8.6.4. Origin, Cycling, Composition, and Fate of DOC in the Ocean | 4011 | ||
8.6.4.1. Background | 4011 | ||
8.6.4.2. Transport and Cycling of DOC in the Ocean | 4011 | ||
8.6.4.3. Recalcitrant DOC | 4013 | ||
8.6.4.4. The Composition of Semilabile HMW DOM: Biopolymers or Geopolymers? | 4014 | ||
8.6.4.4.1. Acylpolysaccharides as major components of HMW DOM | 4015 | ||
8.6.4.4.2. Proteins in HMW DOM | 4018 | ||
8.6.4.4.3. Gel polymers and the cycling of HMW DOM | 4018 | ||
8.6.4.5. Labile DOC and the Microbial Loop | 4019 | ||
8.6.5. Emerging Perspectives on OM Preservation | 4022 | ||
8.6.5.1. Background | 4022 | ||
8.6.5.2. Compositional Transformations Associated with Sedimentation and Burial of OM | 4022 | ||
8.6.5.3. Controls on OM Preservation | 4025 | ||
8.6.5.3.1. Physical protection | 4026 | ||
8.6.5.3.2. Role of oxygen | 4027 | ||
8.6.5.3.3. Chemical protection | 4029 | ||
8.6.5.3.3.1. Intrinsically refractory biomolecules | 4029 | ||
8.6.5.3.3.2. Formation of organosulfur compounds | 4029 | ||
8.6.6. Microbial OM Production and Processing: New Insights | 4030 | ||
8.6.6.1. Background | 4030 | ||
8.6.6.2. Planktonic Archaea | 4031 | ||
8.6.6.3. Anaerobic Oxidation of Methane | 4031 | ||
8.6.7. Summary and Future Research Directions | 4032 | ||
Acknowledgments | 4034 | ||
References | 4034 | ||
Chapter 8.7: Hydrothermal Processes | 4042 | ||
8.7.1. Introduction | 4043 | ||
8.7.1.1. What Is Hydrothermal Circulation? | 4043 | ||
8.7.1.2. Where Does Hydrothermal Circulation Occur? | 4045 | ||
8.7.1.3. Why Should Hydrothermal Fluxes Be Considered Important? | 4046 | ||
8.7.1.4. Outline Structure for Rest of this Chapter | 4047 | ||
8.7.2. Vent-Fluid Geochemistry | 4048 | ||
8.7.2.1. Why Are Vent-Fluid Compositions of Interest? | 4048 | ||
8.7.2.2. Processes Affecting Vent-Fluid Compositions | 4048 | ||
8.7.2.3. Compositions of Hydrothermal Vent Fluids | 4052 | ||
8.7.2.3.1. Major element chemistry | 4052 | ||
8.7.2.3.2. Trace element and isotope chemistry | 4053 | ||
8.7.2.3.3. Gas chemistry of hydrothermal fluids | 4054 | ||
8.7.2.3.4. Organic geochemistry of hydrothermal vent fluids | 4055 | ||
8.7.2.4. Geographic Variations in Vent-Fluid Compositions | 4056 | ||
8.7.2.4.1. Basalt-hosted hydrothermal systems | 4056 | ||
8.7.2.4.2. Ultramafic-hosted systems | 4058 | ||
8.7.2.4.3. Back-arc systems | 4059 | ||
8.7.2.5. Temporal Variability in Vent-Fluid Compositions | 4061 | ||
8.7.2.5.1. Temporal variability | 4061 | ||
8.7.2.5.2. Effect on flux estimates | 4062 | ||
8.7.3. The Net Impact of Hydrothermal Activity | 4063 | ||
8.7.4. Near-Vent Deposits | 4064 | ||
8.7.4.1. Alteration and Mineralization of the Upper Ocean Crust | 4064 | ||
8.7.4.2. Near-Vent Hydrothermal Deposits | 4064 | ||
8.7.5. Hydrothermal Plume Processes | 4066 | ||
8.7.5.1. Dynamics of Hydrothermal Plumes | 4066 | ||
8.7.5.2. Modification of Gross Geochemical Fluxes | 4067 | ||
8.7.5.2.1. Dissolved noble gases | 4067 | ||
8.7.5.2.2. Dissolved reduced gases (H2S, H2, and CH4) | 4068 | ||
8.7.5.2.3. Fe and Mn geochemistry in hydrothermal plumes | 4068 | ||
8.7.5.2.4. Coprecipitation and uptake with Fe in buoyant and nonbuoyant plumes | 4071 | ||
8.7.5.2.5. Hydrothermal scavenging by Fe oxyhydroxides | 4071 | ||
8.7.5.3. Physical Controls on Dispersing Plumes | 4072 | ||
8.7.5.4. Biogeochemical Cycling in Hydrothermal Plumes | 4073 | ||
8.7.5.5. Impact of Hydrothermal Plumes on Ocean Geochemical Cycles | 4074 | ||
8.7.6. Hydrothermal Sediments | 4075 | ||
8.7.6.1. Near-Vent Sediments | 4075 | ||
8.7.6.2. Deposition from Hydrothermal Plumes | 4075 | ||
8.7.6.3. Hydrothermal Sediments in Paleoceanography | 4076 | ||
8.7.6.4. Hydrothermal Sediments and Boundary Scavenging | 4076 | ||
8.7.7. Conclusion | 4076 | ||
8.7.7.1. What Are the Integrated Fluxes of Hydrothermal Heat and Mass from a Single Vent System to the Surrounding Ocean? | 4077 | ||
8.7.7.2. What Role Does Protolith Composition Play in Hydrothermal Vent-Fluid Chemistry? | 4077 | ||
8.7.7.3. What Is the Impact of Seafloor Hydrothermal Venting on Global Ocean Biogeochemical Budgets? | 4077 | ||
Remembrance | 4077 | ||
References | 4078 | ||
Chapter 8.8: Tracers of Ocean Mixing | 4086 | ||
8.8.1. Introduction | 4086 | ||
8.8.2. Theoretical Framework 1: Advection–Diffusion Equations | 4088 | ||
8.8.3. The Nature of Oceanic Mixing | 4089 | ||
8.8.3.1. Diapycnal Mixing in the Ocean | 4090 | ||
8.8.3.2. Isopycnal Mixing in the Ocean: | 4091 | ||
8.8.4. Theoretical Framework 2: Tracer Ages | 4091 | ||
8.8.4.1. Radiometric Dating | 4092 | ||
8.8.4.2. Transient Concentration Dating | 4093 | ||
8.8.5. Theoretical Framework 3: Diagnostic Methods | 4094 | ||
8.8.5.1. Optimum Multiparameter Analysis | 4094 | ||
8.8.5.2. Transit Time Distributions | 4095 | ||
8.8.5.3. Tracer Contour Inverse Analysis | 4096 | ||
8.8.6. Steady-State Tracers | 4097 | ||
8.8.6.1. Radiocarbon | 4097 | ||
8.8.6.2. Radon-222 | 4098 | ||
8.8.6.3. Radium | 4098 | ||
8.8.6.4. Argon-39 | 4099 | ||
8.8.6.5. Dissolved Noble Gas Saturation Anomalies | 4099 | ||
8.8.7. Transient Tracers | 4100 | ||
8.8.8. Tracer Age Dating | 4103 | ||
8.8.9. Tracer Release Exp | 4104 | ||
8.8.10. Concluding Remarks | 4104 | ||
References | 4105 | ||
Chapter 8.9: Chemical Tracers of Particle Transport | 4110 | ||
8.9.1. Particle Transport and Ocean Biogeochemistry | 4110 | ||
8.9.2. Tracers of Particle Transport | 4111 | ||
8.9.3. Transfer from Solution to Particles (Scavenging) | 4111 | ||
8.9.4. Colloidal Intermediaries | 4113 | ||
8.9.4.1. Development of the Colloidal Pumping Hypothesis | 4113 | ||
8.9.4.2. Important Features of Colloids | 4114 | ||
8.9.4.3. Evidence for Coagulation of Colloids and of Colloidal Thorium (and other Metals) | 4115 | ||
8.9.4.4. Rate Constants for Colloid Coagulation | 4115 | ||
8.9.4.5. Limitations and Questions | 4117 | ||
8.9.5. Export of Particles from Surface Ocean Waters | 4117 | ||
8.9.5.1. Scavenging Rates and Particle Flux | 4117 | ||
8.9.5.2. Export Flux of POC | 4118 | ||
8.9.5.3. Non-Steady-State Conditions and Advected Fluxes | 4118 | ||
8.9.5.4. Limitations and Prospects | 4119 | ||
8.9.6. Particle Dynamics and Regeneration of Labile Particles | 4120 | ||
8.9.6.1. Particle Dynamics, Fluxes, and Regeneration | 4120 | ||
8.9.6.2. Conceptual Models of Aggregation and Disaggregation | 4120 | ||
8.9.6.3. Strategies to Evaluate Rate Constants | 4121 | ||
8.9.7. Lateral Redistribution of Sediments | 4123 | ||
8.9.7.1. Focusing and Winnowing of Sediments | 4123 | ||
8.9.7.2. Lead-210 | 4124 | ||
8.9.7.3. Thorium-230 | 4124 | ||
8.9.7.4. Helium-3 | 4126 | ||
8.9.8. Summary | 4128 | ||
References | 4128 | ||
Chapter 8.10: Biological Fluxes in the Ocean and Atmospheric pCO2 | 4132 | ||
8.10.1. Introduction | 4132 | ||
8.10.2. How Atmospheric CO2 is Affected by the Biological Pump | 4133 | ||
8.10.2.1. By Internal Rearrangement of the Chemis | 4133 | ||
8.10.2.2. Changes to the Mean Chemical State of the Ocean | 4134 | ||
8.10.3. Visions of the Biological Pump in the Ocean | 4135 | ||
8.10.3.1. Global Rate of Carbon Export from the Euphotic Zone | 4135 | ||
8.10.3.1.1. Sediment traps | 4135 | ||
8.10.3.1.2. Bottles | 4135 | ||
8.10.3.1.3. Ocean carbon cycle GCMs | 4135 | ||
8.10.3.2. Organic Carbon Sinking Depth | 4135 | ||
8.10.3.2.1. Observations | 4135 | ||
8.10.3.2.2. Theory | 4136 | ||
8.10.3.2.2.1. Zooplankton | 4136 | ||
8.10.3.2.2.2. Aggregation | 4136 | ||
8.10.3.2.2.3. Ballast | 4137 | ||
8.10.3.2.2.4. Water column dissolution | 4137 | ||
8.10.3.3. The CaCO3 Pump | 4137 | ||
8.10.3.4. The SiO2 Pump | 4138 | ||
8.10.4. How the Biological Pump Could Change | 4139 | ||
8.10.4.1. Iron | 4139 | ||
8.10.4.2. pH | 4140 | ||
8.10.4.3. Transparent Exopolymers | 4140 | ||
8.10.4.4. Climate Change Impacts | 4140 | ||
8.10.4.5. Global Nutrient Inventories | 4140 | ||
8.10.5. Conclusion | 4141 | ||
References | 4141 | ||
Chapter 8.11: Sedimentary Diagenesis, Depositional Environments, and Benthic Fluxes | 4144 | ||
8.11.1. Introduction | 4144 | ||
8.11.1.1. Early Diagenesis and the Global Sedimentary Cycle | 4144 | ||
8.11.1.2. General Classes of Conceptual Models | 4145 | ||
8.11.1.3. Diagenetic Regimes and Depositional Environments | 4145 | ||
8.11.2. Diagenetic Oxidation-Reduction Reactions | 4149 | ||
8.11.2.1. Biogeochemical Redox Reaction Sequence | 4149 | ||
8.11.2.1.1. Coupled stoichiometric relations | 4150 | ||
8.11.2.1.2. Pore water solutes as reaction indicators | 4151 | ||
8.11.2.2. Reaction Rates and Kinetics | 4151 | ||
8.11.2.3. Sedimentary Redox Reaction Patterns | 4152 | ||
8.11.2.3.1. Redox oscillation | 4154 | ||
8.11.2.3.2. Microenvironmental heterogeneity | 4154 | ||
8.11.3. Diagenetic Transport Processes | 4155 | ||
8.11.3.1. Diffusive Transport | 4155 | ||
8.11.3.2. Advective Transport | 4156 | ||
8.11.4. Diagenetic Transport-Reaction Models | 4156 | ||
8.11.4.1. General Mass Balance Relations and Reference Frames | 4156 | ||
8.11.4.2. Simplification of Diagenetic Models | 4157 | ||
8.11.4.2.1. Characteristic scaling and steady state | 4157 | ||
8.11.5. Patterns in Boundary Conditions and Reaction Balances | 4158 | ||
8.11.5.1. Spatial Patterns in Sediment Accumulation and Biogenic Transport | 4158 | ||
8.11.5.2. Spatial Patterns in Reactive Corg Delivery and Magnitudes of Benthic Fluxes | 4159 | ||
8.11.5.3. Global Patterns in the Scaling of Redox Reactions | 4160 | ||
8.11.5.4. Sedimentary Record of Diagenetic Reaction Dominance and Balances | 4160 | ||
8.11.5.5. Temporal Patterns in Boundary Conditions and the Scaling of Reactions | 4162 | ||
8.11.6. Corg Burial and Preservation: Reactants and Diagenetic Regime | 4164 | ||
8.11.6.1. Patterns in Corg Distributions and Particle Associations | 4164 | ||
8.11.6.2. Corg Preservation: O2, Accumulation Rates, and Diagenetic Regimes | 4166 | ||
8.11.7. Carbonate Mineral Dissolution-Alteration-Preservation | 4168 | ||
8.11.7.1. Coupling of Redox Reactions and Carbonate Diagenesis | 4168 | ||
8.11.7.2. Carbonate Mineral Equilibria and Saturation States | 4168 | ||
8.11.7.3. Kinetics of Biogenic Carbonate Dissolution in Sediments | 4169 | ||
8.11.7.4. Shallow Water Carbonate Dissol | 4171 | ||
8.11.7.5. Dissolution of Carbonate in Deep-Sea Deposits and Internal Reaction Patterns | 4172 | ||
8.11.7.6. Benthic Alkalinity Fluxes | 4172 | ||
8.11.8. Biogenic Silica and Reverse Weathering | 4175 | ||
8.11.8.1. Patterns in Biogenic SiO2 Distributions | 4175 | ||
8.11.8.2. Diagenetic Fates, Equilibria, and Dissolution Reaction Kinetics of Biogenic SiO2 | 4175 | ||
8.11.8.3. Authigenic Silicate Formation and Reverse Weathering | 4177 | ||
8.11.9. Future Directions | 4179 | ||
Acknowledgments | 4179 | ||
References | 4180 | ||
Chapter 8.12: Geochronometry of Marine Deposits | 4186 | ||
8.12.1. Introduction | 4186 | ||
8.12.2. Principles | 4186 | ||
8.12.2.1. Radioactive Geochronometry | 4186 | ||
8.12.2.2. Secondary Stratigraphic Procedures | 4187 | ||
8.12.3. Radioactive Systems Used in Marine Geochronometry | 4187 | ||
8.12.3.1. Radiocarbon | 4187 | ||
8.12.3.2. Uranium and Thorium Decay Chain Nuclides | 4187 | ||
8.12.3.3. Cosmogenic Nuclides | 4187 | ||
8.12.3.4. Potassium-Argon | 4188 | ||
8.12.4. Coastal Deposits | 4188 | ||
8.12.4.1. Applicable Methods and Requirements | 4188 | ||
8.12.4.2. Unbioturbated Deposits | 4188 | ||
8.12.4.3. Bioturbated Deposits | 4189 | ||
8.12.5. Deep-Sea Sediments | 4190 | ||
8.12.5.1. Radiocarbon | 4190 | ||
8.12.5.2. 230Th and 231Pa | 4191 | ||
8.12.5.2.1. The basic theory | 4191 | ||
8.12.5.2.2. The underlying assumptions | 4192 | ||
8.12.5.2.3. Applications | 4192 | ||
8.12.5.2.4. Problems of erosion and focusing | 4193 | ||
8.12.5.3. 10Be | 4193 | ||
8.12.5.4. 3He | 4194 | ||
8.12.5.5. Volcanic Layers | 4195 | ||
8.12.5.6. Extension of Dating Techniques | 4195 | ||
8.12.5.6.1. The Milankovitch cycles and chronology | 4195 | ||
8.12.5.6.2. Oxygen and carbon isotopes in carbonate tests | 4195 | ||
8.12.5.6.3. Magnetic-reversal stratigraphy | 4196 | ||
8.12.5.6.4. Element accumulation: Titanium and cobalt | 4196 | ||
8.12.6. Ferromanganese Deposits | 4197 | ||
8.12.6.1. Applicable Methods | 4197 | ||
8.12.6.2. The Underlying Assumptions | 4197 | ||
8.12.6.3. Applications | 4197 | ||
8.12.7. Corals | 4198 | ||
8.12.8. Methods Not Depending on Radioactive Decay | 4200 | ||
8.12.8.1. Amino Acid Racemization | 4200 | ||
8.12.8.2. Thermoluminescence | 4200 | ||
Acknowledgments | 4201 | ||
References | 4201 | ||
Chapter 8.13: Geochemical Evidence for Quaternary Sea-Level Changes | 4206 | ||
8.13.1. Introduction | 4206 | ||
8.13.2. Methods of Sea-Level Reconstruction | 4206 | ||
8.13.2.1. Methods of Direct Sea-Level Reconstruction | 4207 | ||
8.13.2.2. Methods of Sea-Level Reconstruction from Oxygen Isotope Measurements | 4207 | ||
8.13.3. History and Current State of Direct Sea-Level Reconstruction | 4209 | ||
8.13.3.1. 230Th and 231Pa Dating: Current Status and Historical Overview | 4209 | ||
8.13.3.2. 230Th and 231Pa Dating: Theory | 4210 | ||
8.13.3.3. Tests of Dating Assumptions | 4211 | ||
8.13.3.4. Sources of Error in Age | 4212 | ||
8.13.3.5. Open-System 230Th Dating | 4214 | ||
8.13.3.6. Current Status of Direct Sea-Level Reconstruction: The Past 500 ky | 4214 | ||
8.13.4. History and Current State of Sea-Level Determinations from Oxygen Isotope Measurements | 4219 | ||
8.13.4.1. History of Marine Oxygen Isotope Measurements and Sea Level | 4219 | ||
8.13.4.2. Comparison of Direct Sea-Level and Benthic Foram Records | 4219 | ||
8.13.4.3. 18O/16O-Based Sea-Level Records | 4219 | ||
8.13.5. Causes of Sea-Level Change and Future Work | 4220 | ||
References | 4221 | ||
Chapter 8.14: Elemental and Isotopic Proxies of Past Ocean Temperatures | 4224 | ||
8.14.1. Introduction | 4224 | ||
8.14.2. A Brief History of Early Research on Geochemical Proxies of Temperature | 4225 | ||
8.14.3. Oxygen Isotopes as a Paleotemperature Proxy in Foraminifera | 4226 | ||
8.14.3.1. Background | 4226 | ||
8.14.3.2. Paleotemperature Equations | 4227 | ||
8.14.3.3. Secondary Effects and Diagenesis | 4227 | ||
8.14.3.4. Results on Quaternary Timescales | 4228 | ||
8.14.3.5. Results for the Neogene, Paleogene, and Earlier Periods | 4228 | ||
8.14.3.6. Summary of Outstanding Research Issues | 4228 | ||
8.14.4. Oxygen Isotopes as a Climate Proxy in Reef Corals | 4229 | ||
8.14.4.1. Background | 4229 | ||
8.14.4.2. Paleotemperature Equations | 4229 | ||
8.14.4.3. Secondary Effects and Diagenesis | 4229 | ||
8.14.4.4. Results on Historical Timescales | 4230 | ||
8.14.4.5. Results on Late Quaternary Timescales | 4230 | ||
8.14.4.6. Summary of Outstanding Research Issues | 4231 | ||
8.14.5. Oxygen Isotopes as a Climate Proxy in other Marine Biogenic Phases | 4231 | ||
8.14.6. Clumped Oxygen Isotopes | 4231 | ||
8.14.7. Magnesium as a Paleotemperature Proxy in Foraminifera | 4231 | ||
8.14.7.1. Background and History | 4231 | ||
8.14.7.2. Calibration and Paleotemperature Equations | 4232 | ||
8.14.7.3. Effect of Dissolution | 4233 | ||
8.14.7.4. Other Secondary Effects on Mg/Ca | 4234 | ||
8.14.7.5. Results over the Last Few Millennia | 4235 | ||
8.14.7.6. Results on Quaternary Timescales | 4235 | ||
8.14.7.7. Results for the Neogene, Paleogene, and Beyond | 4237 | ||
8.14.7.8. Summary of Outstanding Research Issues | 4237 | ||
8.14.8. Magnesium as a Paleotemperature Proxy in Ostracoda | 4238 | ||
8.14.9. Strontium as a Climate Proxy in Corals | 4238 | ||
8.14.9.1. Background | 4238 | ||
8.14.9.2. Paleotemperature Equations | 4238 | ||
8.14.9.3. Secondary Effects and Diagenesis | 4239 | ||
8.14.9.4. Results on Historical Timescales | 4240 | ||
8.14.9.5. Results on Geological Timescales | 4240 | ||
8.14.9.6. Summary of Outstanding Research Issues | 4241 | ||
8.14.10. Magnesium and Uranium in Corals as Paleotemperature Proxies | 4241 | ||
8.14.11. Calcium Isotopes as a Paleotemperature Proxy | 4241 | ||
8.14.12. Conclusions | 4241 | ||
Acknowledgments | 4242 | ||
References | 4242 | ||
Chapter 8.15: Alkenone Paleotemperature Determinations | 4250 | ||
8.15.1. Introduction | 4250 | ||
8.15.2. Systematics and Detection | 4252 | ||
8.15.3. Occurrence of Alkenones in Marine Waters and Sediments | 4254 | ||
8.15.3.1. Genetic and Evolutionary Aspects of Alkenone Production | 4255 | ||
8.15.4. Function | 4256 | ||
8.15.5. Ecological Controls on Alkenone Production and Downward Flux | 4257 | ||
8.15.5.1. Effects of Water Column Recycling and Sediment Diagenesis on the Alkenone Unsaturation Index | 4260 | ||
8.15.6. Calibration of Uk37 Index to Temperature | 4262 | ||
8.15.6.1. Culture Calibrations | 4263 | ||
8.15.6.2. Particles | 4264 | ||
8.15.6.2.1. Sediment traps | 4265 | ||
8.15.6.3. Core Tops | 4265 | ||
8.15.7. Synthesis of Calibration | 4267 | ||
8.15.8. Paleotemperature Studies Using the Alkenone Method | 4268 | ||
8.15.8.1. Holocene High-Resolution Studies | 4268 | ||
8.15.8.2. Millennial-Scale Events of the Late Pleistocene and Last Glacial Termination | 4269 | ||
8.15.8.3. Marine Temperatures During the LGM | 4270 | ||
8.15.8.4. SST Records of the Late Pleistocene Ice Age Cycles | 4273 | ||
8.15.8.5. SST before the Late Pleistocene | 4274 | ||
8.15.8.6. Comparison with other Proxies: delta18O | 4274 | ||
8.15.8.7. Comparison with other Proxies: Microfossils | 4275 | ||
8.15.8.8. Comparison with other Proxies: Mg/Ca | 4276 | ||
8.15.8.9. Comparison with other Proxies: TEX86 and other Glycerol Dialkyl Glycerol Tetraethers Indice | 4277 | ||
8.15.8.10. Intercomparison of SST Proxies: Some Generalities | 4277 | ||
8.15.9. Conclusions | 4277 | ||
References | 4278 | ||
Chapter 8.16: Tracers of Past Ocean Circulation | 4286 | ||
8.16.1. Introduction | 4286 | ||
8.16.2. Nutrient Water Mass Tracers | 4287 | ||
8.16.2.1. Carbon Isotopes | 4287 | ||
8.16.2.1.1. Controls on delta13C of oceanic carbon | 4287 | ||
8.16.2.1.2. Carbon isotope ratios in benthic foraminifera | 4288 | ||
8.16.2.2. Cadmium in Benthic Foraminifera | 4289 | ||
8.16.2.2.1. Cd in seawater | 4289 | ||
8.16.2.2.2. Cd/Ca in foraminifera | 4290 | ||
8.16.2.3. Ba/Ca | 4291 | ||
8.16.2.4. Zn/Ca | 4291 | ||
8.16.2.5. Silicon Isotopes | 4291 | ||
8.16.3. Conservative Water Mass Tracers | 4291 | ||
8.16.3.1. Mg/Ca in Benthic Foraminifera | 4291 | ||
8.16.3.2. Pore Water Chemistry | 4291 | ||
8.16.3.3. Oxygen Isotopes in Benthic Foraminifera | 4292 | ||
8.16.3.4. Clumped Isotopes | 4292 | ||
8.16.3.5. Carbon Isotope Air-Sea Exchange Signature | 4292 | ||
8.16.4. Neodymium Isotope Ratios | 4293 | ||
8.16.5. Circulation Rate Tracers | 4293 | ||
8.16.5.1. Radiocarbon | 4293 | ||
8.16.5.2. 231Pa/230Th Ratio | 4294 | ||
8.16.5.3. Geostrophic Shear Estimates from delta18O in Benthic Foraminifera | 4294 | ||
8.16.6. Nongeochemical Tracers of Past Ocean Circulation | 4294 | ||
8.16.7. Ocean Circulation during the LGM | 4295 | ||
8.16.8. Conclusions | 4299 | ||
References | 4299 | ||
Chapter 8.17: Long-lived Isotopic Tracers in Oceanography, Paleoceanography, and Ice-sheet Dynamics | 4304 | ||
8.17.1. Introduction | 4304 | ||
8.17.2. Long-lived Isotopic Tracers and Their Applications | 4304 | ||
8.17.3. Systematics of Long-lived Isotope Systems in the Earth | 4305 | ||
8.17.3.1. Early Applications to the Oceans | 4307 | ||
8.17.4. Neodymuim Isotopes in the Oceans | 4308 | ||
8.17.4.1. REEs in Seawater | 4308 | ||
8.17.4.2. Neodymium-isotope Ratios in Seawater | 4309 | ||
8.17.4.3. Where does Seawater Neodymium Come From? | 4313 | ||
8.17.4.4. Neodymium Isotopes as Water-mass Tracers | 4314 | ||
8.17.4.5. The ``Nd Paradox´´ | 4316 | ||
8.17.4.6. Implications of Nd Isotopes and Concentrations in Seawater | 4319 | ||
8.17.5. Applications to Paleoclimate | 4320 | ||
8.17.5.1. Radiogenic Isotopes in Authigenic Ferromanganese Oxides | 4320 | ||
8.17.5.2. Long-term Time Series in Fe-Mn Crusts | 4322 | ||
8.17.5.3. Hf-Nd Isotope Trends in the Oceans | 4323 | ||
8.17.6. Long-lived Radiogenic Tracers and Ice-sheet Dynamics | 4324 | ||
8.17.6.1. Heinrich Events | 4325 | ||
8.17.6.2. K/Ar ages of Heinrich Event Detritus | 4325 | ||
8.17.6.3. Nd-Sr-Pb Isotopes in Terrigenous Sediments | 4325 | ||
8.17.6.4. Isotopic and Geochronologic Measurements on Individual Mineral Grains | 4327 | ||
8.17.6.5. Contrasting Provenance of H3 and H6 | 4327 | ||
8.17.6.6. Summary of Geochemical Provenance Analysis of Heinrich Layers | 4328 | ||
8.17.6.7. Trough Mouth Fans as Archives of Major IRD Sources | 4328 | ||
8.17.6.8. 40Ar/39Ar Hornblende Evidence for History of the Laurentide Ice Sheet During the Last Glacial Cycle | 4329 | ||
8.17.7. Final Thoughts | 4331 | ||
Acknowledgments | 4331 | ||
References | 4331 | ||
Chapter 8.18: The Biological Pump in the Past | 4336 | ||
8.18.1. Introduction | 4336 | ||
8.18.2. Concepts | 4340 | ||
8.18.2.1. Aqueous Carbon Chemistry | 4340 | ||
8.18.2.2. Soft-Tissue versus Carbonate Pump | 4341 | ||
8.18.2.3. Low- versus High-Latitude Ocean | 4343 | ||
8.18.2.4. Southern Ocean versus North Atlantic | 4346 | ||
8.18.3. Tools | 4348 | ||
8.18.3.1. Surface Ocean Biogeochemistry | 4348 | ||
8.18.3.1.1. Nutrient status | 4349 | ||
8.18.3.1.2. Export production | 4350 | ||
8.18.3.2. Ocean Ventilation | 4351 | ||
8.18.3.2.1. Water mass distribution | 4351 | ||
8.18.3.2.2. Rates of ocean overturning and ventilation | 4352 | ||
8.18.3.3. Integrative Constraints on the Biological Pump | 4353 | ||
8.18.3.3.1. Carbon isotope distribution of the ocean and atmosphere | 4353 | ||
8.18.3.3.2. Deep-ocean oxygen content | 4353 | ||
8.18.3.3.3. Carbon chemistry and boron | 4354 | ||
8.18.4. Observations | 4355 | ||
8.18.4.1. 800 000 Year Perspective | 4355 | ||
8.18.4.2. Deglacial Perspective | 4357 | ||
References | 4359 | ||
Chapter 8.19: The Oceanic CaCO3 Cycle | 4370 | ||
8.19.1. Introduction | 4370 | ||
8.19.2. The Contemporary Marine CaCO3 Cycle | 4370 | ||
8.19.2.1. Formation and Transport Pathways of CaCO3 | 4372 | ||
8.19.2.2. Destruction and Dissolution of CaCO3 | 4373 | ||
8.19.3. Oceanic Distribution and Present-Day Changes in the Seawater CO2-Carbonic Acid System Due to Human Activities | 4376 | ||
8.19.3.1. CO2 Gradients within and across Ocean Basins | 4376 | ||
8.19.3.2. Surface Uptake of Anthropogenic CO2 and Future Projections | 4377 | ||
8.19.3.3. Changes in Distribution of Omega and Shoaling of the CaCO3 Saturation Horizon | 4379 | ||
8.19.4. Implications of Anthropogenic Ocean Acidification to the Marine CaCO3 Cycle | 4379 | ||
8.19.4.1. Formation of CaCO3 | 4379 | ||
8.19.4.2. Destruction and Dissolution of CaCO3 | 4383 | ||
8.19.4.3. Feedbacks to Atmospheric CO2 and the Earth Climate System | 4384 | ||
8.19.5. A Brief Commentary on Past Alterations to the Marine CaCO3 Cycle and Analogies to the Present Perturbation | 4385 | ||
8.19.5.1. Calcite and Aragonite Seas | 4385 | ||
8.19.5.2. Glacial to Interglacial Periods | 4386 | ||
8.19.5.3. Paleocene Eocene Thermal Maximum | 4387 | ||
8.19.5.4. Volcanic Vent Systems | 4387 | ||
8.19.6. Back to the Future: Summary of Past and Present Clues on the Future CaCO3 Cycle | 4387 | ||
Acknowledgments | 4388 | ||
References | 4388 | ||
Chapter 8.20: Records of Cenozoic Ocean Chemistry | 4394 | ||
8.20.1. Introduction | 4394 | ||
8.20.2. Cenozoic Deep-Sea Stable Isotope Record | 4395 | ||
8.20.2.1. Oxygen Isotopes and Climate | 4395 | ||
8.20.2.2. Carbon Isotopes and Ocean Carbon Chemistry | 4398 | ||
8.20.3. The Marine Strontium and Osmium Isotope Records | 4399 | ||
8.20.3.1. Globally Integrated Records of Inputs to the Ocean | 4399 | ||
8.20.3.2. Osmium-Strontium Decoupling | 4400 | ||
8.20.3.2.1. Decoupled riverine fluxes of strontium and osmium? | 4401 | ||
8.20.3.2.2. Decoupled unradiogenic fluxes? | 4401 | ||
8.20.3.3. Reconstructing Seawater Isotope Composition from Sediments | 4401 | ||
8.20.3.4. Cenozoic Strontium and Osmium Isotope Records | 4402 | ||
8.20.3.4.1. Overview of the Cenozoic marine strontium isotope record | 4402 | ||
8.20.3.4.2. Overview of the Cenozoic marine osmium isotope record | 4403 | ||
8.20.3.4.3. Significance of uplift and weathering of the Himalayan-Tibetan Plateau (HTP) | 4404 | ||
8.20.3.4.4. Glaciation and the marine strontium and osmium isotope records | 4405 | ||
8.20.3.5. Variations in the Strontium and Osmium Isotope Composition of Riverine Input | 4406 | ||
8.20.3.6. Osmium and Strontium Isotopes as Chemical Weathering Proxies | 4407 | ||
8.20.4. Mg/Ca Records from Benthic Foraminifera | 4408 | ||
8.20.4.1. Coupling Benthic Foraminiferal Mg/Ca and Oxygen Isotope Records | 4408 | ||
8.20.4.2. Calibration of the Mg/Ca Thermometer | 4408 | ||
8.20.4.3. Cenozoic Benthic Foraminiferal Mg/Ca Records | 4410 | ||
8.20.4.4. Changing Seawater Mg/Ca Ratio | 4411 | ||
8.20.5. Boron Isotopes, Paleo-pH, and Atmospheric CO2 | 4411 | ||
8.20.5.1. The pH Dependence of Boron Isotope Fractionation | 4411 | ||
8.20.5.2. Boron Partitioning into Calcite | 4412 | ||
8.20.5.3. Paleo-pH and Atmospheric CO2 Reconstruction | 4413 | ||
8.20.5.4. Outstanding Questions about Paleo-pH Reconstructions | 4414 | ||
8.20.6. Closing Synthesis: Does Orogenesis Lead to Cooling? | 4415 | ||
References | 4416 | ||
Chapter 8.21: The Geologic History of Seawater | 4420 | ||
8.21.1. Introduction | 4420 | ||
8.21.2. The Hadean (4.5-4.0 Ga) | 4421 | ||
8.21.3. The Archean (4.0-2.5 Ga) | 4422 | ||
8.21.3.1. The Paleoarchean (4.0-3.7 Ga) | 4422 | ||
8.21.3.2. The Mesoarchean (3.7-3.0 Ga) | 4423 | ||
8.21.3.3. The Neoarchean (3.0-2.5 Ga) | 4424 | ||
8.21.4. The Proterozoic (2.5-0.542 Ga) | 4427 | ||
8.21.4.1. The Early and Middle Paleoproterozoic (2.5-1.8 Ga) | 4428 | ||
8.21.4.2. The Late Paleoproterozoic and Mesoproterozoic (1.8-1.0 Ga) | 4430 | ||
8.21.4.3. The Early and Middle Neoproterozoic (1.0-0.635 Ga) | 4434 | ||
8.21.4.4. The Ediacaran Period (0.635-0.542 Ga) | 4439 | ||
8.21.5. The Phanerozoic (0.542 Ga-Present) | 4442 | ||
8.21.5.1. Evidence from Marine Evaporites | 4442 | ||
8.21.5.2. Fluid Inclusions in Marine Halites | 4443 | ||
8.21.5.3. The Analysis of Unevaporated Seawater in Fluid Inclusions | 4446 | ||
8.21.5.4. Br- Concentration in Halite | 4446 | ||
8.21.5.5. The Mineralogy of Marine Oölites and Carbonate Cements | 4447 | ||
8.21.5.6. Mg2+/Ca2+ and Sr2+/Ca2+ Ratios of Marine Shell-Building Organisms (Echinoderms, Rudists, and Foraminifera) | 4448 | ||
8.21.5.7. Seawater Mg2+/Ca2+ Ratio and the Carbonate Mineralogy of Shell-Building Organisms | 4449 | ||
8.21.5.8. Seawater Mg2+/Ca2+ Ratio and Shell-Building Organisms: Experimental Results | 4450 | ||
8.21.5.9. Models of Phanerozoic Seawater Chemistry | 4451 | ||
8.21.5.10. The Isotopic Composition of Strontium in Marine Carbonates | 4454 | ||
8.21.5.11. The Isotopic Composition of Sulfur in Seawater | 4456 | ||
8.21.5.12. The Isotopic Composition of Calcium in Seawater | 4460 | ||
8.21.6. Summary | 4460 | ||
Acknowledgments | 4462 | ||
References | 4462 | ||
e9780080983004v9 | 4474 | ||
Front Cover | 4474 | ||
Sediments, Diagenesis, and Sedimentary Rocks | 4477 | ||
Copyright | 4478 | ||
In Memoriam | 4479 | ||
Heinrich Dieter Holland (1927–2012) | 4481 | ||
Karl Karekin Turekian (1927–2013) | 4483 | ||
References | 4485 | ||
Dedication | 4487 | ||
Contents | 4489 | ||
Executive Editors’ Foreword to the Second Edition | 4491 | ||
Contributors | 4495 | ||
Volume Editors' Introduction | 4497 | ||
References | 4505 | ||
Chapter 9.1: Chemical Composition and Mineralogy of Marine Sediments | 4507 | ||
9.1.1. Introduction | 4507 | ||
9.1.2. Pelagic Sediments | 4510 | ||
9.1.2.1. Equatorial Pacific | 4512 | ||
9.1.2.2. South Pacific | 4513 | ||
9.1.2.3. Central Indian Basin | 4515 | ||
9.1.3. Ferromanganese Nodules and Crusts | 4517 | ||
9.1.3.1. Equatorial Pacific Nodules | 4520 | ||
9.1.3.2. Seamount Ferromanganese Crusts from the Central Pacific | 4522 | ||
9.1.4. Metalliferous Ridge and Basal Sediments | 4524 | ||
9.1.4.1. Metalliferous Ridge Sediments | 4527 | ||
9.1.4.2. Metalliferous Basal Sediments | 4528 | ||
9.1.5. Marine Phosphorites | 4528 | ||
9.1.6. Conclusions | 4532 | ||
References | 4535 | ||
Chapter 9.2: The Recycling of Biogenic Material at the Sea Floor | 4539 | ||
9.2.1. Introduction | 4539 | ||
9.2.2. Pore Water Sampling and Profiling | 4540 | ||
9.2.3. Organic Matter Decomposition in Sediments | 4542 | ||
9.2.3.1. Electron Acceptors for Sedimentary Organic Matter Oxidation | 4542 | ||
9.2.3.2. The Stoichiometry of Sedimentary Organic Matter Decomposition | 4547 | ||
9.2.3.2.1. Pelagic sediments | 4548 | ||
9.2.3.2.2. Continental margin sediments | 4549 | ||
9.2.3.3. The Depth Distribution of Organic Matter Oxidation in the Sediment Column | 4550 | ||
9.2.3.4. The Response Time for Organic Matter Oxidation | 4552 | ||
9.2.3.5. The Burial of Organic Matter in Marine Sediments | 4553 | ||
9.2.3.6. Benthic Organic Matter Cycling and Marine Geochemical Cycles | 4554 | ||
9.2.4. Particle Mixing in Surface Sediments: Bioturbation | 4554 | ||
9.2.5. CaCO3 Dissolution in Sediments | 4556 | ||
9.2.5.1. Metabolic Dissolution in Sediments Above the Calcite Lysocline | 4556 | ||
9.2.5.2. Metabolic Dissolution and the Kinetics of Organic Matter Oxidation | 4558 | ||
9.2.5.3. CaCO3 Dissolution in Sediments on the Continental Slope and Rise | 4559 | ||
9.2.5.4. Dissolution in Carbonate-Rich Sediments on the Continental Shelf | 4559 | ||
9.2.6. Silica Cycling in Sediments | 4559 | ||
9.2.6.1. Dissolved Silica Profiles in Pore Waters | 4559 | ||
9.2.6.2. Preservation Efficiency | 4560 | ||
9.2.6.3. Summary: Silica in Pore Waters and Sediments | 4562 | ||
9.2.7. Conclusions | 4562 | ||
References | 4563 | ||
Chapter 9.3: Formation and Diagenesis of Carbonate Sediments | 4567 | ||
9.3.1. Introduction | 4568 | ||
9.3.2. Physical Geochemistry of Carbonate Minerals | 4569 | ||
9.3.2.1. Preliminary Remarks | 4569 | ||
9.3.2.2. Carbonic Acid System and Basic Solution Equilibria | 4569 | ||
9.3.2.3. Major Marine Carbonate Phases | 4571 | ||
9.3.2.3.1. Magnesian calcite | 4572 | ||
9.3.2.3.2. Dolomite and related phases | 4574 | ||
9.3.2.3.3. Aragonite and other related carbonates | 4575 | ||
9.3.3. Surface Reactions: Review of Theory | 4575 | ||
9.3.3.1. Preliminary Remarks | 4575 | ||
9.3.3.2. General Rate Equations | 4576 | ||
9.3.3.2.1. Crystal growth | 4578 | ||
9.3.3.2.2. Crystal dissolution | 4580 | ||
9.3.3.2.3. Reaction rate and cation/anion ratios | 4582 | ||
9.3.4. New Directions, New Insights | 4583 | ||
9.3.4.1. Preliminary Remarks | 4583 | ||
9.3.4.2. Structure of the Cleavage Surface | 4583 | ||
9.3.4.3. Detail Rate Measurements by Surface Microscopy | 4585 | ||
9.3.4.3.1. Atomic force microscopy | 4585 | ||
9.3.4.3.2. Vertical scanning interferometry | 4585 | ||
9.3.4.4. Growth Measurements | 4586 | ||
9.3.4.4.1. Saturation state | 4586 | ||
9.3.4.4.2. Impurities and growth inhibition | 4587 | ||
9.3.4.4.3. Beyond BCF | 4588 | ||
9.3.4.5. Dissolution Measurements | 4588 | ||
9.3.4.5.1. Baseline rates | 4589 | ||
9.3.4.5.2. Etch-pit morphology and significance | 4589 | ||
9.3.4.5.3. Dissolution inhibitors | 4589 | ||
9.3.4.5.4. Magnesite and dolomite dissolution | 4590 | ||
9.3.4.6. Rate Control by `Indifferent´ Electrolytes | 4590 | ||
9.3.4.7. Rate Control by Dissolved Ratio of Lattice Ions | 4591 | ||
9.3.4.8. Concluding Remarks | 4592 | ||
9.3.5. Sources and Diagenesis of Deep-Sea Carbonates | 4592 | ||
9.3.5.1. Sources and Sedimentation | 4592 | ||
9.3.5.2. Distribution of CaCO3 in Deep-Sea Sediments | 4593 | ||
9.3.5.3. CaCO3 Diagenesis in Deep-Sea Sediments | 4594 | ||
9.3.5.3.1. General relations | 4594 | ||
9.3.5.3.2. Early diagenetic processes in deep-sea sediments | 4594 | ||
9.3.6. Sources and Diagenesis of Shoal-Water Carbonate-Rich Sediments | 4596 | ||
9.3.6.1. Sources of Shoal-Water Carbonates | 4596 | ||
9.3.6.1.1. General considerations | 4596 | ||
9.3.6.1.2. Sources of carbonate muds | 4596 | ||
9.3.6.1.3. Formation of carbonate sands | 4597 | ||
9.3.6.2. Early Marine Diagenesis of Shoal-Water Carbonate-Rich Sediments | 4597 | ||
9.3.6.2.1. Pore-water chemistry | 4597 | ||
9.3.6.2.2. Precipitation `of early´ carbonate cements | 4598 | ||
9.3.6.2.3. Dissolution of carbonates | 4600 | ||
9.3.6.2.4. Carbonate diagenesis associated with reefs | 4600 | ||
9.3.6.2.5. Early dolomite formation | 4601 | ||
References | 4601 | ||
Chapter 9.4: The Diagenesis of Biogenic Silica: Chemical Transformations Occurring in the Water Column, Seabed, and Crust | 4609 | ||
9.4.1. Introduction | 4609 | ||
9.4.2. The Precipitation of Biogenic Silica | 4610 | ||
9.4.3. The Physical Properties of Biogenic Silica | 4610 | ||
9.4.4. Changes in Biogenic Silica Chemistry Occurring in the Water Column | 4611 | ||
9.4.5. Diagenesis of Biogenic Silica in the Upper Meter of the Seabed | 4612 | ||
9.4.5.1. Modeling Biogenic Silica Distributions and Pore-Water Silicate Concentrations | 4612 | ||
9.4.5.2. Transformation of Biogenic Silica to Authigenic Clay Minerals | 4613 | ||
9.4.5.3. Preservation of Biogenic Silica in the Seabed | 4613 | ||
9.4.6. Silica Diagenesis on Timescales of Millions of Years | 4614 | ||
9.4.6.1. Formation of Opal-CT and Chert | 4614 | ||
9.4.6.2. Geological Settings for Chert Formation | 4616 | ||
Acknowledgments | 4616 | ||
References | 4616 | ||
Chapter 9.5: Formation and Geochemistry of Precambrian Cherts | 4619 | ||
9.5.1. Introduction | 4619 | ||
9.5.1.1. Definition and Importance | 4620 | ||
9.5.1.2. Fundamental Difference Between Precambrian and Phanerozoic Chert | 4621 | ||
9.5.1.3. Aspects of Inorganic Geochemistry of Silica | 4622 | ||
9.5.1.4. Possible Association of Chert and Evaporite | 4623 | ||
9.5.2. Neoproterozoic and Mesoproterozoic Environments of Chert Formation | 4623 | ||
9.5.3. Chert of Late Archean and Paleoproterozoic Iron Formation | 4624 | ||
9.5.3.1. Chert of Lake Superior-Type Iron Formation | 4626 | ||
9.5.3.2. The End of Major Cherty Iron Formation Deposition | 4627 | ||
9.5.3.3. Chert of the Transvaal Supergroup and Hamersley Group | 4627 | ||
9.5.4. Archean Chert and Cherty Iron Formation | 4628 | ||
9.5.5. Stable Isotopes and Rare Earth Elements in Precambrian Chert and Cherty Iron Formation | 4629 | ||
9.5.5.1. Silicon Isotope Systematics | 4629 | ||
9.5.5.2. Raleigh-Type Fractionation of Silicon Isotopes | 4630 | ||
9.5.5.3. Silicon Isotopic Studies in Early Archean Chert and Temporal Changes in δ30Si | 4631 | ||
9.5.5.4. Oxygen Isotope Systematics | 4634 | ||
9.5.5.5. Preservation of the Oxygen Isotope Record in Precambrian Chert | 4635 | ||
9.5.5.6. Oxygen Isotope Composition of Seawater | 4636 | ||
9.5.5.7. Hydrogen Isotopes in Precambrian Chert | 4637 | ||
9.5.5.8. Iron Isotope Systematics | 4638 | ||
9.5.5.9. Iron and Silicon Isotope Studies of Early Paleoproterozoic Iron Formation | 4638 | ||
9.5.5.10. Rare Earth Elements as Indicators of Depositional Environments of Cherts and Cherty Iron Formation | 4639 | ||
9.5.6. Conclusions | 4640 | ||
Acknowledgments | 4641 | ||
References | 4641 | ||
Chapter 9.6: Geochemistry of Fine-Grained, Organic Carbon-Rich Facies | 4647 | ||
9.6.1. Introduction | 4647 | ||
9.6.2. Conceptual Model: Processes | 4648 | ||
9.6.2.1. Detrital Flux | 4649 | ||
9.6.2.2. Biogenic Flux | 4650 | ||
9.6.2.3. Authigenic Flux | 4651 | ||
9.6.2.4. Carbon Cycle and Climate Feedback | 4652 | ||
9.6.3. Conceptual Model: Proxies | 4653 | ||
9.6.3.1. Limitations of Proxy Data | 4653 | ||
9.6.3.2. Detrital Proxies | 4654 | ||
9.6.3.2.1. Physical methods | 4654 | ||
9.6.3.2.2. Elemental proxies | 4655 | ||
9.6.3.3. Biogenic Proxies | 4655 | ||
9.6.3.3.1. Organic matter sources | 4655 | ||
9.6.3.3.2. Stable carbon isotopes of organic matter (delta13C) | 4656 | ||
9.6.3.3.3. Elemental ratios | 4656 | ||
9.6.3.3.4. Biomarkers | 4656 | ||
9.6.3.3.5. Accumulation rates | 4657 | ||
9.6.3.4. Authigenic Proxies | 4657 | ||
9.6.3.4.1. C-S relationships | 4657 | ||
9.6.3.4.2. Sulfur isotope relationships | 4658 | ||
9.6.3.4.3. Iron | 4660 | ||
9.6.3.4.4. Trace metals | 4661 | ||
9.6.4. Geochemical Case Studies of Fine-Grained, Organic Carbon-Rich Sediments and Sedimentary Rocks | 4663 | ||
9.6.4.1. Modern Anoxic Environments of OM Burial: The Black Sea and Cariaco Basin | 4663 | ||
9.6.4.2. Cretaceous Western Interior Basin | 4665 | ||
9.6.4.3. Devonian Appalachian Basin | 4671 | ||
9.6.4.4. Recent Precambrian Advances | 4674 | ||
9.6.5. Discussion: A Unified View of the Geochemistry of Fine-Grained Organic Carbon-Rich Sediments and Sedim | 4674 | ||
9.6.5.1. Applicability of the Model | 4675 | ||
9.6.5.2. Detrital Fluxes and Bulk Sedimentation | 4675 | ||
9.6.5.3. Productivity and Nutrients | 4675 | ||
9.6.5.4. Productivity and C Isotope Records | 4675 | ||
9.6.5.5. Mo, Fe, and Redox Control | 4675 | ||
9.6.5.6. Elemental Ratios versus Accumulation Rates | 4676 | ||
9.6.5.7. Future Prospects | 4676 | ||
Acknowledgments | 4676 | ||
References | 4676 | ||
Chapter 9.7: Late Diagenesis and Mass Transfer in Sandstone-Shale Sequences | 4687 | ||
9.7.1. Introduction | 4687 | ||
9.7.2. The Realm of `Late Diagenesis´ | 4688 | ||
9.7.3. Elemental Mobility at the Grain Scale | 4688 | ||
9.7.3.1. Physical Processes | 4688 | ||
9.7.3.1.1. Compaction | 4688 | ||
9.7.3.1.2. Fracturing | 4689 | ||
9.7.3.2. Chemical Processes in Late Diagenesis | 4689 | ||
9.7.3.2.1. Dissolution | 4689 | ||
9.7.3.2.2. Cementation | 4691 | ||
9.7.3.2.3. Replacement | 4691 | ||
9.7.4. Volumetrically Significant Processes of Late Diagenesis | 4692 | ||
9.7.4.1. Detrital Feldspar Dissolution and Replacement | 4692 | ||
9.7.4.2. Dissolution of Detrital Quartz (Pressure Solution) | 4696 | ||
9.7.4.3. Dissolution of Detrital Carbonate | 4697 | ||
9.7.4.4. Illitization | 4697 | ||
9.7.4.5. Quartz Cement | 4698 | ||
9.7.4.6. Authigenic Calcite | 4699 | ||
9.7.4.7. Kaolinite and Chlorite | 4701 | ||
9.7.4.8. Minor Cement and Replacement Phases | 4701 | ||
9.7.4.9. Summary: Massive Reorganization by Fluid-Mediated Reactions | 4702 | ||
9.7.5. Whole-Rock Elemental Data and Larger-Scale Elemental Mobility | 4703 | ||
9.7.5.1. SiO2 | 4703 | ||
9.7.5.2. CaO (and CO2) | 4704 | ||
9.7.5.3. K2O | 4704 | ||
9.7.6. Fluid Flow | 4704 | ||
9.7.7. Reverse Weathering and Concluding Comments | 4704 | ||
Acknowledgments | 4705 | ||
References | 4705 | ||
Chapter 9.8: Coal Formation and Geochemistry | 4713 | ||
9.8.1. Introduction | 4713 | ||
9.8.2. Coal Formation | 4714 | ||
9.8.2.1. Peat Formation and Early Diagenesis | 4714 | ||
9.8.2.2. Coalification, Early Stage (Lignite, Brown Coal) | 4719 | ||
9.8.2.3. Coalification, Late Stage (Subbituminous, Bituminous, Anthracite) | 4721 | ||
9.8.3. Coal Rank | 4721 | ||
9.8.4. Structure of Coal | 4722 | ||
9.8.5. Hydrocarbons from Coal | 4723 | ||
9.8.5.1. Liquid Hydrocarbons (Oil) | 4723 | ||
9.8.5.2. Natural Gas | 4724 | ||
9.8.6. Inorganic Geochemistry of Coal | 4726 | ||
9.8.6.1. Abundance of Elements | 4726 | ||
9.8.6.2. Mineralogy | 4728 | ||
9.8.6.3. Modes of Occurrence | 4729 | ||
9.8.7. Geochemistry of Coal Utilization | 4731 | ||
9.8.8. Economic Potential of Metals from Coal | 4731 | ||
9.8.9. Inorganics in Coal as Indicators of Depositional Environments | 4731 | ||
9.8.10. Environmental Impacts | 4732 | ||
9.8.10.1. Coal Combustion | 4732 | ||
9.8.10.1.1. Sulfur emissions | 4732 | ||
9.8.10.1.2. Nitrogen emissions | 4732 | ||
9.8.10.1.3. Carbon dioxide emissions | 4733 | ||
9.8.10.1.4. Emission of particulates | 4733 | ||
9.8.10.1.5. Emission of trace elements | 4733 | ||
9.8.10.1.6. Emission of organic compounds | 4733 | ||
9.8.10.2. Environmental Impacts of Mining and Disposal of Coal Wastes | 4733 | ||
9.8.10.3. Disposal of Solid Wastes from Coal Utilization | 4733 | ||
9.8.10.4. Human Health | 4733 | ||
9.8.11. Conclusions | 4734 | ||
References | 4735 | ||
Chapter 9.9: Formation and Geochemistry of Oil and Gas | 4739 | ||
9.9.1. Introduction | 4739 | ||
9.9.2. The Early Steps in Oil and Gas Formation: Where Does It All Begin? | 4739 | ||
9.9.3. Insoluble Organic Material - Kerogen | 4741 | ||
9.9.4. Soluble Organic Material | 4744 | ||
9.9.4.1. Source | 4746 | ||
9.9.4.2. Depositional Environments | 4747 | ||
9.9.4.3. Maturity | 4750 | ||
9.9.4.4. Biodegradation | 4753 | ||
9.9.4.5. Age Dating | 4754 | ||
9.9.4.6. Migration | 4754 | ||
9.9.5. Geochemistry and Sequence Stratigraphy | 4755 | ||
9.9.5.1. Lowstand System Tract | 4755 | ||
9.9.5.2. Transgressive System Tract | 4755 | ||
9.9.5.3. Condensed Section | 4755 | ||
9.9.5.4. Highstand System Tract | 4756 | ||
9.9.6. Fluid Inclusions | 4757 | ||
9.9.7. Reservoir Geochemistry | 4757 | ||
9.9.8. Basin Modeling | 4759 | ||
9.9.9. Natural Gas | 4763 | ||
9.9.10. Surface Prospecting | 4765 | ||
9.9.11. Summary | 4766 | ||
References | 4766 | ||
Chapter 9.10: The Sedimentary Sulfur System: Biogeochemistry and Evolution through Geologic Time | 4773 | ||
9.10.1. Introduction | 4774 | ||
9.10.2. Sulfur in Sediments | 4775 | ||
9.10.2.1. Forms of Sulfur in Sediments and Sedimentary Rocks | 4776 | ||
9.10.2.1.1. Dissolved reduced sulfur species in sedimentary sulfide systems | 4777 | ||
9.10.2.1.2. Iron sulfide minerals in sedimentary sulfide systems | 4778 | ||
9.10.2.1.2.1. Iron(II) monosulfide: mackinawite | 4778 | ||
9.10.2.1.2.2. Iron thiospinel, greigite | 4779 | ||
9.10.2.1.2.3. Pyrite, FeS2p | 4780 | ||
9.10.2.1.2.4. Cubic FeSc | 4780 | ||
9.10.2.1.2.5. Troilite (FeSt) and the pyrrhotite group | 4781 | ||
9.10.2.1.2.6. Smythite, rhombohedral Fe1-xSs | 4781 | ||
9.10.2.1.2.7. Marcasite, orthorhombic FeS2m | 4781 | ||
9.10.2.2. Distribution of Iron Sulfides in Sediments | 4782 | ||
9.10.2.2.1. Observations of iron sulfide minerals in sediments | 4782 | ||
9.10.2.2.2. Distribution of iron sulfide minerals in sediments | 4783 | ||
9.10.3. Pyrite Formation in Sediments | 4783 | ||
9.10.3.1. Thermodynamics of Sedimentary Pyrite Formation | 4783 | ||
9.10.3.2. Mechanisms of Pyrite Formation | 4785 | ||
9.10.3.3. Kinetics of Sedimentary Pyrite Formation | 4786 | ||
9.10.4. Other Forms of Sulfur in Sediments | 4787 | ||
9.10.4.1. Distribution of S8 in Sediments | 4787 | ||
9.10.4.2. Sulfur in Suboxic Sedimentary Systems | 4788 | ||
9.10.5. Reactive Iron | 4789 | ||
9.10.5.1. The Nature of Nonsulfide Fe in Sediments | 4789 | ||
9.10.5.2. Degree of Pyritization | 4791 | ||
9.10.5.3. The Reactive Iron Shuttle | 4791 | ||
9.10.5.4. Iron Isotopic Fractionation in Sulfidic Sediments | 4792 | ||
9.10.5.5. Secular Variations in the Iron Isotopic Composition of Sedimentary Pyrite | 4793 | ||
9.10.6. Microbial Ecology | 4793 | ||
9.10.6.1. Microbial Sulfur Transformations | 4793 | ||
9.10.6.2. Microbial Sulfate Reduction | 4794 | ||
9.10.6.3. Microbiological Sulfate Reduction Rates (SRR) | 4796 | ||
9.10.6.4. Iron Sulfides in Microorganisms | 4796 | ||
9.10.6.5. Molecular Phylogeny of Sulfur Microorganisms in Sediments | 4797 | ||
9.10.6.6. Syntrophy in the Sedimentary Sulfur System | 4797 | ||
9.10.6.7. Microbial Sulfide Oxidation in Sediments | 4798 | ||
9.10.6.8. Sulfur Disproportionating Organisms | 4799 | ||
9.10.6.9. Microbial Stratigraphy: Biofilms and Microbial Mats | 4799 | ||
9.10.6.10. Global Distribution of Sulfate-Reducing Microorganisms | 4800 | ||
9.10.7. Evolution of the Sulfur Biome | 4802 | ||
9.10.7.1. Sulfur Isotopic Fractionation by Microbial Sulfate Reduction | 4802 | ||
9.10.7.2. Oxygen Isotope Fractionation During Sulfate Reduction and Sulfide Oxidation | 4803 | ||
9.10.7.3. Microbial Disproportionation of Intermediate Sulfur Compounds | 4803 | ||
9.10.7.4. Evolution of the Sulfur Biome from Sedimentary Sulfur Stable Isotope Geochemistry | 4803 | ||
9.10.7.5. Carbon Isotopes | 4804 | ||
9.10.7.6. Molecular Fossils: Sedimentary Biomarkers for Ancient Sulfur Microbes | 4805 | ||
9.10.7.7. Molecular Biological Probes of the Evolution of the Sulfur Biome | 4805 | ||
9.10.8. Euxinic Systems | 4806 | ||
9.10.8.1. Oceanic Euxinic Conditions | 4806 | ||
9.10.8.2. Sulfide Distribution in Natural Waters | 4807 | ||
9.10.8.3. Proxies for Euxinia | 4807 | ||
9.10.8.3.1. Sediment S/Corg ratios | 4807 | ||
9.10.8.3.2. Biogenic silica | 4808 | ||
9.10.8.3.3. Degree of pyritization | 4808 | ||
9.10.8.3.4. Degree of sulfidation | 4808 | ||
9.10.8.3.5. Reactive Fe content (FeHR) | 4808 | ||
9.10.8.3.6. Pyrite framboid size | 4809 | ||
9.10.8.3.7. Organic carbon:phosphate ratios, Corg/P | 4809 | ||
9.10.8.3.8. Trace elements | 4809 | ||
9.10.8.4. Sulfur Stable Isotopes in Euxinic Sediments | 4809 | ||
9.10.8.5. Molybdenum Isotopes | 4810 | ||
9.10.8.6. Other Stable Isotopes | 4810 | ||
9.10.8.7. Biomarker Proxies | 4811 | ||
9.10.9. The Geochemistry of Sulfidic Sedimentary Rocks | 4811 | ||
9.10.9.1. Black Shales over the Last 200 Ma | 4812 | ||
9.10.9.2. Paleozoic Black Shales | 4813 | ||
9.10.9.3. Distribution of Black Shales in the Proterozoic | 4814 | ||
9.10.9.4. Archean Sulfidic Sediments | 4816 | ||
9.10.10. Geochemical Evolution of Sulfur-Based Sediments | 4817 | ||
9.10.10.1. Mass-Independent Fractionation (MIF) of Sulfur Isotopes | 4817 | ||
9.10.10.2. Sulfur-Rich Sediments and the History of Oceanic Oxygen | 4818 | ||
9.10.10.3. The History of Marine Sulfate Concentration | 4819 | ||
9.10.10.4. Variations in the Geochemistry of Sulfur-Rich Sediments through Geologic Time | 4820 | ||
Acknowledgments | 4821 | ||
References | 4821 | ||
Chapter 9.11: Manganiferous Sediments, Rocks, and Ores | 4833 | ||
9.11.1. Chemical Fundamentals | 4833 | ||
9.11.2. Distribution of Manganese in Rocks and Natural Waters | 4837 | ||
9.11.3. Common Manganese Minerals | 4838 | ||
9.11.4. Composition of Manganese Accumulations | 4840 | ||
9.11.5. Behavior of Manganese in Igneous Settings, Especially Mid-Ocean Ridge Vents | 4843 | ||
9.11.6. Behavior of Manganese in Sedimentation | 4845 | ||
9.11.6.1. Manganese Nodules and Crusts in Modern Sediments | 4845 | ||
9.11.6.2. Manganese Carbonates in Modern Sediments | 4845 | ||
9.11.7. Two Models of Sedimentary Manganese Mineralization | 4846 | ||
9.11.8. Behavior in Soils and Weathering | 4848 | ||
9.11.8.1. Manganese in Uncontaminated Soils | 4848 | ||
9.11.8.2. Manganese in Soils Affected by Mining and Smelting | 4850 | ||
9.11.9. Manganese through Geologic Time | 4850 | ||
9.11.10. Conclusions | 4851 | ||
References | 4851 | ||
Chapter 9.12: Green Clay Minerals | 4857 | ||
9.12.1. What Are We Looking At? | 4857 | ||
9.12.1.1. The Cycle of Transformation | 4858 | ||
9.12.1.2. Geologic Conditions of Green Clay Stability | 4858 | ||
9.12.2. Description of Green Clay Minerals | 4859 | ||
9.12.2.1. Potassic Green Clays (Mica-Based Structures) | 4859 | ||
9.12.2.1.1. Glauconites | 4859 | ||
9.12.2.1.2. Celadonite | 4861 | ||
9.12.2.1.3. Summary of potassic green clays | 4863 | ||
9.12.2.2. Ferrous, Green Clays | 4863 | ||
9.12.2.2.1. Occurrence of berthiérine and verdine minerals | 4863 | ||
9.12.2.2.2. Chamosite | 4866 | ||
9.12.3. Nonchlorite, Nonmicaceous Green Clay Minerals | 4866 | ||
9.12.3.1. Nontronite | 4866 | ||
9.12.3.2. Talc | 4867 | ||
9.12.4. Geochemical Origin of Green Clays | 4867 | ||
9.12.4.1. Shallow Ocean Bottom, Marine Green Clays - Glauconite and Berthiérine | 4867 | ||
9.12.4.2. Alteration Environments | 4868 | ||
9.12.4.3. Diagenesis Reactions | 4868 | ||
9.12.5. General Reflections | 4869 | ||
References | 4869 | ||
Chapter 9.13: Chronometry of Sediments and Sedimentary Rocks | 4871 | ||
9.13.1. Introduction | 4871 | ||
9.13.2. Chronometry Based on the Fossil Record - First Steps | 4871 | ||
9.13.3. Refinements in Chronometry Using Fossils | 4875 | ||
9.13.4. Oil Recovery in California Using Fossil-Based Chronometry | 4877 | ||
9.13.5. Principles of Chorology: The Science of the Distribution of Organisms | 4878 | ||
9.13.6. Constraints on Chronometry Imposed by Chorology | 4882 | ||
9.13.7. Radiochronometry | 4885 | ||
9.13.8. Magnetic Field Polarity and Chronometry | 4886 | ||
9.13.9. Orbital Chronometry | 4887 | ||
9.13.9.1. Aurichorology - The Golden Spikes and Global Statotype Section and Points | 4888 | ||
9.13.10. Terminologies | 4889 | ||
9.13.11. Summary | 4889 | ||
References | 4890 | ||
Chapter 9.14: The Geochemistry of Mass Extinction | 4891 | ||
9.14.1. Introduction | 4891 | ||
9.14.2. Isotope Records of the Major Mass Extinctions | 4892 | ||
9.14.2.1. Carbon Isotope Record | 4892 | ||
9.14.2.2. Sulfur Isotope Record | 4892 | ||
9.14.2.3. Strontium Isotope Record | 4893 | ||
9.14.2.4. Oxygen Isotope Record | 4894 | ||
9.14.3. Interpreting the Geochemical Records of Mass Extinction | 4894 | ||
9.14.3.1. Late Ordovician | 4894 | ||
9.14.3.2. Late Devonian | 4897 | ||
9.14.3.3. Permian-Triassic | 4897 | ||
9.14.3.4. Triassic-Jurassic | 4898 | ||
9.14.3.5. Cretaceous-Tertiary | 4898 | ||
9.14.4. Summary with Extensions | 4899 | ||
9.14.4.1. Paleozoic Suffocation | 4899 | ||
9.14.4.2. Mesozoic Menace | 4900 | ||
Acknowledgments | 4901 | ||
References | 4901 | ||
Chapter 9.15: Evolution of Sedimentary Rocks | 4905 | ||
9.15.1. Introduction | 4905 | ||
9.15.2. The Earth System | 4906 | ||
9.15.2.1. Population Dynamics | 4906 | ||
9.15.3. Generation and Recycling of the Oceanic and Continental Crust | 4907 | ||
9.15.4. Global Tectonic Realms and Their Recycling Rates | 4908 | ||
9.15.5. Present-Day Sedimentary Shell | 4909 | ||
9.15.6. Tectonic Settings and Their Sedimentary Packages | 4910 | ||
9.15.7. Petrology, Mineralogy, and Major Element Composition of Clastic Sediments | 4911 | ||
9.15.7.1. Provenance | 4911 | ||
9.15.7.2. Transport Sorting | 4912 | ||
9.15.7.3. Sedimentary Recycling | 4913 | ||
9.15.8. Trace Element and Isotopic Composition of Clastic Sediments | 4913 | ||
9.15.9. Secular Evolution of Clastic Sediments | 4914 | ||
9.15.9.1. Tectonic Settings and Lithology | 4914 | ||
9.15.9.2. Chemistry | 4914 | ||
9.15.9.3. Isotopes | 4916 | ||
9.15.10. Sedimentary Recycling | 4917 | ||
9.15.11. Ocean/Atmosphere System | 4917 | ||
9.15.11.1. The Chemical Composition of Ancient Ocean | 4918 | ||
9.15.11.2. Isotopic Evolution of Ancient Oceans | 4919 | ||
9.15.11.2.1. Strontium isotopes | 4919 | ||
9.15.11.2.2. Osmium isotopes | 4922 | ||
9.15.11.2.3. Sulfur isotopes | 4922 | ||
9.15.11.2.4. Carbon isotopes | 4924 | ||
9.15.11.2.5. Oxygen isotopes | 4927 | ||
9.15.11.2.6. Isotope tracers in developmental stages | 4929 | ||
9.15.12. Major Trends in the Evolution of Sediments during Geologic History | 4929 | ||
9.15.12.1. Overall Pattern of Lithologic Types | 4929 | ||
9.15.12.2. Phanerozoic Carbonate Rocks | 4930 | ||
9.15.12.2.1. Mass-age distribution and recycling rates | 4930 | ||
9.15.12.2.2. Dolomite/calcite ratios | 4932 | ||
9.15.12.2.3. Ooids and ironstones | 4934 | ||
9.15.12.2.4. Calcareous shelly fossils | 4935 | ||
9.15.12.2.5. The carbonate cycle in the ocean | 4936 | ||
9.15.12.3. Geochemical Implications of the Phanerozoic Carbonate Record | 4936 | ||
Acknowledgment | 4937 | ||
References | 4937 | ||
Chapter 9.16: Stable Isotopes in the Sedimentary Record | 4943 | ||
9.16.1. Introduction | 4943 | ||
9.16.2. Isotopic Concentration Units and Fractionation | 4947 | ||
9.16.3. Hydrogen and Oxygen Isotopes in the Water Cycle | 4948 | ||
9.16.4. Hydrogen and Oxygen Fractionation in Clays, Water, and Carbonates | 4951 | ||
9.16.4.1. Illite and Kaolinite Reactions with Water | 4952 | ||
9.16.4.2. Other Clay-Water Reactions | 4953 | ||
9.16.4.3. δ18O in Carbonates | 4955 | ||
9.16.5. Calcium Isotopes in Seawater and Carbonates | 4956 | ||
9.16.6. Carbon Isotopes in Carbonates and Organic Matter | 4959 | ||
9.16.6.1. Storage of Carbonates and Organic Matter | 4960 | ||
9.16.6.2. Long-Term Trends of Carbonate Sediments | 4962 | ||
9.16.7. Nitrogen Isotopes in Sedimentary Environment | 4965 | ||
9.16.7.1. Specific Analytical Aspects | 4966 | ||
9.16.7.2. N in the Mantle and Igneous Environment | 4966 | ||
9.16.7.3. N in Sedimentary Rocks | 4968 | ||
9.16.7.4. N in Oceanic Sediments and Particulate Matter | 4968 | ||
9.16.7.5. Anthropogenic Effects | 4970 | ||
9.16.8. Sulfur Isotopes in Sedimentary Sulfate and Sulfide | 4971 | ||
9.16.9. Boron Isotopes at the Earth's Surface | 4973 | ||
9.16.9.1. Ocean Water | 4974 | ||
9.16.9.2. Ground- and River Waters | 4975 | ||
9.16.9.3. Oceanic Sediments | 4976 | ||
9.16.9.4. Clay Mineral-Water Exchange | 4976 | ||
9.16.10. 40Ar in the Clay Fraction of Sediments | 4977 | ||
9.16.10.1. 40Ar in Clay Diagenesis | 4977 | ||
9.16.10.2. Clay Particle Size and K-Ar Apparent Age | 4978 | ||
9.16.10.3. 40Ar Loss in Thermal Events | 4981 | ||
9.16.10.4. 40Ar Flux from Sediments | 4981 | ||
Acknowledgments | 4983 | ||
References | 4983 | ||
Chapter 9.17: Geochemistry of Evaporites and Evolution of Seawater | 4989 | ||
9.17.1. Introduction | 4990 | ||
9.17.2. Definition of Evaporites | 4990 | ||
9.17.3. Brines and Evaporites | 4990 | ||
9.17.4. Environment of Evaporite Deposition | 4991 | ||
9.17.4.1. Evaporation | 4992 | ||
9.17.4.2. Freezing | 4992 | ||
9.17.4.3. Brine (Evaporating Waters) | 4993 | ||
9.17.4.4. Salinity | 4993 | ||
9.17.4.5. Temperature | 4994 | ||
9.17.4.6. Heliothermal Effect | 4995 | ||
9.17.4.7. pH | 4995 | ||
9.17.5. Seawater as a Salt Source for Evaporites | 4995 | ||
9.17.6. Evaporite and Saline Minerals | 4996 | ||
9.17.7. Model of Marginal Marine Evaporite Basin | 4997 | ||
9.17.7.1. Conceptual Model of the Basin | 4998 | ||
9.17.7.2. Quantitative Model of the Basin | 5001 | ||
9.17.8. Mode of Evaporite Deposition | 5002 | ||
9.17.9. Primary and Secondary Evaporites | 5004 | ||
9.17.10. Evaporation of Seawater - Experimental Approach | 5005 | ||
9.17.11. Crystallization Sequence before K–Mg Salt Precipitation | 5005 | ||
9.17.11.1. Early Salinity Rise - Calcium Carbonate Precipitation | 5005 | ||
9.17.11.2. Gypsum Crystallization Field | 5007 | ||
9.17.11.3. Halite Crystallization Field | 5007 | ||
9.17.12. Crystallization Sequence of K–Mg Salts | 5009 | ||
9.17.12.1. Natural Crystallization | 5009 | ||
9.17.12.2. Theoretical Crystallization Paths | 5010 | ||
9.17.13. Isotopic Effects in Evaporating Seawater Brines and Evaporite Salts | 5011 | ||
9.17.14. Usiglio Sequence - A Summary | 5011 | ||
9.17.15. Principles and Record of Chemical Evolution of Evaporating Seawater | 5011 | ||
9.17.15.1. Principle of the Chemical Divide for Seawater | 5011 | ||
9.17.15.2. Jänecke Diagrams | 5013 | ||
9.17.15.3. Spencer Triangle | 5014 | ||
9.17.16. Evaporation of Seawater - Remarks on Theoretical Approaches | 5015 | ||
9.17.17. Sulfate Deficiency in Ancient K-Mg Evaporites | 5015 | ||
9.17.17.1. Sulfate Deficiency as the Secondary Feature | 5017 | ||
9.17.17.2. Sulfate Deficiency as a Record of Ancient Seawater Composition | 5019 | ||
9.17.18. Ancient Ocean Chemistry Interpreted from Evaporites | 5020 | ||
9.17.18.1. Implications from Evaporite Mineralogy and from Usiglio Sequence | 5020 | ||
9.17.18.2. Implications of Primary Evaporite Minerals (Excluding Implications from Fluid Inclusions) | 5022 | ||
9.17.19. Recognition of Ancient Marine Evaporites | 5022 | ||
9.17.19.1. Sedimentological Criteria | 5023 | ||
9.17.19.2. Mineralogical Criteria | 5023 | ||
9.17.19.3. Geochemical Criteria | 5023 | ||
9.17.20. Fluid Inclusions Reveal the Composition of Ancient Brines | 5024 | ||
9.17.20.1. Criteria for Seawater Recognition in Halite Fluid Inclusions | 5025 | ||
9.17.20.2. Reconstruction of Ancient Seawater Composition from Halite Fluid Inclusions | 5026 | ||
9.17.21. Ancient Ocean Chemistry from Halite Fluid Inclusions - Summary and Comments | 5029 | ||
9.17.22. Salinity of Ancient Oceans | 5036 | ||
9.17.23. Evaporite Deposition through Time | 5037 | ||
9.17.23.1. Late Ediacaran-Phanerozoic Marine Evaporites | 5038 | ||
9.17.23.2. Precambrian (Pre-Ediacaran) Marine Evaporites | 5042 | ||
9.17.23.3. Nonmarine Evaporites in Precambrian | 5050 | ||
9.17.23.4. Pseudomorphs after Evaporite Minerals in Precambrian | 5050 | ||
9.17.24. Significance of Evaporites in the Earth History | 5052 | ||
9.17.24.1. Paleogeographic Indicators | 5052 | ||
9.17.24.2. Seals for Hydrocarbons and More (Evaporites and Hydrocarbons) | 5052 | ||
9.17.24.3. Halotectonics | 5053 | ||
9.17.24.4. Diagenesis and Metamorphism of Evaporites | 5053 | ||
9.17.25. Summary | 5053 | ||
Acknowledgments | 5054 | ||
References | 5054 | ||
Chapter 9.18: Iron Formations: Their Origins and Implications for Ancient Seawater Chemistry | 5067 | ||
9.18.1. Introduction | 5068 | ||
9.18.2. Definition of IF | 5069 | ||
9.18.3. Mineralogy of IF | 5072 | ||
9.18.3.1. Precursor Sediments | 5074 | ||
9.18.3.1.1. Secular trend in Fe mineralogy of GIFs | 5075 | ||
9.18.4. Depositional Setting and Sequence-Stratigraphic Framework | 5075 | ||
9.18.4.1. Basin-Type Control on IF Deposition | 5077 | ||
9.18.4.2. Sedimentation Rates | 5078 | ||
9.18.5. IF: A Proxy for Ancient Seawater Composition | 5079 | ||
9.18.5.1. Trace Elements | 5079 | ||
9.18.5.1.1. Rare earth elements | 5079 | ||
9.18.5.1.2. Phosphorus | 5080 | ||
9.18.5.1.3. Nickel | 5083 | ||
9.18.5.1.4. Chromium | 5083 | ||
9.18.5.2. Stable Isotope Studies of IF | 5085 | ||
9.18.5.2.1. Traditional light stable isotopes | 5085 | ||
9.18.5.2.2. Nontraditional stable isotopes | 5087 | ||
9.18.5.2.2.1. Fe isotopes | 5087 | ||
9.18.5.2.2.2. Chromium isotopes | 5089 | ||
9.18.5.2.2.3. Silicon isotopes in chert and IF bands | 5089 | ||
9.18.6. Perspective from the Modern Iron Cycle | 5090 | ||
9.18.6.1. Hydrothermal Pulses of Si Synchronous with Fe Addition to Seawater | 5092 | ||
9.18.6.2. Oxidation Mechanism: Biological versus Nonbiological | 5092 | ||
9.18.6.2.1. Oxidation of Fe(II) by cyanobacterial O2 | 5094 | ||
9.18.6.2.2. Metabolic Fe(II) oxidation | 5094 | ||
9.18.6.2.3. Ultraviolet photooxidation of Fe(II) | 5095 | ||
9.18.7. Secular Trends for Exhalites, IFs, and VMS Deposits | 5095 | ||
9.18.7.1. Relationships among Mantle Plumes, IF | 5095 | ||
9.18.7.2. Secular Patterns in Precambrian VMS-Related Exhalites | 5097 | ||
9.18.7.3. Secular Patterns in Sedimentary Iron Deposits | 5098 | ||
9.18.7.3.1. Eoarchean IFs | 5098 | ||
9.18.7.3.2. Paleoarchean IFs | 5098 | ||
9.18.7.3.3. Neoarchean to Mesoarchean IFs | 5098 | ||
9.18.7.3.4. Neoarchean IFs | 5099 | ||
9.18.7.3.5. IFs deposited after the GOE and before 1.93Ga | 5099 | ||
9.18.7.3.6. C.1.93-1.85Ga IFs coeval with large VMS de | 5100 | ||
9.18.7.3.7. Proterozoic age gap in major IF deposition | 5102 | ||
9.18.7.3.8. Neoproterozoic manganese deposits and IFs | 5103 | ||
9.18.7.3.9. Phanerozoic ironstones, anoxic events, and VMS deposits | 5104 | ||
9.18.8. Controls on IF Deposition | 5106 | ||
9.18.8.1. Influence of Hydrothermal Processes on Ocean Composition and Organic Productivity | 5108 | ||
9.18.8.2. Implications for Atmospheric Oxidation | 5111 | ||
9.18.9. Euxinic Conditions Induced by Shift in Dissolved Fe/S Ratio of Seawater due to Iron Oxidation | 5111 | ||
9.18.10. Research Perspectives and Future Directions | 5112 | ||
Appendix 1. Precambrian Banded Iron Formations, Granular Iron Formations, and Rapitan-Type Iron Formationsa | 5113 | ||
Appendix 2. Exhalites Associated with Precambrian Deep-Water (Cu-Rich) Volcanogenic Massive Sulfide Depositsa | 5120 | ||
References | 5123 | ||
Chapter 9.19: Bedded Barite Deposits: Environments of Deposition, Styles of Mineralization, and Tectonic Settings | 5135 | ||
9.19.1. Introduction | 5135 | ||
9.19.1.1. Modern Deposits of Massive Barite | 5136 | ||
9.19.1.2. Ancient Deposits of Massive Barite | 5137 | ||
9.19.1.3. Setting I. Continental Rifts (e.g., Red Dog) | 5138 | ||
9.19.1.3.1. Regional setting | 5139 | ||
9.19.1.3.2. Mudstone host | 5140 | ||
9.19.1.3.3. Volcanic rocks | 5141 | ||
9.19.1.3.4. Mineralization | 5141 | ||
9.19.1.3.5. Fluid flow | 5143 | ||
9.19.1.4. Setting II. Transtensional Basins (e.g., California Borderland) | 5144 | ||
9.19.1.4.1. Regional setting | 5144 | ||
9.19.1.4.2. Mudstone host | 5145 | ||
9.19.1.4.3. Volcanic rocks | 5146 | ||
9.19.1.4.4. Mineralization | 5147 | ||
9.19.1.4.5. Fluid flow | 5148 | ||
9.19.1.5. Setting III. Accretionary Prisms (e.g., Arkansas) | 5150 | ||
9.19.1.5.1. Regional setting | 5150 | ||
9.19.1.5.2. Mudstone host | 5150 | ||
9.19.1.5.3. Volcanic rocks | 5151 | ||
9.19.1.5.4. Mineralization | 5152 | ||
9.19.1.5.5. Fluid flow | 5153 | ||
9.19.2. Comparisons | 5154 | ||
9.19.2.1. Environments of Deposition | 5155 | ||
9.19.2.2. Mineralization | 5155 | ||
9.19.2.3. Tectonic Setting | 5155 | ||
9.19.3. The Nevada Barites: A Test Case | 5155 | ||
9.19.3.1. Host Strata | 5155 | ||
9.19.3.2. Mineralization | 5156 | ||
9.19.3.3. Tectonic Setting | 5157 | ||
9.19.4. Summary | 5157 | ||
Acknowledgments | 5159 | ||
References | 5159 | ||
e9780080983004v10 | 5163 | ||
Front Cover | 5163 | ||
Biogeochemistry | 5166 | ||
Copyright | 5167 | ||
In Memoriam | 5168 | ||
Heinrich Dieter Holland (1927–2012) | 5170 | ||
Karl Karekin Turekian (1927–2013) | 5172 | ||
References | 5174 | ||
Dedication | 5176 | ||
Contents | 5178 | ||
Executive Editors’ Foreword to the Second Edition | 5180 | ||
Contributors | 5184 | ||
Volume Editors’ Introduction | 5186 | ||
References | 5188 | ||
Chapter 10.1: The Early History of Life | 5190 | ||
10.1.1. Introduction | 5191 | ||
10.1.1.1. Strangeness and Familiarity - the Youth of the Earth | 5191 | ||
10.1.1.2. Evidence in Rocks, Moon, Planets, and Meteorites - the Sources of Information | 5191 | ||
10.1.1.3. Reading the Palimpsests – Using Evidence from the Modern Earth and Biology to Reconstruct the Ancestors and their Home | 5192 | ||
10.1.1.4. Modeling - The Problem of Taking Fragments of Evidence and Rebuilding the Childhood of the Planet | 5192 | ||
10.1.1.5. What Does a Planet Need to be Habitable? | 5193 | ||
10.1.1.6. The Power of Biology: The Infinite Improbability Drive | 5193 | ||
10.1.2. The Chaotian and Hadean (~4.56–4.0 Ga Ago) | 5194 | ||
10.1.2.1. Definition of the Chaotian | 5194 | ||
10.1.2.2. Definition of the Hadean | 5194 | ||
10.1.2.3. Building a Habitable Planet | 5194 | ||
10.1.2.4. The Hadean Geological Record | 5196 | ||
10.1.2.5. When and Where Did Life Start? | 5197 | ||
10.1.3. The Archean (~4-2.5Ga Ago) | 5197 | ||
10.1.3.1. Definition of Archean | 5197 | ||
10.1.3.2. The Archean Record | 5198 | ||
10.1.3.2.1. Greenland | 5198 | ||
10.1.3.2.2. Barberton | 5198 | ||
10.1.3.2.3. Western Australia | 5198 | ||
10.1.3.2.4. Steep Rock, Ontario, and Pongola, South Africa | 5199 | ||
10.1.3.2.5. Belingwe | 5200 | ||
10.1.4. The Functioning of the Earth System in the Archean | 5201 | ||
10.1.4.1. The Physical State of the Archean Planet | 5201 | ||
10.1.4.2. The Surface Environment | 5202 | ||
10.1.5. Life: Early Setting and Impact on the Environment | 5203 | ||
10.1.5.1. Origin of Life | 5203 | ||
10.1.5.2. RNA World | 5204 | ||
10.1.5.3. Hydrothermal World | 5204 | ||
10.1.5.4. LUCA - The Last Common Ancestor | 5204 | ||
10.1.5.5. A Hyperthermophile Heritage? | 5208 | ||
10.1.5.6. Metabolic Strategies | 5209 | ||
10.1.6. The Early Biomes | 5210 | ||
10.1.6.1. Location of Early Biomes | 5210 | ||
10.1.6.2. Methanogenesis: Impact on the Environment | 5210 | ||
10.1.6.3. Prephotosynthetic Ecology | 5211 | ||
10.1.6.4. Geological Settings of the Early Biomes | 5212 | ||
10.1.7. The Evolution of Photosynthesis | 5213 | ||
10.1.7.1. The Chain of Photosynthesis | 5213 | ||
10.1.7.2. The Rubisco Fingerprint | 5214 | ||
10.1.7.3. The Evolutionary Chain | 5215 | ||
10.1.7.4. Anoxygenic Photosynthesis | 5216 | ||
10.1.7.5. Oxygenic Photosynthesis | 5217 | ||
10.1.7.6. Archean Oxygen | 5218 | ||
10.1.8. Mud-Stirrers: Origin and Impact of the Eucarya | 5219 | ||
10.1.8.1. The Ancestry of the Eucarya | 5219 | ||
10.1.8.2. The Last Eukaryote Common Ancestor: Possible Settings for the Eukaryote Endosymbiotic Event | 5221 | ||
10.1.8.3. Water and Mud Stirring - Consequences | 5222 | ||
10.1.9. The breath of Life: The Impact of Life on the Ocean/Atmosphere System | 5223 | ||
10.1.9.1. The Breath of Life | 5223 | ||
10.1.9.2. Oxygen and Carbon Dioxide | 5223 | ||
10.1.9.3. Nitrogen and Fixed Nitrogen | 5224 | ||
10.1.9.4. Methane | 5225 | ||
10.1.9.5. Sulfur and Biology | 5225 | ||
10.1.10. Feedback from the Biosphere to the Physical State of the Planet | 5225 | ||
Acknowledgments | 5226 | ||
References | 5226 | ||
Chapter 10.2: Evolution of Metabolism | 5232 | ||
10.2.1. Introduction | 5232 | ||
10.2.2. The Domains of Life | 5232 | ||
10.2.3. Life and Rocks | 5233 | ||
10.2.4. Mechanisms for Energy Conservation | 5233 | ||
10.2.5. Extant Patterns of Metabolism | 5235 | ||
10.2.5.1. Kinds of Phototrophs | 5236 | ||
10.2.5.2. Lithotrophic Energy Sources | 5237 | ||
10.2.5.3. Carbon Sources for Life | 5237 | ||
10.2.5.4. Fermentative and Respiratory Metabolism | 5238 | ||
10.2.6. Reconstructing the Evolution of Metabolism | 5239 | ||
10.2.6.1. Approaches Employing Genomics and Molecular Genetics | 5239 | ||
10.2.6.2. Approaches Employing Geochemical and Geophysical Methods | 5240 | ||
10.2.6.2.1. Physical fossils | 5240 | ||
10.2.6.2.2. Signature molecules | 5241 | ||
10.2.6.2.3. Inorganic chemistry of sediments | 5241 | ||
10.2.6.2.4. Stable isotope fractionation | 5242 | ||
10.2.6.2.5. Carbon isotopes | 5242 | ||
10.2.6.2.6. Sulfur isotopes | 5244 | ||
10.2.6.2.7. Nitrogen isotopes | 5244 | ||
10.2.6.2.8. Iron isotopes | 5244 | ||
10.2.7. Overview | 5245 | ||
References | 5246 | ||
Chapter 10.3: Sedimentary Hydrocarbons, Biomarkers for Early Life | 5250 | ||
10.3.1. Introduction | 5250 | ||
10.3.2. Biomarkers as Molecular Fossils | 5251 | ||
10.3.2.1. The Fate of Dead Biomass: Diagenesis, Catagenesis, and Metagenesis | 5251 | ||
10.3.2.2. Compound-Specific Stable Isotopes | 5253 | ||
10.3.3. Thermal Stability and Maturity of Biomarkers | 5253 | ||
10.3.3.1. Biomarkers as Maturity Indicators | 5253 | ||
10.3.3.2. The Survival of Biomarkers with Increasing Temperature and Time | 5254 | ||
10.3.4. Experimental Approaches to Biomarker and Kerogen Analysis | 5256 | ||
10.3.5. Discussion of Biomarkers by Hydrocarbon Class | 5257 | ||
10.3.5.1. Advantages and Limitations of the Biomarker Approach | 5257 | ||
10.3.5.2. n-Alkanes, Algaenans, and other Polymethylenic Biopolymers | 5257 | ||
10.3.5.3. Methyl and Ethyl Alkanes | 5259 | ||
10.3.5.4. Alkyl Cyclohexanes and Cyclopentanes | 5260 | ||
10.3.5.5. Isoprenoids | 5260 | ||
10.3.5.6. Carotenoids | 5263 | ||
10.3.5.6.1. Aromatic carotenoids and arylisoprenoids | 5264 | ||
10.3.5.6.2. Bacterioruberin | 5267 | ||
10.3.5.7. Chlorophylls and Maleimides | 5267 | ||
10.3.5.8. Sesquiterpanes (C15) and Diterpanes (C20) | 5268 | ||
10.3.5.9. Cheilanthanes and other Tricyclic Polyprenoids | 5270 | ||
10.3.5.10. Hopanoids and other Pentacyclic Triterpanes | 5270 | ||
10.3.5.11. Steroid Hydrocarbons | 5273 | ||
10.3.6. Reconstruction of Ancient Biospheres: Biomarkers for the Three Domains of Life | 5276 | ||
10.3.6.1. Bacteria | 5276 | ||
10.3.6.1.1. Hopanoids as biomarkers for bacteria | 5276 | ||
10.3.6.1.2. Cyanobacteria | 5276 | ||
10.3.6.1.3. Methanotrophs, methylotrophs, and acetic acid bacteria | 5276 | ||
10.3.6.1.4. Phototrophic sulfur bacteria | 5276 | ||
10.3.6.2. Archaea | 5277 | ||
10.3.6.2.1. Methanogens | 5277 | ||
10.3.6.2.2. Biomarkers and ecology at marine methane seeps | 5277 | ||
10.3.6.2.3. Halobacteria | 5278 | ||
10.3.6.2.4. Marine Crenarchaeota | 5278 | ||
10.3.6.3. Eukarya | 5278 | ||
10.3.7. Biomarkers as Environmental Indicators | 5278 | ||
10.3.7.1. Marine versus Lacustrine Conditions | 5278 | ||
10.3.7.2. Hypersaline Conditions | 5279 | ||
10.3.7.3. Anoxic and Euxinic Conditions | 5280 | ||
10.3.7.4. Carbonates versus Clay-rich Sediments | 5280 | ||
10.3.7.5. Paleotemperature and Paleolatitude Biomarkers | 5281 | ||
10.3.8. Age Diagnostic Biomarkers | 5281 | ||
10.3.9. Biomarkers in Precambrian Rocks | 5281 | ||
10.3.9.1. Biomarkers in the Proterozoic (0.54-2.5 Ga) | 5281 | ||
10.3.9.2. Biomarkers Extracted from Archean Rocks (>2.5 Ga) | 5282 | ||
10.3.10. Outlook | 5283 | ||
Acknowledgments | 5283 | ||
References | 5283 | ||
Chapter 10.4: Biomineralization | 5294 | ||
10.4.1. Introduction | 5295 | ||
10.4.1.1. Outline of the Chapter | 5295 | ||
10.4.1.2. Definitions and General Background on Biomineralization | 5296 | ||
10.4.2. Biominerals | 5297 | ||
10.4.2.1. Calcium Carbonates | 5297 | ||
10.4.2.1.1. Calcite | 5297 | ||
10.4.2.1.2. Aragonite | 5297 | ||
10.4.2.1.3. Vaterite | 5299 | ||
10.4.2.2. Silica | 5300 | ||
10.4.2.2.1. Opal | 5300 | ||
10.4.2.3. Bioapatite | 5301 | ||
10.4.2.4. Iron Oxides and Hydroxides | 5303 | ||
10.4.2.4.1. Magnetite | 5303 | ||
10.4.3. Examples of Biomineralization | 5304 | ||
10.4.3.1. Introduction | 5304 | ||
10.4.3.2. Sulfur Biomineralization | 5305 | ||
10.4.3.2.1. Sulfur oxidizers | 5305 | ||
10.4.3.2.2. Sulfate reducers | 5305 | ||
10.4.3.2.3. Formation of elemental sulfur | 5305 | ||
10.4.3.2.4. Sulfate biomineralization | 5306 | ||
10.4.3.3. Iron Biomineralization | 5306 | ||
10.4.3.3.1. Roles of iron in archaea and bacteria | 5306 | ||
10.4.3.3.2. Bacterial iron mineral formation | 5306 | ||
10.4.3.3.3. Magnetotactic bacteria | 5307 | ||
10.4.3.4. Carbonate Biomineralization | 5307 | ||
10.4.3.4.1. Cyanobacteria | 5309 | ||
10.4.3.4.2. Cnidaria (coelenterates) | 5310 | ||
10.4.3.4.2.1. Corals | 5310 | ||
10.4.3.4.2.2. Other taxa that biomineralize | 5310 | ||
10.4.3.4.3. Coccoliths | 5311 | ||
10.4.3.4.3.1. E. huxleyi: Intracellular calcification | 5311 | ||
10.4.3.4.4. Foraminifera | 5311 | ||
10.4.3.4.5. Echinoids | 5313 | ||
10.4.3.4.6. Mollusks | 5314 | ||
10.4.3.4.6.1. Bivalves | 5314 | ||
10.4.3.4.6.2. Bivalve shell architecture | 5314 | ||
10.4.3.4.6.3. Chitons | 5314 | ||
10.4.3.4.6.4. Teeth of chitons | 5316 | ||
10.4.3.4.7. Arthropods | 5316 | ||
10.4.3.4.7.1. Crustacea exoskeleton: The carapace | 5317 | ||
10.4.3.4.7.2. Gastroliths | 5317 | ||
10.4.3.5. Silica Biomineralization | 5317 | ||
10.4.3.5.1. Radiolarians | 5318 | ||
10.4.3.5.2. Diatoms | 5319 | ||
10.4.3.5.3. Sponges | 5321 | ||
10.4.3.5.4. Plant biominerals | 5323 | ||
10.4.3.5.4.1. Silica in plants | 5323 | ||
10.4.3.5.5. Phytoliths: Indicators of the environment and paleoenvironment | 5327 | ||
10.4.3.5.5.1. Radiocarbon dating using plant biominerals | 5329 | ||
10.4.3.5.5.2. Stable isotope investigations on plant biominerals | 5329 | ||
10.4.3.5.6. Bones and bone tissues | 5329 | ||
10.4.3.5.6.1. Biomineralization of bone: The bone morphogenic unit | 5329 | ||
10.4.3.5.6.2. Bone cells and products: Collagen | 5332 | ||
10.4.3.5.6.3. Matrix-mediated biomineralization and other possibilities | 5333 | ||
10.4.3.5.6.4. Analyses of the bone | 5335 | ||
10.4.3.5.7. Cartilage | 5335 | ||
10.4.3.5.7.1. Biomineralization of cartilage | 5337 | ||
10.4.3.5.8. Antlers | 5337 | ||
10.4.3.5.9. Teeth | 5337 | ||
10.4.3.5.9.1. Introduction | 5337 | ||
10.4.3.5.9.2. Tooth biology and mineralogy | 5339 | ||
10.4.3.5.10. Otoliths | 5341 | ||
10.4.4. Summary: Why Biomineralize? | 5341 | ||
10.4.4.1. Physical or Macrobiomineralization Contributions | 5341 | ||
10.4.4.1.1. Defense and protection through biomineralization | 5343 | ||
10.4.4.2. Chemical or Microbiomineralization Contributions | 5343 | ||
Acknowledgments | 5344 | ||
References | 5344 | ||
Chapter 10.5: Biogeochemistry of Primary Production in the Sea | 5352 | ||
10.5.1. Introduction | 5352 | ||
10.5.1.1. The Two Carbon Cycles | 5353 | ||
10.5.1.2. A Primer on Redox Chemistry | 5353 | ||
10.5.2. Chemoautotrophy | 5353 | ||
10.5.3. Photoautotrophy | 5354 | ||
10.5.3.1. Selective Forces in the Evolution of Photoautotrophy | 5354 | ||
10.5.3.2. Selective Pressure in the Evolution of Oxygenic Photosynthesis | 5355 | ||
10.5.4. Primary Productivity by Photoautotrophs | 5355 | ||
10.5.4.1. What Are Photoautotrophs? | 5356 | ||
10.5.4.1.1. The red and green lineages | 5357 | ||
10.5.4.2. Estimating Chlorophyll Biomass | 5357 | ||
10.5.4.2.1. Satellite-based algorithms for ocean color retrievals | 5357 | ||
10.5.4.3. Estimating Net Primary Production | 5359 | ||
10.5.4.3.1. Global models of net primary production for the ocean | 5359 | ||
10.5.4.4. Quantum Efficiency of NPP | 5360 | ||
10.5.4.4.1. Comparing efficiencies for oceanic and terrestrial primary production | 5360 | ||
10.5.5. Export, New, and `True New` Production | 5361 | ||
10.5.5.1. Steady State Versus Transient State | 5362 | ||
10.5.5.2. Climate Change and the Transient State | 5362 | ||
10.5.6. Nutrient Fluxes | 5363 | ||
10.5.6.1. The Redfield Ratio | 5363 | ||
10.5.7. Nitrification | 5363 | ||
10.5.7.1. Carbon Burial | 5364 | ||
10.5.7.2. Carbon Isotope Fractionation in Organic Matter and Carbonates | 5364 | ||
10.5.7.3. Balance Between Net Primary Production and Losses | 5364 | ||
10.5.7.4. Carbon Burial in the Contemporary Ocean | 5364 | ||
10.5.7.5. Carbon Burial in the Precambrian Ocean | 5366 | ||
10.5.8. Limiting Macronutrients | 5366 | ||
10.5.8.1. The Two Concepts of Limitation | 5366 | ||
10.5.9. The Evolution of the Nitrogen Cycle | 5366 | ||
10.5.10. Functional Groups | 5367 | ||
10.5.10.1. Siliceous Organisms | 5368 | ||
10.5.10.2. Calcium Carbonate Precipitation | 5369 | ||
10.5.10.3. Vacuoles | 5369 | ||
10.5.11. High-Nutrient, Low-Chlorophyll Regions: Iron Limitation | 5370 | ||
10.5.12. Glacial-Interglacial Changes in the Biological CO2 Pump | 5370 | ||
10.5.13. Iron Stimulation of Nutrient Utilization | 5371 | ||
10.5.14. Linking Iron to N2 Fixation | 5371 | ||
10.5.15. Other Trace-Element Controls on NPP | 5371 | ||
10.5.16. Concluding Remarks | 5373 | ||
Acknowledgments | 5373 | ||
References | 5373 | ||
Chapter 10.6: Biogeochemical Interactions Governing Terrestrial Net Primary Production | 5378 | ||
10.6.1. Introduction | 5379 | ||
10.6.2. General Constraints on NPP | 5379 | ||
10.6.2.1. What is NPP? | 5379 | ||
10.6.2.2. Physiological Controls over NPP | 5380 | ||
10.6.2.3. Environmental Controls over NPP | 5381 | ||
10.6.2.4. Species Controls over NPP | 5384 | ||
10.6.3. Limitations to Leaf-Level Carbon Gain | 5384 | ||
10.6.3.1. The Basic Recipe for Carbon Gain | 5384 | ||
10.6.3.2. CO2 Limitation | 5384 | ||
10.6.3.3. Light Limitation | 5385 | ||
10.6.3.4. Nitrogen Limitation | 5385 | ||
10.6.3.5. Water Limitation | 5386 | ||
10.6.4. Stand-Level Carbon Gain | 5386 | ||
10.6.4.1. Spatial Scaling of GPP | 5386 | ||
10.6.4.2. Temporal Scaling of GPP | 5387 | ||
10.6.5. Respiration | 5388 | ||
10.6.6. Allocation of NPP | 5390 | ||
10.6.7. Tissue Turnover | 5390 | ||
10.6.8. Global Patterns of Biomass and NPP | 5390 | ||
10.6.9. Nutrient Use | 5391 | ||
10.6.10. Balancing Nutrient Limitation | 5392 | ||
10.6.10.1. Nutrient Requirements | 5392 | ||
10.6.10.2. Limitation by Different Nutrients | 5392 | ||
10.6.10.3. Stoichiometry of NPP | 5393 | ||
10.6.10.4. Uncoupling Mechanisms | 5394 | ||
10.6.10.4.1. Litterfall and leaching inputs | 5394 | ||
10.6.10.4.2. Nutrient mineralization | 5395 | ||
10.6.10.4.3. Nutrient availability | 5396 | ||
10.6.10.4.4. Element interactions | 5396 | ||
10.6.10.4.5. Plant uptake | 5396 | ||
10.6.10.5. Recoupling Mechanisms | 5397 | ||
10.6.11. Community-Level Adjustments | 5398 | ||
10.6.12. Species Effects on Interactive Controls | 5398 | ||
10.6.12.1. Species Effects on Resources | 5398 | ||
10.6.12.1.1. Decomposition and nitrogen mineralization | 5398 | ||
10.6.12.1.2. Water dynamics | 5399 | ||
10.6.12.2. Species Effects on Climate | 5399 | ||
10.6.12.3. Species Effects on Disturbance | 5400 | ||
10.6.13. Species Interactions and Ecosystem Processes | 5400 | ||
10.6.14. Summary | 5400 | ||
Acknowledgments | 5401 | ||
References | 5401 | ||
Chapter 10.7: Biogeochemistry of Decomposition and Detrital Processing | 5406 | ||
10.7.1. Introduction | 5406 | ||
10.7.2. Composition of Decomposer Resources | 5407 | ||
10.7.2.1. Plant Litter | 5408 | ||
10.7.2.2. Roots | 5409 | ||
10.7.2.3. Secondary Resources | 5410 | ||
10.7.2.4. Soil Organic Matter | 5410 | ||
10.7.2.5. Summary | 5411 | ||
10.7.3. The Decomposer Organisms | 5411 | ||
10.7.3.1. Functional Ecology | 5412 | ||
10.7.3.2. Soil Microorganisms | 5412 | ||
10.7.3.3. Soil Fauna | 5415 | ||
10.7.3.4. Interactions | 5418 | ||
10.7.4. Methods for Studying Decomposition | 5419 | ||
10.7.4.1. Litter Techniques | 5419 | ||
10.7.4.2. SOM Techniques | 5420 | ||
10.7.5. Detrital Processing | 5421 | ||
10.7.5.1. Time Course of Litter Decomposition | 5421 | ||
10.7.5.2. Comminution | 5424 | ||
10.7.5.3. Leaching | 5424 | ||
10.7.5.4. Catabolism | 5426 | ||
10.7.5.5. Change in Nutrient Status | 5430 | ||
10.7.5.6. Priming Effect on Native SOM | 5432 | ||
10.7.6. Humification | 5432 | ||
10.7.6.1. Selective Preservation | 5433 | ||
10.7.6.2. Condensation Models | 5433 | ||
10.7.7. Control of Decomposition and Stabilization | 5434 | ||
10.7.7.1. Decomposer Organisms | 5435 | ||
10.7.7.2. Resource Quality | 5437 | ||
10.7.7.3. Soil Characteristics | 5441 | ||
10.7.7.4. Climate | 5445 | ||
10.7.7.5. Multiple Constraints | 5451 | ||
10.7.8. Modeling Approaches | 5451 | ||
10.7.9. Conclusions | 5453 | ||
References | 5454 | ||
Chapter 10.8: Anaerobic Metabolism: Linkages to Trace Gases and Aerobic Processes | 5462 | ||
10.8.1. Overview of Life in the Absence of O2 | 5463 | ||
10.8.1.1. Introduction | 5463 | ||
10.8.1.2. Overview of Anaerobic Metabolism | 5464 | ||
10.8.1.3. Anaerobic-Aerobic Interface Habitats | 5465 | ||
10.8.1.4. Syntax of Metabolism | 5466 | ||
10.8.2. Autotrophic Metabolism | 5466 | ||
10.8.2.1. Phototroph (Photolithoautotrophy) Diversity and Metabolism | 5466 | ||
10.8.2.2. Chemotroph (Chemolithoautotrophy) Diversity and Metabolism | 5468 | ||
10.8.2.3. Pathways of CO2 Fixation | 5469 | ||
10.8.3. Decomposition and Fermentation | 5469 | ||
10.8.3.1. Polymer Degradation | 5469 | ||
10.8.3.1.1. Polysaccharides | 5470 | ||
10.8.3.1.2. Lignin | 5471 | ||
10.8.3.2. Fermentation | 5471 | ||
10.8.3.2.1. Acetogenesis | 5472 | ||
10.8.3.2.2. Syntrophy and interspecies hydrogen transfer | 5472 | ||
10.8.4. Methane | 5475 | ||
10.8.4.1. Methane in the Environment | 5475 | ||
10.8.4.2. Methanogen Diversity and Metabolism | 5476 | ||
10.8.4.3. Regulation of Methanogenesis | 5477 | ||
10.8.4.3.1. O2 and oxidant inhibition | 5477 | ||
10.8.4.3.2. Nutrients and pH | 5477 | ||
10.8.4.3.3. Temperature | 5477 | ||
10.8.4.3.4. Carbon quantity and quality | 5478 | ||
10.8.4.3.5. Plants as carbon sources | 5479 | ||
10.8.4.4. Contributions of Acetotrophy versus Hydrogenotrophy | 5480 | ||
10.8.4.4.1. Organic carbon availability | 5480 | ||
10.8.4.4.2. Temperature | 5482 | ||
10.8.4.5. Anaerobic Methane Oxidation | 5483 | ||
10.8.4.6. Aerobic Methane Oxidation | 5485 | ||
10.8.4.6.1. Methanotroph diversity | 5485 | ||
10.8.4.6.2. Regulation of methanotrophy | 5486 | ||
10.8.4.6.3. Methane oxidation efficiency | 5487 | ||
10.8.4.7. Wetland Methane Emissions and Global Change | 5488 | ||
10.8.5. Nitrogen | 5489 | ||
10.8.5.1. Nitrogen in the Environment | 5489 | ||
10.8.5.2. Nitrogen Fixation | 5491 | ||
10.8.5.3. Respiratory Denitrification | 5491 | ||
10.8.5.3.1. Denitrifier diversity and metabolism | 5491 | ||
10.8.5.3.2. Regulation of denitrification | 5492 | ||
10.8.5.3.3. Nitrification-denitrification coupling | 5494 | ||
10.8.5.3.4. Animals and plants | 5494 | ||
10.8.5.3.5. N2O and NO fluxes | 5495 | ||
10.8.5.4. Dissimilatory Nitrate Reduction to Ammonium (DNRA) | 5495 | ||
10.8.5.4.1. Physiology and diversity of DNRA bacteria | 5495 | ||
10.8.5.4.2. DNRA versus denitrification | 5496 | ||
10.8.5.5. Alternative Pathways to N2 Production | 5497 | ||
10.8.5.5.1. Anammox | 5497 | ||
10.8.5.5.2. Nitrifier denitrification | 5499 | ||
10.8.5.5.3. Abiotic and autotrophic denitrification | 5499 | ||
10.8.6. Iron and Manganese | 5500 | ||
10.8.6.1. Iron and Manganese in the Environment | 5501 | ||
10.8.6.2. Iron and Manganese Geochemistry | 5501 | ||
10.8.6.3. Microbial Reduction of Iron and Manganese | 5501 | ||
10.8.6.3.1. Metabolic diversity in Fe(III)- and Mn(IV)-reducing organisms | 5502 | ||
10.8.6.3.2. Physical mechanisms for accessing oxides | 5502 | ||
10.8.6.4. Factors that Regulate Fe(III) Reduction | 5503 | ||
10.8.6.4.1. Electron shuttling compounds | 5503 | ||
10.8.6.4.2. Fe(lll) chelators | 5504 | ||
10.8.6.4.3. Mineral reactivity | 5504 | ||
10.8.6.4.4. Abiotic versus biotic reduction | 5505 | ||
10.8.6.4.5. Separating enzymatic and nonenzymatic Fe(lll) reduction | 5506 | ||
10.8.6.4.6. Fe(III) reduction in ecosystems | 5506 | ||
10.8.6.5. Microbial Oxidation of Iron and Manganese | 5507 | ||
10.8.6.5.1. Energetics of Fe(ll) and Mn(ll) oxidation | 5507 | ||
10.8.6.5.2. Anaerobic Fe(ll) oxidation | 5507 | ||
10.8.6.5.3. Aerobic Fe(ll) oxidation | 5508 | ||
10.8.6.6. Iron Cycling | 5509 | ||
10.8.7. Sulfur | 5509 | ||
10.8.7.1. Sulfur Geochemistry | 5510 | ||
10.8.7.2. Microbial Reduction of Sulfate | 5510 | ||
10.8.7.2.1. Overview of sulfate reduction | 5510 | ||
10.8.7.2.2. Metabolic diversity | 5511 | ||
10.8.7.3. Taxonomic Considerations | 5513 | ||
10.8.7.4. Sulfate-Reducing Populations | 5514 | ||
10.8.7.5. Factors Regulating Sulfate Reduction Activity | 5514 | ||
10.8.7.5.1. Sulfate-reducing activity | 5514 | ||
10.8.7.5.2. Temperature | 5515 | ||
10.8.7.5.3. Carbon | 5515 | ||
10.8.7.5.4. Sulfate and molecular oxygen concentrations | 5515 | ||
10.8.7.6. Microbial Reduction of Sulfur | 5516 | ||
10.8.7.7. Disproportionation | 5516 | ||
10.8.7.8. Sulfur Gases | 5517 | ||
10.8.7.8.1. Hydrogen sulfide | 5517 | ||
10.8.7.8.2. Methylsulfides | 5517 | ||
10.8.7.8.3. Carbonyl sulfide and carbon disulfide | 5519 | ||
10.8.7.9. Microbial Oxidation of Sulfur | 5519 | ||
10.8.7.9.1. Colorless sulfur bacteria | 5520 | ||
10.8.7.9.2. Single-cell colorless sulfur bacteria | 5520 | ||
10.8.7.9.3. Filamentous colorless sulfur bacteria | 5521 | ||
10.8.7.9.4. Anoxyphototrophic bacteria | 5521 | ||
10.8.7.9.5. Ecological aspects of sulfide oxidation | 5522 | ||
10.8.8. Coupled Anaerobic Element Cycles | 5522 | ||
10.8.8.1. Evidence of Competitive Interactions | 5522 | ||
10.8.8.2. Mechanisms of Competition | 5522 | ||
10.8.8.3. Exceptions | 5523 | ||
10.8.8.4. Noncompetitive Interactions | 5524 | ||
10.8.8.5. Contributions to Carbon Metabolis | 5524 | ||
10.8.8.6. Concluding Remarks | 5524 | ||
Acknowledgments | 5524 | ||
References | 5525 | ||
Chapter 10.9: The Geologic History of the Carbon Cycle | 5550 | ||
10.9.1. Introduction | 5550 | ||
10.9.2. Modes of Carbon-Cycle Change | 5551 | ||
10.9.2.1. The Carbon Cycle over Geologic Timescales | 5551 | ||
10.9.2.1.1. The ``carbon dioxide´´ carbon cycle | 5551 | ||
10.9.2.1.2. The ``methane´´ carbon subcycle | 5554 | ||
10.9.2.2. Timescales of Carbon-cycle Change | 5557 | ||
10.9.3. The Quaternary Record of Carbon-Cycle Change | 5558 | ||
10.9.3.1. Analysis of CO2 and CH4 in Ice Cores | 5558 | ||
10.9.3.2. Holocene Carbon-cycle Variations | 5562 | ||
10.9.3.3. Glacial/interglacial Carbon-cycle Variations | 5566 | ||
10.9.3.3.1. Carbon-cycle influences on glacial/interglacial climate | 5566 | ||
10.9.3.3.2. Climate influences on glacial/interglacial carbon cycling | 5568 | ||
10.9.3.3.3. Carbon/climate interactions at glacial terminations | 5571 | ||
10.9.4. The Phanerozoic Record of Carbon-Cycle Change | 5572 | ||
10.9.4.1. Mechanisms of Gradual Geologic Carbon-cycle Change | 5573 | ||
10.9.4.1.1. The carbonate weathering-sedimentation cycle | 5573 | ||
10.9.4.1.2. The silicate-carbonate weathering-decarbonation cycle | 5573 | ||
10.9.4.1.3. The organic carbon production-consumption-oxidation cycle | 5574 | ||
10.9.4.2. Model Simulations of Gradual Geologic Carbon-cycle Change | 5576 | ||
10.9.4.3. Geologic Evidence for Phanerozoic Atmospheric CO2 Concentrations | 5576 | ||
10.9.4.4. Abrupt Carbon-cycle Change | 5578 | ||
10.9.5. The Precambrian Record of Carbon-Cycle Change | 5579 | ||
10.9.6. Conclusions | 5580 | ||
Acknowledgments | 5580 | ||
References | 5580 | ||
Chapter 10.10: The Contemporary Carbon Cycle | 5588 | ||
10.10.1. Introduction | 5589 | ||
10.10.2. Major Reservoirs and Natural Fluxes of Carbon | 5589 | ||
10.10.2.1. Reservoirs | 5589 | ||
10.10.2.1.1. The atmosphere | 5589 | ||
10.10.2.1.2. Terrestrial ecosystems: vegetation and soils | 5590 | ||
10.10.2.1.3. The oceans | 5591 | ||
10.10.2.1.4. Fossil fuels | 5591 | ||
10.10.2.2. The Natural Flows of Carbon | 5592 | ||
10.10.2.2.1. Between land and atmosphere | 5592 | ||
10.10.2.2.2. Between oceans and atmosphere | 5594 | ||
10.10.2.2.3. Between land and oceans | 5594 | ||
10.10.3. Changes in the Stocks and Fluxes of Carbon as a Result of Human Activities | 5595 | ||
10.10.3.1. Changes over the Period 1850-2008 | 5595 | ||
10.10.3.1.1. Emissions of carbon from combustion of fossil fuels | 5595 | ||
10.10.3.1.2. The increase in atmospheric carbon dioxide | 5596 | ||
10.10.3.1.3. Net uptake of carbon by the oceans | 5597 | ||
10.10.3.1.3.1. Ocean carbon models | 5598 | ||
10.10.3.1.4. Land: net exchange of carbon between terrestrial ecosystems and the atmosphere | 5598 | ||
10.10.3.1.5. Land: changes in land use | 5598 | ||
10.10.3.1.6. Land: the residual carbon sink | 5601 | ||
10.10.3.2. Changes over the Period 1980-2008 | 5602 | ||
10.10.3.2.1. The global carbon budget | 5602 | ||
10.10.3.2.1.1. Sources and sinks inferred from inverse modeling with atmospheric transport models and atmospheric concentrat... | 5602 | ||
10.10.3.2.1.2. Terrestrial sources and sinks of carbon from land-use change | 5604 | ||
10.10.3.2.2. Regional distribution of sources and sinks of carbon: the northern mid-latitudes | 5604 | ||
10.10.3.2.2.1. Inferring changes in terrestrial carbon storage from analysis of forest inventories | 5605 | ||
10.10.3.2.3. Regional distribution of sources and sinks of carbon: the tropics | 5606 | ||
10.10.3.2.3.1. Forest inventories | 5606 | ||
10.10.3.2.3.2. Direct measurement of CO2 flux | 5607 | ||
10.10.3.2.4. Summary: synthesis of the results of different methods | 5607 | ||
10.10.4. Mechanisms Thought to be Responsible for Current Terrestrial Carbon Sink | 5608 | ||
10.10.4.1. Physiological or Metabolic Responses to Environmental Change | 5609 | ||
10.10.4.1.1. CO2 fertilization | 5609 | ||
10.10.4.1.2. Nitrogen fertilization | 5610 | ||
10.10.4.1.3. Atmospheric chemistry | 5610 | ||
10.10.4.1.4. Climatic variability and climatic change | 5611 | ||
10.10.4.1.5. Synergies among physiological `mechanisms´ | 5612 | ||
10.10.4.1.6. Terrestrial carbon models | 5612 | ||
10.10.4.2. Structural, Demographic, and Disturbance Mechanisms | 5613 | ||
10.10.4.3. Which Terrestrial Mechanisms Are Most Important? | 5613 | ||
10.10.4.4. Other Possible Explanations for the Residual Terrestrial Carbon Sink | 5613 | ||
10.10.4.4.1. Aquatic transport: erosion and redeposition of carbon | 5614 | ||
10.10.4.4.2. Woody encroachment | 5614 | ||
10.10.4.4.3. Emissions from draining and burning of peatlands | 5614 | ||
10.10.5. The Future | 5614 | ||
10.10.5.1. Natural Feedbacks | 5614 | ||
10.10.5.1.1. The airborne fraction | 5615 | ||
10.10.5.1.2. How will the magnitude of the current terrestrial sink change in the future? | 5615 | ||
10.10.5.1.3. How will the oceanic uptake of carbon change in the future? | 5616 | ||
10.10.5.1.3.1. Physical and chemical mechanisms | 5616 | ||
10.10.5.1.3.1.1. The buffer factor | 5616 | ||
10.10.5.1.3.1.2. Warming | 5616 | ||
10.10.5.1.3.1.3. Vertical mixing and stratification | 5617 | ||
10.10.5.1.3.1.4. Rate of CO2 emissions | 5617 | ||
10.10.5.1.3.2. Biological feedback/processes | 5617 | ||
10.10.5.1.3.2.1. Addition of nutrients limiting primary production | 5617 | ||
10.10.5.1.3.2.2. Enhanced utilization of nutrients | 5617 | ||
10.10.5.1.3.2.3. Changes in the elemental ratios of organic matter in the ocean | 5617 | ||
10.10.5.1.3.2.4. Increases in the organic carbon/carbonate ratio of export production | 5617 | ||
10.10.5.2. Deliberate Sequestering of Carbon (or Reduction of Emissions) | 5618 | ||
10.10.5.2.1. Terrestrial | 5618 | ||
10.10.5.2.2. Oceanic | 5618 | ||
10.10.5.2.3. Geologic | 5618 | ||
10.10.6. Conclusion | 5618 | ||
References | 5619 | ||
Chapter 10.11: The Global Oxygen Cycle | 5626 | ||
10.11.1. Introduction | 5628 | ||
10.11.2. Distribution of O2 among Earth Surface Reservoirs | 5628 | ||
10.11.2.1. The Atmosphere | 5628 | ||
10.11.2.2. The Oceans | 5628 | ||
10.11.2.3. Freshwater Environments | 5631 | ||
10.11.2.4. Soils and Groundwaters | 5631 | ||
10.11.3. Mechanisms of O2 Production | 5632 | ||
10.11.3.1. Photosynthesis | 5632 | ||
10.11.3.2. Photolysis of Water | 5633 | ||
10.11.4. Mechanisms of O2 Consumption | 5634 | ||
10.11.4.1. Aerobic Respiration | 5634 | ||
10.11.4.2. Photorespiration | 5634 | ||
10.11.4.3. C1 Metabolism | 5634 | ||
10.11.4.4. Inorganic Metabolism | 5635 | ||
10.11.4.5. Macroscale Patterns of Aerobic Respiration | 5635 | ||
10.11.4.6. Volcanic Gases | 5636 | ||
10.11.4.7. Mineral Oxidation | 5636 | ||
10.11.4.8. Hydrothermal Vents | 5636 | ||
10.11.4.9. Fe and S Oxidation at the Oxic&ndas | 5636 | ||
10.11.4.10. Abiotic Organic Matter Oxidati | 5637 | ||
10.11.5. Global O2 Budgets | 5637 | ||
10.11.6. Atmospheric O2 Throughout Earth History | 5637 | ||
10.11.6.1. Early Models | 5637 | ||
10.11.6.2. The Archean | 5639 | ||
10.11.6.2.1. Constraints on the O2 content of the Archean atmosphere | 5639 | ||
10.11.6.2.2. The evolution of oxygenic photosynthesis | 5640 | ||
10.11.6.2.3. Carbon isotope effects associated with photosynthesis | 5642 | ||
10.11.6.2.4. Evidence for oxygenic photosynthesis in the late Archean | 5643 | ||
10.11.6.3. The Proterozoic Atmosphere | 5643 | ||
10.11.6.3.1. The Great Oxidation Event | 5643 | ||
10.11.6.3.2. Survival strategies on the earliest oxygenated Earth | 5646 | ||
10.11.6.3.3. Atmospheric O2 during the Mesoproterozoic | 5646 | ||
10.11.6.3.4. Neoproterozoic atmospheric O2 | 5647 | ||
10.11.6.4. Phanerozoic Atmospheric O2 | 5649 | ||
10.11.6.4.1. Constraints on Phanerozoic O2 variation | 5649 | ||
10.11.6.4.2. Evidence for variations in Phanerozoic O2 | 5649 | ||
10.11.6.4.3. Numerical models of Phanerozoic atmospheric O2 | 5651 | ||
10.11.7. Conclusions | 5658 | ||
References | 5659 | ||
Chapter 10.12: The Global Nitrogen Cycle | 5664 | ||
10.12.1. Introduction | 5664 | ||
10.12.2. Biogeochemical Reactions | 5666 | ||
10.12.2.1. The Initial Reaction: Nr Creation | 5666 | ||
10.12.2.2. Atmosphere | 5666 | ||
10.12.2.2.1. Inorganic reduced N | 5667 | ||
10.12.2.2.2. Inorganic oxidized N | 5667 | ||
10.12.2.2.3. Reduced organic N | 5668 | ||
10.12.2.2.4. Oxidized organic N | 5668 | ||
10.12.2.3. Biosphere | 5668 | ||
10.12.2.3.1. Nitrogen fixation | 5668 | ||
10.12.2.3.2. Ammonia assimilation | 5668 | ||
10.12.2.3.3. Nitrification | 5668 | ||
10.12.2.3.4. Assimilatory nitrate reduction | 5668 | ||
10.12.2.3.5. Ammonification | 5668 | ||
10.12.2.3.6. Denitrification | 5668 | ||
10.12.2.3.7. Anammox | 5669 | ||
10.12.3. N Reservoirs and Their Exchanges | 5669 | ||
10.12.3.1. Land to Atmosphere | 5669 | ||
10.12.3.2. Ocean to Atmosphere | 5669 | ||
10.12.3.3. Atmosphere to Surface | 5669 | ||
10.12.3.4. Land to Ocean | 5669 | ||
10.12.3.5. Continent to Continent - Commerce | 5669 | ||
10.12.4. Nr Creation | 5669 | ||
10.12.4.1. Introduction | 5669 | ||
10.12.4.2. Lightning | 5669 | ||
10.12.4.3. Terrestrial BNF | 5670 | ||
10.12.4.4. Anthropogenic | 5670 | ||
10.12.4.4.1. Introduction | 5670 | ||
10.12.4.4.2. Food production | 5671 | ||
10.12.4.4.3. Energy production | 5672 | ||
10.12.4.5. Anthropogenic Nr Creation Rates from 1860 to 2005 | 5672 | ||
10.12.5. Global Terrestrial N Budgets | 5672 | ||
10.12.5.1. Introduction | 5672 | ||
10.12.5.2. Nr Creation | 5674 | ||
10.12.5.3. Nr Distribution | 5674 | ||
10.12.5.4. Nr Conversion to N2 | 5675 | ||
10.12.6. Global Marine N Budget | 5676 | ||
10.12.7. Regional N Budgets | 5678 | ||
10.12.8. Consequences | 5679 | ||
10.12.8.1. Introduction | 5679 | ||
10.12.8.2. Atmosphere | 5680 | ||
10.12.8.3. Terrestrial Ecosystems | 5680 | ||
10.12.8.4. Aquatic Ecosystems | 5681 | ||
10.12.9. Future | 5682 | ||
10.12.10. Societal Responses | 5682 | ||
10.12.11. Summary | 5685 | ||
Acknowledgments | 5685 | ||
References | 5685 | ||
Chapter 10.13: The Global Phosphorus Cycle | 5688 | ||
10.13.1. Introduction | 5688 | ||
10.13.2. The Global Phosphorus Cycle: Overview | 5689 | ||
10.13.2.1. The Terrestrial Phosphorus Cycle | 5689 | ||
10.13.2.2. Transport of Phosphorus from Continents to the Ocean | 5692 | ||
10.13.2.2.1. Human impacts on the global phosphorus cycle | 5693 | ||
10.13.2.3. The Marine Phosphorus Cycle | 5693 | ||
10.13.3. Phosphorus Biogeochemistry and Cycling: Current Research | 5694 | ||
10.13.3.1. Phosphorus Cycling in Terrestrial Ecosystems and Soils | 5694 | ||
10.13.3.2. Phosphorus Cycling in Terrestrial Aquatic Systems: Lakes, Rivers, and Estuaries | 5695 | ||
10.13.3.2.1. Biogeochemistry and cycling of phosphorus in lakes | 5695 | ||
10.13.3.2.2. Biogeochemistry and cycling of phosphorus in rivers and estuaries | 5696 | ||
10.13.3.2.3. Submarine groundwater discharge of phosphorus to the ocean | 5698 | ||
10.13.3.3. Biogeochemistry and Cycling of Phosphorus in the Modern Ocean | 5699 | ||
10.13.3.3.1. Phosphorus in the oceanic water column: Composition and cycling | 5699 | ||
10.13.3.3.1.1. Dissolved inorganic phosphorus | 5699 | ||
10.13.3.3.1.2. Dissolved organic phosphorus | 5700 | ||
10.13.3.3.1.3. Water column particulate P | 5704 | ||
10.13.3.3.1.3.1. Water column C:P ratios | 5704 | ||
10.13.3.3.1.3.2. Application of 31P-NMR to water column particulate matter | 5704 | ||
10.13.3.3.1.3.3. Cosmogenic 32P and 33P as tracers of phosphorus cycling in surface waters | 5705 | ||
10.13.3.3.1.4. Oxygen isotopes of phosphate in seawater | 5706 | ||
10.13.3.3.1.5. Phosphorus limitation of marine primary photosynthetic production | 5707 | ||
10.13.3.3.2. Phosphorus burial and the marine phosphorus budget | 5710 | ||
10.13.3.3.2.1. Historical perspective | 5710 | ||
10.13.3.3.2.2. Diagenesis and burial of phosphorus in marine sediments | 5711 | ||
10.13.3.3.2.2.1. Sedimentary organic phosphorus: composition and reactivity | 5714 | ||
10.13.3.3.2.2.2. Authigenic CFA: modern phosphorites | 5715 | ||
10.13.3.3.2.2.3. Disseminated authigenic CFA | 5717 | ||
10.13.3.3.2.2.4. Experimental studies of authigenic apatite precipitation | 5723 | ||
10.13.3.3.2.2.5. Other authigenic phosphate minerals | 5724 | ||
10.13.3.3.2.2.6. Sedimentary organic carbon to organic phosphorus [(C:P)org] ratios | 5725 | ||
10.13.3.3.2.2.7. Coupled iron-phosphorus cycling | 5729 | ||
10.13.3.3.3. Benthic return flux of phosphorus from the seabed | 5731 | ||
10.13.3.3.4. The oceanic residence time of phosphorus | 5732 | ||
10.13.3.4. Phosphorus Cycling Over Long, Geologic Timescales | 5733 | ||
10.13.3.4.1. The role of tectonics in the global phosphorus cycle | 5733 | ||
10.13.3.4.2. Links to other biogeochemical cycles on long, geologic timescales | 5733 | ||
10.13.3.4.2.1. The nutrient-CO2 connection | 5733 | ||
10.13.3.4.2.2. The phosphorus-iron-o& | 5734 | ||
10.13.3.4.3. Phosphorus in paleoceanography: P burial as a proxy for weathering, paleoproductivity, and climate change | 5735 | ||
10.13.3.4.4. Ancient phosphorites | 5736 | ||
10.13.4. Summary | 5736 | ||
References | 5736 | ||
Chapter 10.14: The Global Sulfur Cycle | 5748 | ||
10.14.1. Elementary Issues | 5749 | ||
10.14.1.1. History | 5749 | ||
10.14.1.2. Isotopes | 5749 | ||
10.14.1.3. Allotropes | 5750 | ||
10.14.1.4. Vapor Pressure | 5750 | ||
10.14.1.5. Chemistry | 5750 | ||
10.14.2. Abundance of Sulfur and Early History | 5752 | ||
10.14.2.1. Sulfur in the Cosmos | 5753 | ||
10.14.2.2. Condensation, Accretion, and Evolution | 5753 | ||
10.14.2.3. Sulfur on the Early Earth | 5754 | ||
10.14.3. Occurrence of Sulfur | 5755 | ||
10.14.3.1. Elemental Sulfur | 5755 | ||
10.14.3.2. Sulfides | 5755 | ||
10.14.3.3. Evaporites | 5755 | ||
10.14.3.4. The Geological History of Sulfur | 5755 | ||
10.14.3.5. Utilization and Extraction of Sulfur Minerals | 5756 | ||
10.14.4. Chemistry of Volcanogenic Sulfur | 5757 | ||
10.14.4.1. Deep-Sea Vents | 5757 | ||
10.14.4.1.1. Chemistry of volcanogenic sulfur | 5757 | ||
10.14.4.2. Aerial Emissions | 5757 | ||
10.14.4.3. Fumaroles | 5758 | ||
10.14.4.4. Crater Lakes | 5758 | ||
10.14.4.5. Impacts of Emissions on Local Environments | 5758 | ||
10.14.5. Biochemistry of Sulfur | 5759 | ||
10.14.5.1. Origin of Life | 5759 | ||
10.14.5.2. Sulfur Biomolecules | 5759 | ||
10.14.5.3. Uptake of Sulfur | 5760 | ||
10.14.6. Sulfur in Seawater | 5760 | ||
10.14.6.1. Sulfate | 5760 | ||
10.14.6.2. Hydrogen Sulfide | 5761 | ||
10.14.6.3. OCS and Carbon Disulfide | 5761 | ||
10.14.6.4. Organosulfides | 5761 | ||
10.14.6.5. Coastal Marshes | 5763 | ||
10.14.7. Surface and Groundwaters | 5763 | ||
10.14.8. Marine Sediments | 5764 | ||
10.14.9. Soils and Vegetation | 5765 | ||
10.14.10. Troposphere | 5766 | ||
10.14.10.1. Atmospheric Budget of Sulfur Compounds | 5766 | ||
10.14.10.2. Hydrogen Sulfide | 5767 | ||
10.14.10.3. Carbonyl Sulfide | 5767 | ||
10.14.10.4. Carbon Disulfide | 5768 | ||
10.14.10.5. Dimethyl Sulfide | 5768 | ||
10.14.10.6. DMSO and Methanesulfonic Acid | 5769 | ||
10.14.10.7. Sulfur | 5769 | ||
10.14.10.8. Sulfur Dioxide | 5769 | ||
10.14.10.9. Aerosol Sulfates and Climate | 5771 | ||
10.14.10.10. Deposition | 5771 | ||
10.14.11. Anthropogenic Impacts on the Sulfur Cycle | 5772 | ||
10.14.11.1. Combustion Emissions | 5772 | ||
10.14.11.2. Organosulfur Gases | 5772 | ||
10.14.11.3. Acid Rain | 5773 | ||
10.14.11.4. Water and Soil Pollutants | 5773 | ||
10.14.11.5. Coastal Pollution | 5774 | ||
10.14.12. Sulfur in Upper Atmospheres | 5774 | ||
10.14.12.1. Radiation Balance and Sulfate Particles | 5774 | ||
10.14.12.2. Ozone | 5775 | ||
10.14.12.3. Aircraft | 5775 | ||
10.14.13. Planets and Moons | 5776 | ||
10.14.13.1. Venus | 5776 | ||
10.14.13.2. Jupiter | 5776 | ||
10.14.13.3. Io | 5776 | ||
10.14.13.4. Europa | 5776 | ||
10.14.14. Conclusions | 5777 | ||
References | 5777 | ||
Chapter 10.15: Plankton Respiration, Net Community Production and the Organic Carbon Cycle in the Oceanic Water Column | 5782 | ||
10.15.1. Introduction | 5782 | ||
10.15.2. Biogeochemical Background | 5783 | ||
10.15.3. Biochemical Background | 5784 | ||
10.15.4. Measurement of Respiration Rates | 5785 | ||
10.15.4.1. Measurement of the Rate of Concentration Change of a Reactant or Product | 5785 | ||
10.15.4.2. Measurements of the ETS | 5785 | ||
10.15.4.3. Other Biological Approaches | 5786 | ||
10.15.5. First Order Overall Global Organic Budget of the Oceans | 5786 | ||
10.15.6. Distribution of Respiration within the Oceans | 5787 | ||
10.15.6.1. Respiration in the Epipelagic Zone | 5788 | ||
10.15.6.2. Assessment of the Output of Organic Material from the Euphotic Zone | 5793 | ||
10.15.6.3. Consumption of Organic Material Within the Dark Zone of the Ocean | 5794 | ||
10.15.7. Distribution of Respiration within the Community | 5797 | ||
10.15.8. Summary | 5799 | ||
References | 5800 | ||
Chapter 10.16: Respiration in Terrestrial Ecosystems | 5802 | ||
10.16.1. Introduction | 5804 | ||
10.16.1.1. Photosynthesis and Respiration | 5805 | ||
10.16.1.2. A Definition of Respiration | 5805 | ||
10.16.1.3. Objectives and Outline | 5806 | ||
10.16.2. Cellular Respiration | 5806 | ||
10.16.2.1. Carbon Metabolism in Respiration | 5806 | ||
10.16.2.1.1. Glycolysis | 5806 | ||
10.16.2.1.2. Tricarboxylic acid cycle | 5807 | ||
10.16.2.2. The Mitochondrial Electron Transport Chain and Oxidative Phosphorylation | 5807 | ||
10.16.2.3. Daytime Foliar Respiration | 5809 | ||
10.16.2.4. Fermentation | 5809 | ||
10.16.2.5. Alternative Microbial Terminal Electron Acceptors | 5809 | ||
10.16.2.5.1. Nitrate and sulfate reduction | 5809 | ||
10.16.2.5.2. Methanogenesis | 5810 | ||
10.16.2.5.3. Methane oxidation | 5810 | ||
10.16.3. Whole-Plant Respiration | 5810 | ||
10.16.3.1. Sinks for Respiratory Energy: Growth, Maintenance, and Transport | 5810 | ||
10.16.3.2. Nonphosphorylating Respiration | 5811 | ||
10.16.3.3. Contribution of the Alternative, Nonphosphorylating Pathway to Respiration in Roots and Leaves | 5812 | ||
10.16.3.4. Effects of Some Environmental Conditions on Plant Respiration | 5813 | ||
10.16.3.5. Plant Respiration and Photosynthesis | 5815 | ||
10.16.3.5.1. The RA/GPP of plants and in terrestrial ecosystems | 5815 | ||
10.16.3.5.2. Environmental effects on plant RA/GPP | 5815 | ||
10.16.3.5.3. Forest RA/GPP during stand development | 5818 | ||
10.16.4. Animal Respiration | 5819 | ||
10.16.4.1. Respiration of Individual Animals | 5819 | ||
10.16.4.2. Animal Respiration in Terrestrial Ecosystems | 5819 | ||
10.16.4.3. Respiration by Humans and Domestic Farm Animals | 5820 | ||
10.16.4.4. Methane Production by Terrestrial Animals | 5821 | ||
10.16.4.4.1. Methane production by wild vertebrates | 5821 | ||
10.16.4.4.2. Methane production by termites and other terrestrial arthropods | 5821 | ||
10.16.4.4.3. Methane production by domestic animals | 5821 | ||
10.16.5. Respiration of Terrestrial Ecosystems | 5822 | ||
10.16.5.1. Soil Respiration | 5822 | ||
10.16.5.1.1. Soil CO2 efflux and soil respiration | 5822 | ||
10.16.5.1.2. Temporal variations in soil CO2 efflux | 5823 | ||
10.16.5.1.3. Annual soil respiration within terrestrial ecosystems | 5823 | ||
10.16.5.1.4. Sources of soil-derived CO2 | 5824 | ||
10.16.5.1.4.1. Decomposer contributions to soil respiration | 5825 | ||
10.16.5.1.4.2. Root contributions to soil respiration | 5825 | ||
10.16.5.1.4.3. Soil methane oxidation | 5826 | ||
10.16.5.1.4.4. Soil denitrification | 5826 | ||
10.16.5.1.4.5. Abiotic CO2 production within soils | 5827 | ||
10.16.5.1.5. Effects of environmental factors on microbial and soil respiration | 5828 | ||
10.16.5.1.5.1. Soil organic carbon and climate change | 5828 | ||
10.16.5.1.5.2. Temperature and soil carbon mineralization | 5828 | ||
10.16.5.1.5.3. Soil moisture and soil carbon mineralization | 5829 | ||
10.16.5.2. Net Ecosystem Exchange of Carbon Dioxide | 5829 | ||
10.16.6. Global Terrestrial Ecosystem Respiration | 5830 | ||
References | 5831 | ||
e9780080983004v11 | 5839 | ||
Front Cover | 5839 | ||
Environmental Geochemistry | 5842 | ||
Copyright | 5843 | ||
In Memoriam | 5844 | ||
Heinrich Dieter Holland (1927–2012) | 5846 | ||
Karl Karekin Turekian (1927–2013) | 5848 | ||
References | 5850 | ||
Dedication | 5852 | ||
Contents | 5854 | ||
Executive editors’ Foreword to the Second Edition | 5856 | ||
Contributors | 5860 | ||
Volume editor’s Introduction | 5862 | ||
References | 5863 | ||
Chapter 11.1: Groundwater and Air Contamination: Risk, Toxicity, Exposure Assessment, Policy, and Regulation | 5864 | ||
11.1.1. Introduction | 5864 | ||
11.1.2. Principles, Definitions, and Perspectives of Hazardous Waste Risk Assessments | 5865 | ||
11.1.2.1. Definitions of Hazard and Risk | 5865 | ||
11.1.2.2. Typical Risks Encountered – Natural and Anthropogenic | 5865 | ||
11.1.2.3. Risks Associated with Contaminated Sites and Groundwater | 5865 | ||
11.1.3. Regulatory and Policy Basis for Risk Assessment | 5865 | ||
11.1.3.1. Examples of Contaminated Sites and Potential Risk Exposure Pathways | 5865 | ||
11.1.3.2. Risk-Based Nature of CERCLA | 5866 | ||
11.1.3.3. Risk-Based Corrective Actions | 5866 | ||
11.1.3.4. Use of Applicable or Relevant and Appropriate Requirements | 5867 | ||
11.1.3.5. Limited Uses of Absolute Standards | 5867 | ||
11.1.4. The Risk Assessment Process | 5867 | ||
11.1.4.1. Sources, Pathways, and Receptors: The Fundamental Algorithm for Risk Assessments | 5867 | ||
11.1.4.2. The Four-Step Risk Assessment Process | 5868 | ||
11.1.5. Hazard Identification | 5868 | ||
11.1.5.1. Determining Contaminant Identity, Concentration, and Distribution | 5868 | ||
11.1.5.2. Contaminant Surrogate Analysis | 5868 | ||
11.1.6. Exposure Assessment | 5869 | ||
11.1.6.1. Potential Exposure Pathways | 5869 | ||
11.1.6.2. Estimating Exposure Concentrations | 5869 | ||
11.1.6.3. Identifying Potentially Exposed Populations | 5869 | ||
11.1.6.4. Estimating Chemical Intake | 5869 | ||
11.1.7. Toxicity Assessment | 5870 | ||
11.1.7.1. Overview of Human Health Toxicology | 5870 | ||
11.1.7.1.1. Classification of toxic responses | 5871 | ||
11.1.7.1.2. Quantifying reversible toxic effects | 5871 | ||
11.1.7.2. Quantifying Noncarcinogenic Risk: Reference Dosages | 5871 | ||
11.1.7.2.1. The no observed adverse effect level | 5871 | ||
11.1.7.2.2. Acceptable daily intakes and reference doses | 5871 | ||
11.1.7.3. Quantifying Carcinogenic Risk: Slope Factors | 5872 | ||
11.1.8. Risk Characterization | 5873 | ||
11.1.8.1. Determination of Noncarcinogenic Risk | 5873 | ||
11.1.8.2. Determination of Carcinogenic Risk | 5873 | ||
11.1.9. Sources of Uncertainties in Risk Assessment | 5873 | ||
11.1.9.1. Source Characterization | 5873 | ||
11.1.9.2. Lack of Available Data | 5874 | ||
11.1.9.3. Exposure Assessment Models and Methods | 5874 | ||
11.1.9.4. Quality of Toxicological Data | 5874 | ||
11.1.9.5. Evaluating Uncertainty | 5874 | ||
11.1.10. Risk Management and Risk Communication | 5874 | ||
References | 5875 | ||
Chapter 11.2: Arsenic and Selenium | 5876 | ||
11.2.1. Introduction | 5877 | ||
11.2.2. Sampling | 5879 | ||
11.2.2.1. Rocks, Soils, and Sediments | 5879 | ||
11.2.2.2. Water | 5879 | ||
11.2.2.2.1. Techniques | 5879 | ||
11.2.2.2.2. Filtered or unfiltered samples | 5880 | ||
11.2.2.2.3. Sample preservation and redox stability | 5880 | ||
11.2.3. Analytical Methods | 5881 | ||
11.2.3.1. Arsenic | 5881 | ||
11.2.3.1.1. Total arsenic in aqueous samples | 5881 | ||
11.2.3.1.1.1. Laboratory methods | 5881 | ||
11.2.3.1.1.2. Field-test kits | 5882 | ||
11.2.3.1.2. Total arsenic in solid samples | 5882 | ||
11.2.3.1.3. Arsenic speciation | 5882 | ||
11.2.3.1.3.1. Aqueous speciation | 5882 | ||
11.2.3.1.3.2. Solid-phase speciation | 5882 | ||
11.2.3.1.3.3. EPA TCLP test | 5883 | ||
11.2.3.2. Selenium | 5883 | ||
11.2.3.2.1. Total selenium in aqueous samples | 5883 | ||
11.2.3.2.1.1. Pretreatment to destroy organic matter | 5883 | ||
11.2.3.2.1.2. Laboratory methods | 5883 | ||
11.2.3.2.2. Selenium in solid samples | 5884 | ||
11.2.3.2.3. Selenium speciation | 5884 | ||
11.2.3.3. Quality Control and Standard Reference Materials | 5884 | ||
11.2.4. Abundance and Forms of Arsenic in the Natural Environment | 5885 | ||
11.2.4.1. Abundance in Rocks, Soils, and Sediments | 5885 | ||
11.2.4.2. National and International Standards for Drinking Water | 5885 | ||
11.2.4.3. Abundance and Distribution in Natural Waters | 5886 | ||
11.2.4.3.1. Atmospheric precipitation | 5886 | ||
11.2.4.3.2. River water | 5887 | ||
11.2.4.3.3. Lake water | 5887 | ||
11.2.4.3.4. Seawater and estuaries | 5888 | ||
11.2.4.3.5. Groundwater | 5888 | ||
11.2.4.3.6. Sediment pore water | 5889 | ||
11.2.4.3.7. Acid mine drainage | 5889 | ||
11.2.4.4. Arsenic Species in Natural Waters | 5890 | ||
11.2.4.4.1. Inorganic species | 5890 | ||
11.2.4.4.2. Organic species | 5891 | ||
11.2.4.4.3. Observed speciation in different water types | 5891 | ||
11.2.4.5. Microbial Controls | 5892 | ||
11.2.5. Pathways and Behavior of Arsenic in the Natural Environment | 5892 | ||
11.2.5.1. Release from Primary Minerals | 5893 | ||
11.2.5.1.1. Examples of mining-related arsenic problems | 5893 | ||
11.2.5.1.2. Modern practice in mine-waste stabilization | 5894 | ||
11.2.5.2. Role of Secondary Minerals | 5894 | ||
11.2.5.2.1. The importance of arsenic cycling and diagenesis | 5894 | ||
11.2.5.2.2. Redox behavior | 5894 | ||
11.2.5.3. Adsorption of Arsenic by Oxides and Clays | 5895 | ||
11.2.5.4. Arsenic Transport | 5896 | ||
11.2.5.5. Impact of Changing Environmental Conditions | 5896 | ||
11.2.5.5.1. Release of arsenic at high pH | 5896 | ||
11.2.5.5.2. Release of arsenic on reduction | 5896 | ||
11.2.5.6. Case Studies | 5896 | ||
11.2.5.6.1. The Bengal Basin, Bangladesh, and India | 5896 | ||
11.2.5.6.2. Chaco-Pampean Plain, Argentina | 5898 | ||
11.2.5.6.3. Eastern Wisconsin, USA | 5900 | ||
11.2.6. Abundance and Forms of Selenium in the Natural Environment | 5901 | ||
11.2.6.1. Abundance in Rocks, Soils, and Sediments | 5901 | ||
11.2.6.2. National and International Standards in Drinking Water | 5903 | ||
11.2.6.3. Abundance and Distribution in Natural Waters | 5903 | ||
11.2.6.3.1. Atmospheric precipitation | 5904 | ||
11.2.6.3.2. River and lake water | 5904 | ||
11.2.6.3.3. Seawater and estuaries | 5904 | ||
11.2.6.3.4. Groundwater | 5904 | ||
11.2.6.3.5. Sediment pore water | 5905 | ||
11.2.6.3.6. Mine drainage | 5905 | ||
11.2.6.4. Selenium Species in Water, Sediment, and Soil | 5905 | ||
11.2.7. Pathways and Behavior of Selenium in the Natural Environment | 5906 | ||
11.2.7.1. Release from Primary Minerals | 5906 | ||
11.2.7.2. Adsorption of Selenium by Oxides and Clays | 5906 | ||
11.2.7.3. Selenium Transport | 5907 | ||
11.2.7.3.1. Global fluxes | 5907 | ||
11.2.7.3.2. Selenium fluxes in air | 5907 | ||
11.2.7.3.3. Soil–water–plant relationships | 5908 | ||
11.2.7.4. Case Studies | 5909 | ||
11.2.7.4.1. Kesterson Reservoir, USA | 5909 | ||
11.2.7.4.2. Enshi, China | 5909 | ||
11.2.7.4.3. Soan-Sakesar Valley, Pakistan | 5910 | ||
11.2.7.4.4. Selenium deficiency, China | 5911 | ||
11.2.8. Concluding Remarks | 5911 | ||
Acknowledgments | 5912 | ||
References | 5912 | ||
Chapter 11.3: Heavy Metals in the Environment – Historical Trends | 5922 | ||
11.3.1. Introduction | 5922 | ||
11.3.1.1. Metals: Pb, Zn, Cd, Cr, Cu, Ni | 5922 | ||
11.3.1.2. Sources of Metals | 5923 | ||
11.3.1.2.1. Natural | 5923 | ||
11.3.1.2.2. Anthropogenic | 5923 | ||
11.3.1.3. Source and Pathways | 5924 | ||
11.3.2. Occurrence, Speciation, and Phase Associations | 5924 | ||
11.3.2.1. Geochemical Properties and Major Solute Species | 5924 | ||
11.3.2.1.1. Lead | 5924 | ||
11.3.2.1.2. Zinc | 5926 | ||
11.3.2.1.3. Cadmium | 5926 | ||
11.3.2.1.4. Chromium | 5927 | ||
11.3.2.1.5. Copper | 5927 | ||
11.3.2.1.6. Nickel | 5928 | ||
11.3.2.2. Occurrence in Rocks, Soils, Sediments,Anthropogenic Materials | 5928 | ||
11.3.2.3. Geochemical Phase Associations in Soils and Sediments | 5929 | ||
11.3.3. Atmospheric Emissions of Metals and Geochemical Cycles | 5932 | ||
11.3.3.1. Historical Heavy Metal Fluxes to the Atmosphere | 5933 | ||
11.3.3.2. Perturbed Heavy Metal Cycles | 5934 | ||
11.3.3.3. Global Emissions of Heavy Metals | 5934 | ||
11.3.3.4. US Emissions of Heavy Metals | 5935 | ||
11.3.3.4.1. Lead | 5935 | ||
11.3.3.4.2. Zinc | 5936 | ||
11.3.3.4.3. Cadmium | 5936 | ||
11.3.4. Historical Metal Trends Reconstructed from Sediment Cores | 5937 | ||
11.3.4.1. Paleolimnological Approach | 5937 | ||
11.3.4.2. Age Dating | 5940 | ||
11.3.4.3. Selected Reconstructed Metal Trends | 5940 | ||
11.3.4.3.1. Lead and leaded gasoline: consequence of the clean air act | 5940 | ||
11.3.4.3.2. Zinc from rubber tire wear | 5942 | ||
11.3.4.3.3. Metal processing and metal trends in sediment cores | 5943 | ||
11.3.4.3.4. Reduction in power plant emissions of heavy metals: clean air act amendments and the use of low sulfur coal | 5944 | ||
11.3.4.3.5. European lacustrine records of heavy metal pollution | 5946 | ||
References | 5949 | ||
Chapter 11.4: Geochemistry of Mercury in the Environment | 5954 | ||
11.4.1. Introduction | 5954 | ||
11.4.1.1. The Global Mercury Cycle | 5956 | ||
11.4.2. Fundamental Geochemistry | 5958 | ||
11.4.2.1. Solid Earth Abundance and Distribution | 5958 | ||
11.4.2.2. Isotopic Distributions | 5959 | ||
11.4.2.3. Minable Deposits | 5959 | ||
11.4.2.4. Occurrence of Mercury in Fossil Fuels | 5959 | ||
11.4.3. Sources of Mercury to the Environment | 5960 | ||
11.4.3.1. Volcanic Mercury Emissions | 5960 | ||
11.4.3.2. Mercury Input to the Oceans via Submarine Volcanism | 5963 | ||
11.4.3.3. Low-Temperature Volatilization | 5963 | ||
11.4.3.4. Anthropogenic Sources | 5964 | ||
11.4.3.5. Mining | 5965 | ||
11.4.3.6. Biomass Burning, Soil and Oceanic Evasion – Mixed Sources | 5965 | ||
11.4.3.7. Watersheds and Legacy Mercury | 5966 | ||
11.4.4. Atmospheric Cycling and Chemistry of Mercury | 5966 | ||
11.4.5. Aquatic Biogeochemistry of Mercury | 5970 | ||
11.4.5.1. Environmental Mercury Methylation | 5972 | ||
11.4.5.1.1. Nearshore regions | 5973 | ||
11.4.5.1.2. Open-ocean mercury cycling | 5974 | ||
11.4.5.1.3. Open-ocean mercury profiles | 5975 | ||
11.4.6. Removal of Mercury from the Surficial Cycle | 5977 | ||
11.4.7. Models of the Global Cycle | 5979 | ||
11.4.8. Developments in Studying Mercury in the Environment on a Variety of Scales | 5981 | ||
11.4.8.1. Acid Rain and Mercury Synergy in Lakes | 5981 | ||
11.4.8.2. METAALICUS | 5981 | ||
11.4.8.3. Fractionation of Mercury Isotopes | 5981 | ||
11.4.8.4. Tracing Atmospheric Mercury with 210Pb and Br | 5982 | ||
11.4.8.5. Mercury and Organic Matter Interactions | 5983 | ||
11.4.9. Summary | 5983 | ||
Acknowledgments | 5983 | ||
References | 5983 | ||
Chapter 11.5: The Geochemistry of Acid Mine Drainage | 5994 | ||
11.5.1. Introduction | 5995 | ||
11.5.1.1. Scale of the Problem | 5995 | ||
11.5.1.2. Overview of the Mining Process and Sources of Low-Quality Drainage | 5995 | ||
11.5.1.3. Sources of Low-Quality Drainage | 5995 | ||
11.5.1.3.1. Mine workings | 5995 | ||
11.5.1.3.2. Open-pits | 5996 | ||
11.5.1.3.3. Waste rock | 5996 | ||
11.5.1.3.4. Mill tailings | 5996 | ||
11.5.1.3.5. Wastes from extractive metallurgy operations | 5996 | ||
11.5.2. Mineralogy of Ore Deposits | 5997 | ||
11.5.2.1. Coal Deposits | 5997 | ||
11.5.2.2. Base-Metal Deposits | 5998 | ||
11.5.2.3. Precious-Metal Deposits | 5999 | ||
11.5.2.4. Uranium Deposits | 5999 | ||
11.5.2.5. Diamond Deposits | 6000 | ||
11.5.2.6. Other Deposits | 6000 | ||
11.5.3. Sulfide Oxidation and the Generation of Oxidation Products | 6000 | ||
11.5.3.1. Sulfide-Mineral Oxidation | 6001 | ||
11.5.3.1.1. Pyrite oxidation | 6001 | ||
11.5.3.1.1.1. Chemical oxidation by Fe3+ and O2 | 6001 | ||
11.5.3.1.2. Pyrrhotite oxidation | 6002 | ||
11.5.3.1.2.1. Chemical oxidation by O2 and Fe3 | 6003 | ||
11.5.3.1.2.2. Nonoxidative mechanism | 6003 | ||
11.5.3.1.3. Oxidation of other metal sulfides | 6003 | ||
11.5.3.1.3.1. Sphalerite | 6003 | ||
11.5.3.1.3.2. Galena and chalcopyrite | 6003 | ||
11.5.3.1.3.3. Mercury sulfides | 6004 | ||
11.5.3.1.4. Oxidation of arsenic sulfides | 6004 | ||
11.5.3.2. Bacteria and Sulfide-Mineral Oxidation | 6005 | ||
11.5.3.2.1. Mechanisms of sulfide-mineral dissolution and the role of microorganisms | 6005 | ||
11.5.3.2.2. Biodiversity of iron- and sulfur-oxidizing acidophilic microorganisms | 6007 | ||
11.5.3.2.2.1. Acidophilic bacteria and archaea that oxidize sulfur | 6009 | ||
11.5.3.2.2.2. Acidophilic bacteria and archaea that oxidize both iron and sulfur | 6010 | ||
11.5.4. Acid-Neutralization Mechanisms at Mine Sites | 6010 | ||
11.5.4.1. Mechanisms of Acid Neutralization | 6010 | ||
11.5.4.1.1. Carbonate-mineral dissolution | 6011 | ||
11.5.4.1.2. Dissolution of hydroxide minerals | 6012 | ||
11.5.4.1.3. Dissolution of silicate minerals | 6012 | ||
11.5.4.1.4. Development of pH-buffering sequences | 6013 | ||
11.5.5. Geochemistry and Mineralogy of Secondary Minerals | 6013 | ||
11.5.5.1. Soluble Sulfates: Iron Minerals | 6013 | ||
11.5.5.2. Soluble Sulfates: Other Elements | 6013 | ||
11.5.5.3. Less Soluble Sulfate Minerals | 6014 | ||
11.5.5.4. Metal Oxides and Hydroxides | 6015 | ||
11.5.5.5. Carbonate Minerals | 6016 | ||
11.5.5.6. Arsenic Oxides | 6016 | ||
11.5.5.7. Phosphates | 6017 | ||
11.5.5.8. Secondary Sulfides | 6017 | ||
11.5.5.9. Role of Microorganisms in the Formation and Dissolution of Secondary Minerals | 6018 | ||
11.5.6. AMD in Mines and Mine Wastes | 6021 | ||
11.5.6.1. Underground Workings | 6021 | ||
11.5.6.2. Open-Pits | 6022 | ||
11.5.6.3. Waste-Rock Piles | 6024 | ||
11.5.6.4. Coal-Mine Spoils | 6026 | ||
11.5.6.5. Tailings Impoundments | 6027 | ||
11.5.7. Bioaccumulation and Toxicity of Oxidation Products | 6030 | ||
11.5.7.1. Uptake and Bioaccumulation | 6030 | ||
11.5.7.2. Toxicity of Oxidation Products | 6031 | ||
11.5.7.3. Assessment of Toxicity | 6031 | ||
11.5.7.3.1. Predictive models | 6031 | ||
11.5.7.3.2. Biologic sensors | 6032 | ||
11.5.7.3.3. Molecular tools | 6032 | ||
11.5.8. Methods of Prediction | 6032 | ||
11.5.8.1. Laboratory Static Procedures | 6032 | ||
11.5.8.2. Mineralogical Prediction | 6033 | ||
11.5.8.3. Laboratory Dynamic Procedures | 6034 | ||
11.5.8.4. Geochemical Models | 6034 | ||
11.5.8.4.1. Geochemical modeling approaches | 6034 | ||
11.5.8.4.2. Application of geochemical speciation mass-transfer models | 6035 | ||
11.5.8.5. Reactive-Transport Models | 6036 | ||
11.5.9. Approaches for Remediation and Prevention | 6036 | ||
11.5.9.1. Collection and Treatment | 6037 | ||
11.5.9.2. Controls on Sulfide Oxidation | 6037 | ||
11.5.9.2.1. Physical barriers | 6037 | ||
11.5.9.2.1.1. Subaqueous disposal | 6037 | ||
11.5.9.2.1.2. Dry covers | 6038 | ||
11.5.9.2.1.3. Synthetic covers | 6038 | ||
11.5.9.2.1.4. Oxygen-consuming materials | 6038 | ||
11.5.9.2.2. Chemical treatments | 6039 | ||
11.5.9.2.3. Bactericides | 6039 | ||
11.5.9.3. Passive Remediation Techniques | 6039 | ||
11.5.9.3.1. Types of passive systems | 6039 | ||
11.5.9.3.2. Anaerobic bioreactors | 6039 | ||
11.5.9.3.3. Constructed wetlands | 6040 | ||
11.5.9.3.4. Permeable reactive barriers | 6040 | ||
11.5.9.3.5. Other in situ techniques | 6041 | ||
11.5.10. Summary and Conclusions | 6042 | ||
References | 6042 | ||
Chapter 11.6: Radioactivity, Geochemistry, and Health | 6054 | ||
11.6.1. Introduction | 6055 | ||
11.6.1.1. Approach and Outline of Chapter | 6055 | ||
11.6.1.2. Previous Reviews and Scope of the Chapter | 6055 | ||
11.6.2. Radioactive Processes and Sources | 6056 | ||
11.6.2.1. Radioactive Processes | 6056 | ||
11.6.2.2. Overview of Radioactive Sources and Exposure | 6058 | ||
11.6.2.2.1. Natural sources of radioactivity | 6058 | ||
11.6.2.2.2. Nuclear waste | 6058 | ||
11.6.2.2.3. Sites of radioactive environmental contamination | 6059 | ||
11.6.2.2.4. Exposure to background and anthropogenic sources of radioactivity | 6059 | ||
11.6.3. Radionuclide Geochemistry: Principles and Methods | 6059 | ||
11.6.3.1. Aqueous Speciation and Solubility | 6060 | ||
11.6.3.1.1. Experimental studies | 6060 | ||
11.6.3.1.2. Aqueous speciation and solubility models | 6060 | ||
11.6.3.2. Sorption | 6060 | ||
11.6.3.2.1. Experimental studies | 6060 | ||
11.6.3.2.2. Sorption models | 6061 | ||
11.6.3.2.3. Reactive transport models | 6062 | ||
11.6.3.3. Colloids | 6063 | ||
11.6.3.3.1. Introduction | 6063 | ||
11.6.3.3.2. Microbial and humic colloids | 6063 | ||
11.6.3.3.3. Models for transport of radionuclides by colloids | 6064 | ||
11.6.4. Environmental Radioactivity and Health Effects Relevant to Drinking Water, the Nuclear Fuel Cycle, and Nuclear Weap | 6064 | ||
11.6.4.1. Radium in Groundwater | 6065 | ||
11.6.4.1.1. Geological occurrence | 6065 | ||
11.6.4.1.2. Geochemical controls on Ra concentrations | 6066 | ||
11.6.4.1.3. Isotopic ratios | 6066 | ||
11.6.4.1.3.1. 228Ra/226Ra ratios | 6066 | ||
11.6.4.1.3.2. 224Ra/228Ra ARs | 6067 | ||
11.6.4.1.4. Radium removal and generation of TENORM | 6067 | ||
11.6.4.1.5. Health effects owing to radium exposure | 6068 | ||
11.6.4.2. Uranium and Other Actinides [An(III), An(IV), An(V), An(VI)] | 6069 | ||
11.6.4.2.1. General trends in speciation, solubility, and sorption of the actinides | 6069 | ||
11.6.4.2.1.1. Oxidation state | 6069 | ||
11.6.4.2.1.2. Complexation and solubility | 6070 | ||
11.6.4.2.1.3. Actinide sorption | 6070 | ||
11.6.4.2.2. Behavior of uranium in the environment and impacts on human health | 6072 | ||
11.6.4.2.2.1. Radiochemical, geochemical, and biogeochemical properties of uranium | 6072 | ||
11.6.4.2.2.2. Environmental distribution of uranium | 6074 | ||
11.6.4.2.2.2.1. Overview: relationship between geochemical properties and distribution | 6074 | ||
11.6.4.2.2.2.2. Air | 6074 | ||
11.6.4.2.2.2.3. Rocks and soils | 6075 | ||
11.6.4.2.2.2.4. Water: background concentration levels | 6075 | ||
11.6.4.2.2.2.5. Water concentrations at contaminated sites | 6075 | ||
11.6.4.2.2.3. Uranium exposure and human health | 6076 | ||
11.6.4.2.2.3.1. Exposure pathways | 6076 | ||
11.6.4.2.2.3.2. Environmental toxicology and epidemiology of uranium | 6076 | ||
11.6.4.2.2.4. Application: uranium contamination from mining | 6077 | ||
11.6.4.2.2.4.1. Overview of mining, processing, and disposal | 6077 | ||
11.6.4.2.2.4.2. Uranium mill tailings: introduction | 6077 | ||
11.6.4.2.2.4.3. Geochemistry of mill tailings | 6078 | ||
11.6.4.2.2.4.3.1. Mineralogy of tailings | 6078 | ||
11.6.4.2.2.4.3.2. Geochemistry of aqueous contamination from mill tailings | 6078 | ||
11.6.4.2.2.4.3.3. Bacterial processes | 6079 | ||
11.6.4.2.2.4.3.4. Long-term behavior of mill tailings:&/I | 6079 | ||
11.6.4.2.2.4.4. ISR of Uranium | 6080 | ||
11.6.4.2.2.4.4.1. General description | 6080 | ||
11.6.4.2.2.4.4.2. Choice of leaching solution | 6080 | ||
11.6.4.2.2.4.4.3. Processing | 6080 | ||
11.6.4.2.2.4.4.4. Site restoration | 6081 | ||
11.6.4.2.2.4.4.5. Environmental issues | 6081 | ||
11.6.4.2.2.4.4.6. Case histories | 6082 | ||
11.6.4.2.2.4.4.7. Current controversies | 6083 | ||
11.6.4.2.2.4.4.8. NA for ISR sites | 6083 | ||
11.6.4.2.2.4.5. Remediation of uranium-contaminated sites | 6084 | ||
11.6.4.2.2.4.5.1. Permeable reactive barriers | 6084 | ||
11.6.4.2.2.4.5.2. Bioremediation | 6085 | ||
11.6.4.2.2.4.5.3. Monitored natural attenuation | 6085 | ||
11.6.4.2.3. Actinides and nuclear waste disposal | 6086 | ||
11.6.4.2.3.1. Speciation, solubility, and sorption of actinides in nuclear waste repository environments | 6087 | ||
11.6.4.2.3.1.1. Americium | 6087 | ||
11.6.4.2.3.1.2. Thorium | 6088 | ||
11.6.4.2.3.1.3. Neptunium | 6088 | ||
11.6.4.2.3.1.4. Plutonium | 6090 | ||
11.6.4.2.3.1.5. Uranium | 6091 | ||
11.6.4.2.3.1.6. Actinide sorption by uranium wastes | 6092 | ||
11.6.4.2.3.2. Geochemical models in risk assessment for | 6092 | ||
11.6.4.3. Fission Products | 6093 | ||
11.6.4.3.1. Introduction | 6093 | ||
11.6.4.3.2. Geochemistry of fission products | 6093 | ||
11.6.4.3.2.1. 90Sr | 6093 | ||
11.6.4.3.2.2. 137Cs | 6094 | ||
11.6.4.3.2.3. 99Tc | 6094 | ||
11.6.4.3.2.4. 129I | 6094 | ||
11.6.4.3.3. Application: the Chernobyl reactor accident | 6095 | ||
11.6.4.3.3.1. Introduction | 6095 | ||
11.6.4.3.3.2. Geochemical behavior | 6095 | ||
11.6.4.3.3.3. Environmental epidemiology | 6096 | ||
11.6.4.3.4. Application: contamination from weapons production at Chelyabins | 6096 | ||
11.6.4.3.4.1. Chelyabinsk complex | 6096 | ||
11.6.4.3.4.2. Environmental epidemiology | 6097 | ||
11.6.5. Summary | 6097 | ||
Appendix A. Radioactivity and Human Health | 6098 | ||
Basis for Health Effects from Radiation: Interactions between Io | 6099 | ||
Equivalent Dose and Biokinetics | 6099 | ||
Dosimetry and Environmental Exposure | 6100 | ||
Linear Nonthreshold Dose–Response Curve | 6100 | ||
Epidemiological Studies of the Effects of Radiation | 6101 | ||
Populations Living Near Nuclear Power Plants | 6101 | ||
Survivors of Atomic Bomb Blasts at Hiroshima and Nagasaki | 6102 | ||
Regulating Radionuclide Releases to the Environment and Exposures to the Public | 6102 | ||
US Regulations | 6103 | ||
European Regulations | 6103 | ||
Appendix B Health Effects of Uranium | 6103 | ||
Absorption | 6103 | ||
Distribution | 6104 | ||
Toxicity | 6104 | ||
Carcinogencity | 6104 | ||
Environmental Health Regulations | 6105 | ||
Acknowledgments | 6105 | ||
References | 6105 | ||
Chapter 11.7: The Environmental and Medical Geochemistry of Potentially Hazardous Materials Produced by Disasters | 6120 | ||
11.7.1. Introduction | 6121 | ||
11.7.2. Potentially Hazardous Materials Produced by Disasters | 6121 | ||
11.7.3. Medical Geochemistry – A Review and Update | 6123 | ||
11.7.3.1. Factors Influencing the Health Effects of Disaster Materials | 6128 | ||
11.7.3.2. Interdisciplinary Methods Used to Study the Health Effects of Disaster Materials | 6129 | ||
11.7.4. Sampling, Analytical, and Remote Sensing Methods Applied to Disaster Materials | 6130 | ||
11.7.4.1. Spatially Extensive Sampling in Rapid Response and over the Long Term | 6130 | ||
11.7.4.2. Safety during Sampling | 6130 | ||
11.7.4.3. Sampling Methods for Deposits of Solid Samples | 6130 | ||
11.7.4.4. Sampling Methods for Airborne Particulate Matter and other Aerosols | 6131 | ||
11.7.4.5. Field Analytical Methods for Solids | 6131 | ||
11.7.4.6. Sampling and Field Analysis Methods for Waters | 6131 | ||
11.7.4.7. Processing and Preparation of Solid DM for Lab Analysis | 6131 | ||
11.7.4.8. Laboratory Methods for the Analysis of Disaster Materials | 6132 | ||
11.7.4.9. Remote Sensing Methods for Identification and Mapping of Disaster Materials | 6133 | ||
11.7.5. Volcanic Eruptions and Volcanic Degassing | 6133 | ||
11.7.5.1. Volcanic Gases, Vog, and Laze | 6133 | ||
11.7.5.2. Crater Lake Gas Eruption Disasters – Lake Nyos as an Example | 6134 | ||
11.7.5.3. Volcanic Ash | 6134 | ||
11.7.6. Landslides, Debris Flows, and Lahars | 6136 | ||
11.7.6.1. Landslides and Debris Flows Sourced in Ultramafic Rocks | 6137 | ||
11.7.6.2. Landslides and Debris Flows Sourced in Sulfide-Rich Rocks | 6137 | ||
11.7.6.3. Volcanic Lahars | 6137 | ||
11.7.6.4. Pathogens in Dusts from Earthquake-Generated Landslides | 6138 | ||
11.7.7. Hurricanes, Extreme Storms, and Floods – Katrina as an Exam | 6138 | ||
11.7.7.1. An Overview of DM Produced by Hurricanes, Extreme Storms, and Floods | 6138 | ||
11.7.7.2. Hurricane Katrina Floodwaters, Flood Sediments, and Molds | 6138 | ||
11.7.8. Wildfires at the Wildland–Urban Interface | 6141 | ||
11.7.8.1. Types of Ash Produced by Wildfires | 6142 | ||
11.7.8.2. Ash, Debris, and Burned Soil Characteristics of Potential Environmental or Health Concern | 6142 | ||
11.7.8.3. Potential Environmental and Health Concerns of Wildfire Ash | 6144 | ||
11.7.9. Mud and Waters from the Lusi Mud Eruption, East Java, Indonesia | 6145 | ||
11.7.10. Failures of Mill Tailings or Mineral-Processing Waste Impoundments | 6146 | ||
11.7.10.1. Mine Waste and Mill Tailings Impoundment Failures, Marinduque Island, Philippines | 6146 | ||
11.7.10.2. Mill Tailings Impoundment Failure, Aznalcóllar Mine, Spain | 6148 | ||
11.7.10.3. Cyanide Processing Impoundment Failures – Baia Mare, Romania, as an Example | 6149 | ||
11.7.10.4. Red Mud Spill from Bauxite Processing, Hungary | 6150 | ||
11.7.10.5. General Insights Regarding Environmental and Health Impacts of Mineral-Processing Impound | 6151 | ||
11.7.11. Failures of Coal Slurry or Coal Fly Ash Impoundments | 6151 | ||
11.7.11.1. Coal Slurry Impoundment Failures | 6152 | ||
11.7.11.2. Coal Fly Ash Impoundment Failures | 6152 | ||
11.7.12. Building Collapse – The World Trade Center as an Example | 6154 | ||
11.7.13. Disaster Preparedness | 6159 | ||
11.7.14. Summary | 6161 | ||
Acknowledgments | 6161 | ||
References | 6161 | ||
Chapter 11.8: Eutrophication of Freshwater Systems | 6168 | ||
11.8.1. Introduction | 6169 | ||
11.8.1.1. Importance and Structure of Water | 6169 | ||
11.8.1.2. Movement over and through Land | 6170 | ||
11.8.1.3. Implications for Overloading the System | 6170 | ||
11.8.2. Nutrient Cycles in Aquatic Ecosys | 6170 | ||
11.8.2.1. Phosphorus | 6171 | ||
11.8.2.2. Nitrogen | 6172 | ||
11.8.3. Aquatic Ecosystem Structure | 6174 | ||
11.8.3.1. Nutrient Input Patterns | 6174 | ||
11.8.3.2. Lotic Systems | 6174 | ||
11.8.3.2.1. Determining order | 6175 | ||
11.8.3.2.2. Runoff, discharge, and loading rates | 6175 | ||
11.8.3.3. Wetlands | 6176 | ||
11.8.3.4. Lentic Systems | 6176 | ||
11.8.3.4.1. Temperature and mixing | 6177 | ||
11.8.3.4.2. Light, turbidity, and primary productivity | 6177 | ||
11.8.3.4.3. Nutrient ratios and phytoplankton dynamics | 6178 | ||
11.8.4. Eutrophication | 6180 | ||
11.8.4.1. Ecological Succession versus Eutr | 6180 | ||
11.8.4.2. Natural Eutrophication | 6180 | ||
11.8.4.3. Cultural Eutrophication | 6180 | ||
11.8.4.4. Conditions that Affect Eutrophication | 6180 | ||
11.8.4.4.1. Dissolved organic carbon | 6180 | ||
11.8.4.4.2. Salinity | 6181 | ||
11.8.4.4.3. Fire | 6181 | ||
11.8.4.4.4. Drought | 6181 | ||
11.8.5. Two Case Studies in Eutrophicatio | 6182 | ||
11.8.5.1. A Point-Source-Impacted Deep Water Lake | 6182 | ||
11.8.5.2. A Nonpoint-Source-Impacted Shallow Water Lake | 6183 | ||
11.8.6. Future Opportunities | 6184 | ||
11.8.6.1. Management | 6184 | ||
11.8.6.2. Monitoring | 6184 | ||
11.8.7. Conclusions | 6185 | ||
References | 6185 | ||
Chapter 11.9: Salinization and Saline Environments | 6188 | ||
11.9.1. Introduction | 6190 | ||
11.9.2. River Salinization | 6192 | ||
11.9.3. Lake Salinization | 6199 | ||
11.9.4. Groundwater Salinization | 6201 | ||
11.9.4.1. Seawater Intrusion and Saltwater Displacement in Coastal Aquifers | 6201 | ||
11.9.4.2. Mixing with External Saline Waters in Noncoastal Areas | 6205 | ||
11.9.4.3. Salinization of Shallow Groundwater in River Basins | 6207 | ||
11.9.4.4. Salinization of Nonrenewable Groundwater | 6208 | ||
11.9.5. Salinization of Dryland Environment | 6209 | ||
11.9.6. Anthropogenic Salinization | 6211 | ||
11.9.6.1. Urban Environment and Wastewater Salinization | 6211 | ||
11.9.6.2. Deicing and Salinization | 6213 | ||
11.9.6.3. Agricultural Drainage and the Unsaturated Zone | 6213 | ||
11.9.7. Salinity and the Occurrence of Health-Related Contaminants | 6215 | ||
11.9.7.1. Fluoride and Salinity | 6216 | ||
11.9.7.2. Oxyanions and Salinity | 6216 | ||
11.9.7.2.1. Arsenic | 6216 | ||
11.9.7.2.2. Selenium | 6217 | ||
11.9.7.2.3. Boron | 6217 | ||
11.9.7.3. Naturally Occurring Radionuclides and Salinity | 6219 | ||
11.9.7.4. Trihalomethanes and Salinity | 6219 | ||
11.9.7.5. Salinity and Toxic Algae Bloom | 6220 | ||
11.9.8. Elucidating the Sources of Salinity | 6221 | ||
11.9.9. Remediation and the Chemical Composition of Desalination | 6226 | ||
Acknowledgments | 6230 | ||
References | 6230 | ||
Chapter 11.10: Acid Rain – Acidification and Recovery | 6242 | ||
11.10.1. Introduction | 6243 | ||
11.10.2. What Is Acidification? | 6245 | ||
11.10.3. Long-Term Acidification | 6246 | ||
11.10.3.1. Has Long-Term Acidification Occurred? | 6246 | ||
11.10.3.2. What Controls Long-Term Acidification? | 6247 | ||
11.10.4. Short-Term and Episodic Acidification | 6249 | ||
11.10.5. Drivers of Short-Term and Episodic Acidification | 6250 | ||
11.10.5.1. High Discharge from Snowmelt and Rain | 6250 | ||
11.10.5.2. Pulsed Release of SO4 and NO3 from Soils | 6250 | ||
11.10.5.3. Marine Aerosols | 6250 | ||
11.10.5.4. Organic Acidity | 6251 | ||
11.10.5.5. Dilution | 6251 | ||
11.10.5.6. In-Lake Processes Affecting pH and ANC | 6251 | ||
11.10.6. Effects of Acidification | 6252 | ||
11.10.6.1. Release of Al and other Elements | 6252 | ||
11.10.6.2. Nutrient Availability | 6253 | ||
11.10.7. Effects of a Changing Physical Climate on Acidification | 6255 | ||
11.10.7.1. NO3 | 6256 | ||
11.10.7.2. SO4 | 6256 | ||
11.10.7.3. CO2 | 6256 | ||
11.10.7.4. Organic Acids | 6257 | ||
11.10.7.5. Evaporation/Hydrology | 6257 | ||
11.10.7.6. Marine Aerosols | 6257 | ||
11.10.7.7. Biological Feedbacks | 6258 | ||
11.10.8. Acidification Trajectories through Recent Time | 6258 | ||
11.10.9. Longitudinal Acidification | 6259 | ||
11.10.10. Some Areas with Recently or Potentially Acidified Soft Waters | 6260 | ||
11.10.10.1. Eastern Canada | 6260 | ||
11.10.10.2. Eastern United States | 6260 | ||
11.10.10.3. British Isles | 6260 | ||
11.10.10.4. Scandinavia | 6261 | ||
11.10.10.5. Continental Europe | 6261 | ||
11.10.10.6. South America | 6261 | ||
11.10.10.7. Eastern Asia | 6262 | ||
11.10.11. Experimental Acidification and Deacidification of LowANC Systems | 6262 | ||
11.10.11.1. Experimental Acidification of Lakes | 6262 | ||
11.10.11.2. Experimental Acidification of Wetlands | 6262 | ||
11.10.11.3. Experimental Acidification of Terrestrial Ecosystems | 6262 | ||
11.10.11.4. Experimental Acidification of Streams | 6263 | ||
11.10.12. Remediation of Acidity | 6264 | ||
11.10.12.1. Ca Additions | 6264 | ||
11.10.12.2. Nutrient Additions to Eliminate Excess NO3 | 6264 | ||
11.10.12.3. Land Use | 6264 | ||
11.10.12.3.1. Deforestation | 6264 | ||
11.10.12.3.2. Afforestation | 6265 | ||
11.10.13. Chemical Modeling of Acidification of Soft Water Systems | 6265 | ||
11.10.13.1. SteadyState Models | 6265 | ||
11.10.13.2. Dynamic Models | 6266 | ||
11.10.14. Chemical Recovery from Anthropogenic Acidification | 6266 | ||
Acknowledgments | 6269 | ||
References | 6270 | ||
Chapter 11.11: Tropospheric Ozone and Photochemical Smog | 6278 | ||
11.11.1. Introduction | 6279 | ||
11.11.2. General Description of Photochemical Smog | 6279 | ||
11.11.2.1. Primary and Secondary Pollutants | 6279 | ||
11.11.2.2. Ozone | 6280 | ||
11.11.2.2.1. Urban ozone | 6280 | ||
11.11.2.2.2. Regional pollution events and long-distance transport | 6280 | ||
11.11.2.2.3. Ozone and the global troposphere | 6281 | ||
11.11.2.2.4. Ozone precursors: NOx, CO, and volatile organics | 6282 | ||
11.11.2.2.5. Impact of biogenics | 6283 | ||
11.11.2.3. Particulates | 6283 | ||
11.11.2.4. Environmental and Health Impacts | 6285 | ||
11.11.2.5. Long-Term Trends in Ozone and Particulates | 6287 | ||
11.11.3. Photochemistry of Ozone and Particulates | 6287 | ||
11.11.3.1. Ozone | 6287 | ||
11.11.3.1.1. Ozone formation | 6287 | ||
11.11.3.1.2. Odd hydrogen radicals | 6288 | ||
11.11.3.1.3. O3, NO, and NO2 | 6288 | ||
11.11.3.1.4. O3– NOx–VOC sensitivity and OH | 6289 | ||
11.11.3.1.5. Ozone formation in the remote troposphere | 6290 | ||
11.11.3.1.6. Ozone production efficiency | 6290 | ||
11.11.3.2. Chemistry of Aerosols | 6291 | ||
11.11.3.3. Ozone–Aerosol Interactions | 6292 | ||
11.11.4. Meteorological Aspects of Photochemical Smog | 6292 | ||
11.11.4.1. Dynamics | 6292 | ||
11.11.4.2. Ozone and Temperature | 6294 | ||
11.11.5. New Directions: Evaluation Based on Ambient Measurements | 6294 | ||
Acknowledgments | 6297 | ||
References | 6297 | ||
Chapter 11.12: Volatile Hydrocarbons and Fuel Oxygenates | 6302 | ||
11.12.1. Introduction | 6302 | ||
11.12.1.1. Scope of the Problem | 6302 | ||
11.12.1.2. Petroleum Chemical Composition | 6305 | ||
11.12.1.2.1. Crude oil | 6305 | ||
11.12.1.2.2. Fuels | 6307 | ||
11.12.1.2.3. Fuel oxygenates | 6307 | ||
11.12.1.2.4. Solvents, lubricants, and petrochemical feedstocks | 6309 | ||
11.12.1.3. Ecological Concerns and Human Exposure Pathways | 6309 | ||
11.12.2. The Petroleum Industry | 6311 | ||
11.12.2.1. Petroleum Exploration, Production, and Processing | 6311 | ||
11.12.2.2. Petroleum Transportation and Storage | 6312 | ||
11.12.2.3. Petroleum Usage | 6313 | ||
11.12.2.4. Disposal of Petroleum Wastes | 6314 | ||
11.12.3. Environmental Transport Processes | 6314 | ||
11.12.3.1. Phase Partitioning | 6314 | ||
11.12.3.2. Physical Transport | 6320 | ||
11.12.4. Transformation Processes | 6320 | ||
11.12.4.1. Abiotic Transformation | 6321 | ||
11.12.4.2. Biotic Transformation | 6321 | ||
11.12.4.2.1. Aerobic processes | 6322 | ||
11.12.4.2.2. Anaerobic processes | 6324 | ||
11.12.4.2.3. Fuel hydrocarbon and oxygenate mixtures | 6328 | ||
11.12.5. Environmental Restoration | 6329 | ||
11.12.5.1. Natural Attenuation Processes | 6329 | ||
11.12.5.2. Engineered or Enhanced Remediation | 6332 | ||
11.12.5.3. Innovative Tools to Assess Remediation | 6332 | ||
11.12.6. Challenges | 6335 | ||
Acknowledgments | 6336 | ||
References | 6336 | ||
Chapter 11.13: High Molecular Weight Petrogenic and Pyrogenic Hydrocarbons in Aquatic Environments | 6344 | ||
11.13.1. Introduction | 6344 | ||
11.13.2. Scope of Review | 6345 | ||
11.13.3. Sources | 6346 | ||
11.13.3.1. Petrogenic Hydrocarbons | 6346 | ||
11.13.3.2. Pyrogenic Sources of HMW Hydrocarbons | 6349 | ||
11.13.4. Pathways | 6352 | ||
11.13.5. Fate | 6354 | ||
11.13.5.1. Sorption | 6355 | ||
11.13.5.2. Volatilization | 6356 | ||
11.13.5.3. Water Dissolution and Solubility | 6357 | ||
11.13.5.4. Photochemical Reactions | 6358 | ||
11.13.5.5. Biodegradation | 6358 | ||
11.13.6. Carbon Isotope Geochemistry | 6360 | ||
11.13.6.1. Carbon Isotope Variations in PAH Sources | 6361 | ||
11.13.6.1.1. Pyrogenesis | 6361 | ||
11.13.6.1.2. Pedogenesis | 6362 | ||
11.13.6.2. Weathering and Isotopic Composition | 6363 | ||
11.13.6.3. Isotopic Source Apportionment of PAHs in St. John's Harbor: An Example | 6364 | ||
11.13.7. Synthesis | 6367 | ||
Acknowledgments | 6368 | ||
References | 6368 | ||
Chapter 11.14: Biogeochemistry of Halogenated Hydrocarbons | 6374 | ||
11.14.1. Introduction | 6374 | ||
11.14.2. Global Transport and Distribution of Halogenated Organic Compounds | 6374 | ||
11.14.2.1. Persistent Organic Pollutants | 6375 | ||
11.14.2.1.1. Global distribution mechanisms | 6375 | ||
11.14.2.1.2. Contaminant classification | 6376 | ||
11.14.2.2. Biogenic Pollutants and Anthropogenic Non-POPs | 6376 | ||
11.14.3. Sources and Environmental Fluxes | 6377 | ||
11.14.3.1. Adsorbable Organic Halogens | 6377 | ||
11.14.3.2. Alkyl Halides | 6378 | ||
11.14.3.3. Aryl Halides | 6379 | ||
11.14.4. Chemical Controls on Reactivity | 6380 | ||
11.14.4.1. Phase Partitioning | 6380 | ||
11.14.4.2. Reaction Energetics | 6381 | ||
11.14.5. Microbial Biogeochemistry and Bioavailability | 6382 | ||
11.14.5.1. Ecological Considerations | 6382 | ||
11.14.5.2. Matrix Interactions | 6383 | ||
11.14.6. Environmental Reactivity | 6384 | ||
11.14.6.1. Microbial Reactivity | 6384 | ||
11.14.6.2. Surface-Mediated Reactivity | 6386 | ||
11.14.6.3. Organic-Matter-Mediated Reactivity | 6387 | ||
11.14.6.4. Predictive Models: Structure–Reactivity Relationships | 6388 | ||
11.14.7. Implications for Environmental Cycling of Halogenated Hydrocarbons | 6389 | ||
11.14.8. Knowledge Gaps and Fertile Areas for Future Research | 6392 | ||
Acknowledgments | 6393 | ||
References | 6393 | ||
Chapter 11.15: The Geochemistry of Pesticides | 6398 | ||
11.15.1. Introduction | 6399 | ||
11.15.1.1. Previous Reviews of Pesticide Geochemistry | 6399 | ||
11.15.1.2. Scope of This Review | 6399 | ||
11.15.1.3. Biological Effects of Pesticide Compounds | 6399 | ||
11.15.1.4. Variations in Pesticide Use over Time and Space | 6400 | ||
11.15.1.5. Environmental Distributions in Relation to Use | 6400 | ||
11.15.1.6. Overview of Persistence in the Hydrologic System | 6402 | ||
11.15.2. Partitioning among Environmental Matrices | 6403 | ||
11.15.2.1. Partitioning between Soils, Sediments, and Natural Waters | 6403 | ||
11.15.2.2. Partitioning between Aquatic Biota and Natural Waters | 6406 | ||
11.15.2.3. Partitioning between Earth's Surface and the Atmosphere | 6406 | ||
11.15.2.3.1. Movement between air and natural waters | 6406 | ||
11.15.2.3.2. Movement between air, soil, and plant surfaces | 6407 | ||
11.15.3. Transformations | 6408 | ||
11.15.3.1. Photochemical Transformations | 6409 | ||
11.15.3.2. Neutral Reactions | 6410 | ||
11.15.3.3. Electron-Transfer Reactions | 6411 | ||
11.15.3.4. Governing Factors | 6413 | ||
11.15.3.4.1. Reactant concentrations | 6413 | ||
11.15.3.4.2. Structure and properties of the pesticide substrate | 6414 | ||
11.15.3.4.3. Structure and properties of other reactants | 6416 | ||
11.15.3.4.4. Physical factors | 6418 | ||
11.15.3.4.5. Geochemical environment | 6420 | ||
11.15.3.5. Effects of Transformations on Environmental Transport and Fate | 6424 | ||
11.15.3.6. Occurrence of Pesticide Transformation Products in the Hydrologic System | 6425 | ||
11.15.4. The Future | 6425 | ||
Acknowledgments | 6427 | ||
References | 6428 | ||
Chapter 11.16: The Biogeochemistry of Contaminant Groundwater Plumes Arising from Waste Disposal Facilities | 6436 | ||
11.16.1. Introduction | 6436 | ||
11.16.2. Source and Leachate Composition | 6437 | ||
11.16.3. Spreading of Pollutants in Groundwater | 6438 | ||
11.16.4. Biogeochemistry of Landfill Leachate Plumes | 6439 | ||
11.16.4.1. Redox Environments and Redox Buffering | 6439 | ||
11.16.4.2. Microbial Activity and Redox Processes | 6442 | ||
11.16.5. Overview of Processes Controlling Fate of Landfill Leachate Compounds | 6443 | ||
11.16.5.1. Dissolved Organic Matter, Inorganic Macrocomponents, and Heavy Metals | 6445 | ||
11.16.5.1.1. Dissolved organic carbon | 6445 | ||
11.16.5.1.2. Inorganic macrocomponents | 6445 | ||
11.16.5.1.3. Heavy metals | 6445 | ||
11.16.5.2. Xenobiotic Organic Compounds | 6445 | ||
11.16.6. Norman Landfill (United States) | 6447 | ||
11.16.6.1. Source, Geology, and Hydrogeology | 6447 | ||
11.16.6.2. Landfill Leachate Plume | 6447 | ||
11.16.6.2.1. Biogeochemistry of the plume | 6447 | ||
11.16.6.2.2. Availability of electron acceptors | 6452 | ||
11.16.6.2.3. Fate of XOCs | 6453 | ||
11.16.7. Grindsted Landfill Site (DK) | 6454 | ||
11.16.7.1. Source, Geology, and Hydrogeology | 6454 | ||
11.16.7.2. Landfill Leachate Plume | 6456 | ||
11.16.8. Monitored Natural Attenuation | 6462 | ||
11.16.9. Future Challenges | 6463 | ||
References | 6464 | ||
e9780080983004v12 | 6469 | ||
Front Cover | 6469 | ||
Organic Geochemistry | 6472 | ||
Copyright | 6473 | ||
In Memoriam | 6474 | ||
Heinrich Dieter Holland (1927–2012) | 6476 | ||
Karl Karekin Turekian (1927–2013) | 6478 | ||
Dedication | 6482 | ||
Contents | 6484 | ||
Executive Editors’ Foreword to the Second Edition | 6486 | ||
Contributors | 6490 | ||
Volume Editors’ Introduction | 6492 | ||
Introduction | 6492 | ||
Chapter 12.1: Organic Geochemistry of Meteorites | 6494 | ||
12.1.1. Meteorites and Their Carbon | 6494 | ||
12.1.2. Classification of Carbonaceous Chondrites | 6495 | ||
12.1.3. Stable Isotopes and Carbonaceous Chondrites | 6495 | ||
12.1.4. The Organic Compounds in Carbonaceous Chondrites | 6497 | ||
12.1.5. Carboxylic Acids | 6497 | ||
12.1.5.1. Short-Chain Monocarboxylic Acids | 6497 | ||
12.1.5.2. Long-Chain Monocarboxylic Acids | 6499 | ||
12.1.5.3. Hydroxycarboxylic Acids | 6499 | ||
12.1.5.4. Dicarboxylic Acids | 6499 | ||
12.1.5.5. Aromatic Carboxylic Acids | 6500 | ||
12.1.5.6. Stable Isotopes and Carboxylic Acids | 6500 | ||
12.1.6. Amino Acids | 6501 | ||
12.1.6.1. Free Amino Acids | 6501 | ||
12.1.6.2. Enantiomeric Excesses | 6503 | ||
12.1.6.3. Origin of Enantiomeric Excess | 6504 | ||
12.1.6.4. Amino Acid Precursors | 6504 | ||
12.1.6.5. Stable Isotopes and Amino Acids | 6505 | ||
12.1.7. Amines and Amides | 6506 | ||
12.1.8. Aliphatic Hydrocarbons | 6506 | ||
12.1.8.1. Short-Chain Alkanes | 6506 | ||
12.1.8.2. Normal Alkanes | 6506 | ||
12.1.8.3. Stable Isotopes and Short-Chain Alkanes | 6507 | ||
12.1.8.4. Stable Isotopes and Normal Alkanes | 6508 | ||
12.1.9. Aromatic Hydrocarbons | 6508 | ||
12.1.9.1. Solvent and Thermal Extracts | 6508 | ||
12.1.9.2. Laser Mass Spectrometry | 6509 | ||
12.1.9.3. Stable Isotopes and Aromatic Hydrocarbons | 6509 | ||
12.1.10. Nucleic Acid Bases and Other Nitrogen Heterocycles | 6510 | ||
12.1.11. Alcohols, Polyhydroxylated Compounds, and Carbonyls | 6511 | ||
12.1.12. Sulfonic and Phosphonic Acids | 6512 | ||
12.1.12.1. Chemical Structures | 6512 | ||
12.1.12.2. Stable Isotopes and Sulfonic and Phosphonic Acids | 6512 | ||
12.1.13. Organohalogens | 6512 | ||
12.1.14. Macromolecular Material | 6512 | ||
12.1.14.1. Aliphatic and Aromatic Units | 6512 | ||
12.1.14.2. Oxygen-Containing Units | 6513 | ||
12.1.14.3. Sulfur-Containing Units | 6514 | ||
12.1.14.4. Nitrogen-Containing Units | 6514 | ||
12.1.14.5. Radicals | 6514 | ||
12.1.14.6. Stable Isotopes and the Macromolecular Material | 6514 | ||
12.1.14.7. Variations Within and Between Macromolecular Materials | 6515 | ||
12.1.15. Microvesicles and Nanoglobules | 6516 | ||
12.1.16. Organic-Inorganic Relationships | 6518 | ||
12.1.17. Source Environments | 6518 | ||
References | 6519 | ||
Chapter 12.2: Organic Geochemical Signatures of Early Life on Earth | 6526 | ||
12.2.1. Introduction | 6526 | ||
12.2.2. Eoarchean (4.0-3.6Ga) Biological Remnants? | 6526 | ||
12.2.3. The Post-3.5Ga Sedimentary Record of Stable Carbon Isotopes | 6527 | ||
12.2.4. The Record of Organic Carbon Burial | 6528 | ||
12.2.5. The Composition of Buried Organic Matter | 6530 | ||
12.2.6. Visible Structures with Organic Affinities | 6533 | ||
12.2.6.1. Organic-Walled Microfossils | 6533 | ||
12.2.6.2. Fossil Microbial Mats, Textures, and Trace Fossils | 6534 | ||
12.2.6.3. Stromatolites | 6534 | ||
12.2.7. Summary and Prospects | 6535 | ||
Acknowledgments | 6536 | ||
References | 6536 | ||
Chapter 12.3: The Analysis and Application of Biomarkers | 6540 | ||
12.3.1. Introduction | 6540 | ||
12.3.2. Biomarkers and Environments | 6542 | ||
12.3.2.1. Introduction | 6542 | ||
12.3.2.2. Hopanoids | 6546 | ||
12.3.2.3. Steranes | 6547 | ||
12.3.2.4. Marine/Lacustrine Environments | 6548 | ||
12.3.2.5. Marine Environments | 6548 | ||
12.3.2.6. Lacustrine Environments | 6549 | ||
12.3.2.7. Terrigenous Environments | 6549 | ||
12.3.2.7.1. Steranes | 6549 | ||
12.3.2.7.2. n-Alkanes | 6549 | ||
12.3.2.7.3. Diterpenoids | 6550 | ||
12.3.2.7.4. Triterpenoids | 6550 | ||
12.3.2.8. Biomarkers for Specific Environmental Conditions | 6551 | ||
12.3.2.8.1. Photic zone euxinia | 6551 | ||
12.3.2.8.2. Purple sulfur bacteria | 6551 | ||
12.3.2.8.3. Green sulfur bacteria | 6552 | ||
12.3.2.8.4. Archaea | 6554 | ||
12.3.2.8.5. Glycerol dialkyl glycerol tetraethers | 6554 | ||
12.3.2.8.6. TEX86 and other GDGT-based palaeotemperature proxies | 6556 | ||
12.3.2.8.7. Halophilic archaea | 6556 | ||
12.3.3. Age-Diagnostic Biomarkers | 6557 | ||
12.3.3.1. n-Alkanes | 6557 | ||
12.3.3.2. Evolution of the Land Plants in the Late Devonian | 6557 | ||
12.3.3.3. Evolution of Flowering Plants in the Cretaceous | 6557 | ||
12.3.3.4. Botryococcanes in Tertiary Lakes | 6558 | ||
12.3.3.5. Evolution of Demospongiae in the Latest Neoproterozoic | 6558 | ||
12.3.3.6. Evolution of Dinoflagellates in the Late Mesozoic | 6558 | ||
12.3.3.7. Evolution of Diatoms in the Jurassic | 6558 | ||
12.3.3.8. Age-Diagnostic Steranes | 6559 | ||
12.3.4. Biomarkers of Fungi | 6559 | ||
12.3.5. Biomarkers and Extinction Events | 6559 | ||
12.3.5.1. Permian/Triassic | 6559 | ||
12.3.5.2. Triassic/Jurassic | 6560 | ||
12.3.5.3. The Palaeocene-Eocene Thermal Maximum | 6561 | ||
12.3.6. Analytical Approaches | 6561 | ||
12.3.6.1. Analytical Pyrolysis | 6561 | ||
12.3.6.2. Laser Micropyrolysis GC-MS | 6562 | ||
12.3.6.3. Catalysed HyPy | 6562 | ||
12.3.6.4. Stable Isotope Analysis | 6562 | ||
12.3.6.5. Comprehensive Two-Dimensional Gas Chromatography (GCxGC) | 6563 | ||
12.3.6.6. Time-of-Flight Secondary Ion Mass Spectrometry | 6563 | ||
12.3.7. Summary | 6563 | ||
Acknowledgments | 6563 | ||
References | 6563 | ||
Chapter 12.4: Hydrogen Isotope Signatures in the Lipids of Phytoplankton | 6572 | ||
12.4.1. Introduction | 6572 | ||
12.4.2. The Effect of δDwater on δDlipid | 6573 | ||
12.4.3. The Effect of Biosynthesis on δDlipid | 6575 | ||
12.4.4. The Effect of Species on δDlipid | 6576 | ||
12.4.5. The Effect of Salinity on δDlipid | 6576 | ||
12.4.6. The Effect of Temperature on δDlipid | 6580 | ||
12.4.7. The Effect of Growth Rate on δDlipid | 6582 | ||
12.4.7.1. Substrate-Limited Growth Rate Effects | 6582 | ||
12.4.7.2. Light-Limited Growth Rate Effects | 6585 | ||
12.4.8. Summary and Conclusions | 6585 | ||
Acknowledgments | 6585 | ||
References | 6585 | ||
Chapter 12.4: 13C/12C Signatures in Plants and Algae | 6588 | ||
12.5.1. Introduction | 6588 | ||
12.5.2. The Term ‘Isotopic Fractionation’ | 6589 | ||
12.5.3. Isotopic Fractionation in Plants and Algae | 6592 | ||
12.5.3.1. Outline | 6592 | ||
12.5.3.2. δ13C of Source Carbon | 6592 | ||
12.5.3.3. Isotopic Fractionation During Photosynthesis | 6594 | ||
12.5.3.3.1. Outline | 6594 | ||
12.5.3.3.2. Terrestrial C3 plants | 6594 | ||
12.5.3.3.3. Aquatic plants and algae | 6596 | ||
12.5.3.3.4. Terrestrial C4 plants | 6596 | ||
12.5.3.3.5. Terrestrial CAM plants | 6596 | ||
12.5.3.3.6. Experimental values for | 6597 | ||
12.5.3.3.7. Other factors | 6597 | ||
12.5.3.4. Model for Isotopic Fractionation in Biosynthetic Products | 6598 | ||
12.5.3.5. Sources of Carbon Atoms for Biosynthetic Products | 6602 | ||
12.5.3.5.1. Outline | 6602 | ||
12.5.3.5.2. Lipids | 6602 | ||
12.5.3.5.3. Amino acids | 6603 | ||
12.5.3.5.4. Chlorophylls | 6607 | ||
12.5.3.5.5. Nucleic acid bases | 6607 | ||
12.5.3.5.6. Lignin | 6609 | ||
12.5.3.6. A Deeper Understanding of Isotopic Fractionation in Lipid Biosynthesis | 6609 | ||
12.5.3.7. Topics for Further Study | 6611 | ||
References | 6612 | ||
Chapter 12.6: Dissolved Organic Matter in Aquatic Systems | 6618 | ||
12.6.1. Introduction | 6620 | ||
12.6.2. Inventory and Fluxes | 6621 | ||
12.6.2.1. The Global Inventory | 6621 | ||
12.6.2.2. Sources to the Ocean | 6621 | ||
12.6.2.3. Turnover and Flux of Marine DOM | 6622 | ||
12.6.2.4. What Happens to Terrigenous DOM? | 6624 | ||
12.6.2.5. Global Distribution | 6624 | ||
12.6.3. Bulk Chemical Properties | 6626 | ||
12.6.3.1. Carbon Isotopes | 6626 | ||
12.6.3.1.1. Stable carbon isotopes | 6626 | ||
12.6.3.1.2. Radiocarbon | 6626 | ||
12.6.3.2. Optical Properties | 6627 | ||
12.6.3.2.1. Colored DOM | 6627 | ||
12.6.3.2.2. Fluorescent DOM | 6630 | ||
12.6.3.3. Nuclear Magnetic Resonance | 6631 | ||
12.6.4. The Composition of DOM on an Individual Molecular Level | 6633 | ||
12.6.4.1. Introduction | 6633 | ||
12.6.4.2. Ultrahigh-Resolution Mass Spectrometry | 6634 | ||
12.6.4.3. Interaction Among Individual Molecules | 6638 | ||
12.6.4.4. Molecular Clues on the Source and Turnover of Marine DOM | 6639 | ||
12.6.4.4.1. Introduction | 6639 | ||
12.6.4.4.2. The fate of terrigenous DOM in the ocean | 6639 | ||
12.6.4.4.3. A thermogenic component | 6640 | ||
12.6.4.4.4. The microbial imprint | 6640 | ||
12.6.5. Reasons Behind the Stability of DOM in the Deep Ocean | 6641 | ||
12.6.6. Perspectives | 6643 | ||
12.6.6.1. What Is the Substrate on Which Marine Microorganisms Grow? | 6643 | ||
12.6.6.2. Why Do DOM Molecules Lose Their Physiological Function Over Time? | 6643 | ||
12.6.6.3. Reading the Molecular Archive for Historic Reconstruction | 6643 | ||
12.6.6.4. What Are the Organic Ligands of Essential Trace Metals? | 6644 | ||
Acknowledgments | 6644 | ||
References | 6644 | ||
Chapter 12.7: Dynamics, Chemistry, and Preservation of Organic Matter in Soils | 6650 | ||
12.7.1. Soil Organic Matter and Soil Functions | 6651 | ||
12.7.2. Input and Quantity of SOM | 6653 | ||
12.7.2.1. Amount of OM in Soils | 6653 | ||
12.7.2.2. Plant and Microbial Input to SOM | 6654 | ||
12.7.2.2.1. Aboveground input | 6654 | ||
12.7.2.2.2. Belowground input | 6655 | ||
12.7.2.3. Plant Compound Classes | 6655 | ||
12.7.2.3.1. Cellulose | 6656 | ||
12.7.2.3.2. Noncellulosic polysaccharides | 6656 | ||
12.7.2.3.3. Lignin | 6656 | ||
12.7.2.3.4. Tannins and other polyphenols | 6656 | ||
12.7.2.3.5. Lipids | 6656 | ||
12.7.2.3.6. Cutin and suberin | 6657 | ||
12.7.2.3.7. N-, S-, and P-containing compounds | 6657 | ||
12.7.2.3.8. Specific components of fungi and bacteria | 6658 | ||
12.7.2.4. Charcoal | 6660 | ||
12.7.3. Composition and Transformation of Organic Matter in Soils | 6661 | ||
12.7.3.1. Bulk SOM Composition | 6661 | ||
12.7.3.2. Organic Matter in Subsoils | 6665 | ||
12.7.4. Turnover of SOM | 6666 | ||
12.7.4.1. Pools and Models | 6666 | ||
12.7.4.2. Assessing Mean Residence Times on the Bases of C Isotopic Composition | 6667 | ||
12.7.4.2.1. δ13C abundance measurements as a tool for turnover assessment | 6667 | ||
12.7.4.2.2. Δ14C abundance measurements as a tool for turnover assessment | 6669 | ||
12.7.4.2.3. Turnover of OC in topsoils and subsoils | 6669 | ||
12.7.5. Origin and Turnover of Specific Components in Soils | 6670 | ||
12.7.5.1. Biomarkers for Plant-Derived C | 6670 | ||
12.7.5.1.1. Lignins | 6670 | ||
12.7.5.1.2. Tannins | 6673 | ||
12.7.5.1.3. Aliphatic compounds | 6673 | ||
12.7.5.1.4. Carbohydrates | 6674 | ||
12.7.5.2. Biomarkers for Living Microbial Biomass | 6674 | ||
12.7.5.2.1. Phospholipid fatty acids | 6674 | ||
12.7.5.2.2. Ergosterol and others | 6675 | ||
12.7.5.2.3. Glycerol dialkyl glycerol tetraethers | 6675 | ||
12.7.5.3. Biomarkers for Dead Microbial Biomass | 6675 | ||
12.7.5.3.1. Terpenoids | 6675 | ||
12.7.5.3.2. Nitrogen-containing biomarkers | 6675 | ||
12.7.5.4. Biomarkers for Off-Site Contributions to SOM | 6676 | ||
12.7.5.4.1. Steroids and bile acids | 6676 | ||
12.7.5.4.2. Benzene polycarboxylic acids | 6676 | ||
12.7.5.5. Examples for Applications in Soil Science | 6677 | ||
12.7.5.5.1. Changes in biomarker signature with prolonged arable cropping | 6677 | ||
12.7.5.5.2. Biomarkers in particle-size fractions | 6678 | ||
12.7.5.6. Turnover Rates of Different Biomarkers | 6679 | ||
12.7.5.6.1. Biomarker-specific stable isotope analyses in artificial labeling experiments | 6679 | ||
12.7.5.6.2. Biomarker-specific stable isotope analyses after C3/C4 vegetation change | 6680 | ||
12.7.6. Soil-Specific Interactions of OM with the Mineral Phase | 6682 | ||
12.7.6.1. Soil Architecture and Its Effects on C Turnover and Stabilization | 6682 | ||
12.7.6.1.1. Accessibility/aggregation | 6682 | ||
12.7.6.1.2. Organomineral interactions | 6684 | ||
12.7.6.1.3. Types of C and N in organomineral associations | 6684 | ||
12.7.6.1.4. Phyllosilicate clay minerals | 6684 | ||
12.7.6.1.5. Pedogenic oxides | 6685 | ||
12.7.6.1.6. Interactions with metal ions | 6685 | ||
12.7.6.2. SOM Formation in Major Soil Types | 6685 | ||
12.7.6.2.1. Chernozems | 6686 | ||
12.7.6.2.2. Podzols | 6687 | ||
12.7.6.2.3. Ferralsols | 6689 | ||
12.7.6.2.4. Cryosols | 6690 | ||
12.7.6.2.5. Andosols | 6691 | ||
12.7.6.2.6. Man-made soils (Anthrosols) | 6692 | ||
12.7.6.2.6.1. Paddy soils | 6692 | ||
12.7.6.2.6.2. Terra preta | 6694 | ||
12.7.7. Peculiarities | 6695 | ||
References | 6698 | ||
Chapter 12.8: Weathering of Organic Carbon | 6710 | ||
12.8.1. Introduction | 6710 | ||
12.8.2. Reservoirs and Fluxes in the Geochemical Carbon Cycle | 6710 | ||
12.8.2.1. Rock Reservoirs of Carbon | 6710 | ||
12.8.2.2. Addition of OM to the Rock Reservoir: OM Burial | 6711 | ||
12.8.2.3. Removal of OM from the Rock Reservoir: Weathering, Erosion, and Oxidation | 6712 | ||
12.8.3. Weathering of Kerogen | 6713 | ||
12.8.3.1. Weathering of Kerogen in Black Shales | 6713 | ||
12.8.3.1.1. Decreases in organic carbon content | 6713 | ||
12.8.3.1.2. Changes in kerogen composition accompanying weathering | 6714 | ||
12.8.3.1.3. Changes in bitumen composition during weathering | 6717 | ||
12.8.3.2. Metals and Minerals During Shale Weathering | 6717 | ||
12.8.3.3. Oxidative Weathering of OM-Rich Sediments | 6718 | ||
12.8.3.4. Models of Shale Weathering | 6719 | ||
12.8.4. Biodegradation of Sedimentary OM | 6719 | ||
12.8.4.1. Direct Biodegradation of Kerogen and Coal | 6719 | ||
12.8.4.2. Hydrocarbon Biodegradation During Weathering | 6720 | ||
12.8.4.3. Biodegradation of Rock-Derived Hydrocarbons | 6721 | ||
12.8.4.4. Fossil OM Biodegradation in the Subsurface | 6721 | ||
12.8.5. Surficial Transport and Transformations of Fossil OM | 6722 | ||
12.8.5.1. Kerogen Recycling Along Active Continental Margins | 6722 | ||
12.8.5.2. River Transport and Transformations of Rock-Derived OM | 6722 | ||
12.8.6. Model Estimates of Global Organic Carbon Weathering | 6723 | ||
12.8.6.1. Models of the Geochemical Cycles of Carbon and Oxygen | 6723 | ||
12.8.6.2. OC Loss Through Chemical Weathering of Sedimentary Rocks | 6725 | ||
12.8.6.3. Global Re Fluxes: Constraints on OM Weathering | 6727 | ||
12.8.7. Synthesis and Conclusions: Carbon Weathering in the Global Carbon Cycle | 6727 | ||
References | 6728 | ||
Chapter 12.9: Organic Carbon Cycling and the Lithosphere | 6732 | ||
12.9.1. Introduction | 6732 | ||
12.9.2. Carbon Content of the Continental Crust | 6732 | ||
12.9.3. Isotopic Constraints on Crustal Carbon | 6734 | ||
12.9.4. Cycling of Crustal Carbon | 6735 | ||
12.9.5. Inconsistencies in Crustal-Sedimentary Carbon Budgets | 6736 | ||
12.9.6. Carbon Cycling Under Reduced Atmospheric Oxygen Levels | 6738 | ||
12.9.7. Conclusions | 6740 | ||
References | 6741 | ||
Chapter 12.10: Organic Nitrogen: Sources, Fates, and Chemistry | 6744 | ||
12.10.1. Introduction | 6744 | ||
12.10.2. Nitrogen Assimilation and Isotopic Effects | 6745 | ||
12.10.2.1. Background | 6745 | ||
12.10.2.2. Nitrogen Assimilation Processes by Autotrophs | 6746 | ||
12.10.2.3. Nitrogen Isotopic Fractionation Associated with Autotrophic Nitrogen Assimilation | 6747 | ||
12.10.2.4. Nitrification and Denitrification | 6749 | ||
12.10.2.5. Applications to Oceanic Systems | 6750 | ||
12.10.3. Cellular Nitrogenous Compounds and Isotope Effects | 6751 | ||
12.10.3.1. Background | 6751 | ||
12.10.3.2. Amino Acids and Proteins | 6752 | ||
12.10.3.3. Nucleobases and Nucleic Acids | 6755 | ||
12.10.3.4. Chlorophylls | 6756 | ||
12.10.3.5. Other Tetrapyrroles | 6758 | ||
12.10.3.6. Other Nitrogenous Compounds | 6759 | ||
12.10.4. Organic Nitrogen in Sediments and Its Application to Paleoenvironmental Reconstructions | 6760 | ||
12.10.4.1. Background | 6760 | ||
12.10.4.2. Degradations of Proteins and Amino Acids and Isotopic Effects on Bulk Organic Nitrogen | 6761 | ||
12.10.4.3. Chlorins | 6763 | ||
12.10.4.4. Alkyl Porphyrins | 6765 | ||
12.10.4.5. Maleimides | 6768 | ||
12.10.4.6. Other Nitrogenous Compounds | 6769 | ||
12.10.5. Related Topics | 6770 | ||
12.10.5.1. Ecological and Archaeological Applications | 6770 | ||
12.10.5.2. Recent Advances in Enantiomer-Specific Nitrogen Isotope Analysis of Amino Acids | 6771 | ||
12.10.5.3. Extraterrestrial Nitrogenous Compounds | 6772 | ||
12.10.6. Conclusions | 6775 | ||
Acknowledgments | 6775 | ||
References | 6775 | ||
Chapter 12.11: Lipidomics for Geochemistry | 6784 | ||
12.11.1. Introduction | 6784 | ||
12.11.2. Lipid Biosynthetic Pathways | 6785 | ||
12.11.2.1. Acetogenic Lipids | 6785 | ||
12.11.2.2. Isoprenoids - MVA Pathway | 6788 | ||
12.11.2.3. Isoprenoids - MEP Pathway | 6789 | ||
12.11.2.4. Ether and Ester Linkages to Glycerol - Bacteria and Eukaryotes | 6790 | ||
12.11.2.5. Diethers and Tetraethers of Archaea | 6793 | ||
12.11.2.6. Glycerol Membrane Lipids: Final Comments | 6795 | ||
12.11.2.7. Polar Head Groups | 6795 | ||
12.11.2.8. Linear Polyprenes | 6801 | ||
12.11.2.9. Hopanoids | 6801 | ||
12.11.2.10. Steroids | 6802 | ||
12.11.2.11. Ladderanes | 6803 | ||
12.11.2.12. Long-Chain Alkenones | 6805 | ||
12.11.2.13. Highly Branched Isoprenoids of Diatoms | 6805 | ||
12.11.3. Case Studies and Approaches to Lipidomics | 6806 | ||
12.11.3.1. Examples Using Bacterial Genetics | 6807 | ||
12.11.3.1.1. Role of squalene-hopene cyclase in the synthesis of hopanoid lipids | 6808 | ||
12.11.3.1.2. Synthesis of A-ring methylated bacteriohopanepolyols | 6809 | ||
12.11.3.1.3. Synthesis of hopanoid C5 side chains | 6810 | ||
12.11.3.2. Examples Using Genomic Data from Characterized Species | 6810 | ||
12.11.3.2.1. Bacteria capable of sterol biosynthesis | 6811 | ||
12.11.3.2.2. Anaerobes capable of synthesizing hopanoids | 6811 | ||
12.11.3.2.3. The distribution of phosphatidylcholine in bacteria | 6812 | ||
12.11.3.2.4. Hypotheses about synthesis of ladderane lipids | 6812 | ||
12.11.3.3. Examples Using Environmental Metagenomics and Functional Genomics | 6813 | ||
12.11.3.3.1. Functional gene surveys - environmental shc genes | 6813 | ||
12.11.3.3.2. General metagenomic surveys - hopanoid synthesis genes | 6814 | ||
12.11.3.3.3. General metagenomic surveys - other lipid biosynthetic pathways | 6815 | ||
12.11.3.4. Examples Using Experimental Biochemical Approaches | 6817 | ||
12.11.3.4.1. Synthesis of botryococcene | 6817 | ||
12.11.3.4.2. Multifunctional 2,3-oxidosqualene cyclases in plants | 6817 | ||
12.11.3.5. Examples Using SSU rRNA Combined with Taxonomic Specificity of Biomarkers | 6818 | ||
12.11.3.5.1. Paleorecord of alkenone-producing haptophyte algae | 6819 | ||
12.11.3.5.2. Highly branched isoprenoids of diatoms | 6819 | ||
12.11.4. Conclusions | 6820 | ||
Acknowledgments | 6820 | ||
References | 6820 | ||
Chapter 12.12: Mineral Matrices and Organic Matter | 6830 | ||
12.12.1. Introduction | 6831 | ||
12.12.2. Evidence for Organic Matter Association with Minerals | 6833 | ||
12.12.2.1. Correlations with Grain Size and Mineral-Specific Surface Area | 6833 | ||
12.12.2.2. Why Do Organic Matter and Minerals Stick Together? | 6833 | ||
12.12.2.2.1. Sorption | 6834 | ||
12.12.2.2.1.1. Ligand exchange | 6834 | ||
12.12.2.2.1.2. Ion exchange | 6834 | ||
12.12.2.2.1.3. Cation bridging | 6834 | ||
12.12.2.2.1.4. Van der Waals forces | 6834 | ||
12.12.2.2.1.5. Hydrogen bonding | 6834 | ||
12.12.2.2.1.6. Hydrophobic interactions | 6834 | ||
12.12.2.2.1.7. Multimode sorption | 6835 | ||
12.12.2.2.1.8. Intercalation | 6836 | ||
12.12.2.2.2. Occlusion by biomineralization | 6836 | ||
12.12.2.2.3. Secondary architectures: aggregation | 6836 | ||
12.12.2.2.4. The role of mineral surface area and geometry | 6839 | ||
12.12.3. Impact on Organic Matter | 6839 | ||
12.12.3.1. Compositional Variations Among Different Mineral Associations | 6839 | ||
12.12.3.2. Organic Matter Stabilization and Destabilization | 6840 | ||
12.12.3.2.1. Abiotic and biotic roles of minerals in organic matter decay | 6840 | ||
12.12.3.2.2. Minerals as organic matter stabilizers | 6840 | ||
12.12.3.2.3. Does mineralogy influence protection? | 6842 | ||
12.12.3.3. Crosscutting Themes | 6843 | ||
12.12.3.3.1. Microbial roles | 6843 | ||
12.12.3.3.2. Oxygen effects on bulk organic matter persistence | 6844 | ||
12.12.3.3.3. Physical disturbance | 6845 | ||
12.12.4. Future Directions | 6846 | ||
12.12.5. Conclusion | 6847 | ||
Acknowledgments | 6847 | ||
References | 6847 | ||
Chapter 12.13: Biomarker-Based Inferences of Past Climate: The Alkenone pCO2 Proxy | 6854 | ||
12.13.1. Introduction | 6854 | ||
12.13.2. The Alkenone CO2 Proxy | 6854 | ||
12.13.2.1. Roots of the Methodology | 6854 | ||
12.13.2.2. Models of Algal Carbon Isotope Fractionation Assuming Diffusive Carbon Transport | 6855 | ||
12.13.2.3. Development of the Alkenone-CO2 Proxy | 6857 | ||
12.13.2.4. The Calibration of `b´ and Estimates of εf | 6858 | ||
12.13.3. CO2 Reconstructions, Uncertainties, and Complications | 6859 | ||
12.13.3.1. The Influence of Growth Rate and Irradiance | 6861 | ||
12.13.3.1.1. Dilute cultures and mesocosm experiments | 6861 | ||
12.13.3.1.2. Ocean and surface sediment data | 6862 | ||
12.13.3.1.3. The case against growth rate as the dominant influence on εp37:2 | 6862 | ||
12.13.3.2. Consideration of Cell Geometry Changes on Long-Term εp37:2 Records | 6863 | ||
12.13.4. Active Transport and the Case Against the Diffusive Model of Carbon Uptake | 6865 | ||
12.13.4.1. Models for Active Carbon Uptake | 6866 | ||
12.13.5. Summary | 6867 | ||
References | 6868 | ||
Chapter 12.14: Biomarker-Based Inferences of Past Climate: The TEX86 Paleotemperature Proxy | 6872 | ||
12.14.1. Introduction | 6872 | ||
12.14.2. History and Systematics | 6872 | ||
12.14.3. Detection and Analysis of GDGTs | 6874 | ||
12.14.4. Ecology of the Thaumarchaeota and Implications for TEX86 | 6874 | ||
12.14.5. Preservation of GDGT Lipids in Sediments | 6877 | ||
12.14.6. Calibration of TEX86 to Temperature | 6877 | ||
12.14.6.1. Mesocosm Calibrations | 6877 | ||
12.14.6.2. Surface-Sediment Calibrations | 6878 | ||
12.14.7. Conclusion | 6883 | ||
References | 6884 | ||
Chapter 12.15: Biomarkers for Terrestrial Plants and Climate | 6888 | ||
12.15.1. Higher Plants Biomarkers | 6888 | ||
12.15.1.1. n-Alkyl Compounds in Plant Cuticle | 6888 | ||
12.15.1.1.1. n-Alkane concentrations in leaves of vascular plants | 6889 | ||
12.15.1.1.2. n-Alkane distributions: average chain length | 6889 | ||
12.15.1.1.3. n-Alkane distributions: carbon preference index | 6891 | ||
12.15.1.1.4. Alkyl compounds and aliphatic carbon preservation | 6891 | ||
12.15.1.1.5. Other sources for n-alkyl compounds | 6892 | ||
12.15.1.2. Terpenoids | 6892 | ||
12.15.1.3. Macromolecular Components of Vascular Plants: Lignin and Related Structures | 6894 | ||
12.15.2. Soil and Lake Microbial Lipids and Proxies for Terrestrial Paleoclimate | 6895 | ||
12.15.3. Carbon Isotope Signatures of Vegetation and Climate | 6896 | ||
12.15.3.1. Carbon Isotopic Compositions of C3 Plants | 6897 | ||
12.15.3.1.1. Sensitivity of C3 photosynthetic isotope fractionation to water | 6897 | ||
12.15.3.1.2. Sun exposure, growth rates, and the canopy effect | 6898 | ||
12.15.3.1.3. Atmospheric CO2 concentrations and plant delta13C &INS id | 6898 | ||
12.15.3.1.4. Fractionation and atmospheric CO2 concentration | 6899 | ||
12.15.3.2. Carbon Isotopic Composition of C4 Plants | 6899 | ||
12.15.3.3. C4 Evolution, Ecosystems, and Past Climates | 6899 | ||
12.15.3.4. Woodland-Grassland Ecosystems and C3/C4 Mixing Models | 6900 | ||
12.15.4. Lipid-Leaf Fractionation Factors | 6900 | ||
12.15.5. Transport and Preservation in Soils, Lakes, and Marine Sediments | 6901 | ||
12.15.5.1. Transport Means and Depositional Setting | 6901 | ||
12.15.5.2. The Impact of Fire on Aeolian Inputs | 6901 | ||
12.15.5.3. Catchment and Landscape Dynamics: Hydrology, Topography, Vegetation, and Erosion | 6902 | ||
12.15.5.4. Soil and Shelf Residence Time | 6902 | ||
12.15.6. Terrestrial Biomarkers and Isotopes: Research Outlook | 6902 | ||
Acknowledgments | 6902 | ||
References | 6902 | ||
e9780080983004v13 | 6910 | ||
Front Cover | 6910 | ||
Geochemistry of Mineral Depsoits | 6913 | ||
Copyright | 6914 | ||
IN MEMORIAM | 6915 | ||
HEINRICH DIETER HOLLAND (1927–2012) | 6917 | ||
KARL KAREKIN TUREKIAN (1927–2013) | 6919 | ||
DEDICATION | 6923 | ||
CONTENTS | 6925 | ||
EXECUTIVE EDITORS’ FOREWORD TO THE SECOND EDITION | 6927 | ||
CONTRIBUTORS | 6931 | ||
VOLUME EDITOR’S INTRODUCTION | 6933 | ||
Chapter 13.1: Fluids and Ore Formation in the Earths Crust | 6937 | ||
13.1.1. Ore Deposits and Crustal Geochemistry | 6938 | ||
13.1.1.1. Ores, Mineral Deposits, and Crustal Composition | 6938 | ||
13.1.1.2. Economic Aspects: Deposit Assessment, Grade, and Resources and Reserves | 6938 | ||
13.1.1.3. Extreme Metal Enrichment: A Rare Conjunction of Common Processes | 6940 | ||
13.1.2. Magmatic Ore Formation | 6941 | ||
13.1.3. Ore-Forming Hydrothermal Processes | 6944 | ||
13.1.3.1. Physical Aspects of Hydrothermal Metal Enrichment | 6945 | ||
13.1.3.1.1. Energy sources driving fluid flow | 6945 | ||
13.1.3.1.2. Fluid focusing and `structural control´ | 6946 | ||
13.1.3.1.3. Timescales of fluid flow and heat transfer | 6946 | ||
13.1.3.2. Chemical Driving Forces for Hydrothermal Metal Enrichment | 6947 | ||
13.1.3.2.1. Rock buffering and fluid-chemical master variables | 6947 | ||
13.1.3.2.2. Sulfide solubility and base-metal content of crustal fluids | 6948 | ||
13.1.3.2.3. Example: Hydrothermal redistribution of gold | 6950 | ||
13.1.3.3. Metal Source Regions versus Ore Deposition Sites | 6951 | ||
13.1.3.3.1. Source region characteristics | 6951 | ||
13.1.3.3.2. Ore deposition and wall-rock alteration | 6951 | ||
13.1.4. Hydrothermal Ore Formation in Sedimentary Basins | 6952 | ||
13.1.5. Hydrothermal Ore Systems in the Oceanic Realm | 6953 | ||
13.1.6. Magmatic-Hydrothermal Ore Systems | 6953 | ||
13.1.6.1. Melt Generation, Magma Storage, and Ascent | 6953 | ||
13.1.6.2. Fluid Exsolution and Element Transfer from Melt to Fluid | 6955 | ||
13.1.6.3. Fluid Cooling, Decompression, and Phase Separation | 6955 | ||
13.1.6.4. Fluid Evolution Paths at the Porphyry to Epithermal Transition | 6956 | ||
13.1.6.5. Wall-Rock Alteration and Ore Mineral Precipitation | 6957 | ||
13.1.7. Ore Formation at the Earth's Surface | 6958 | ||
13.1.8. Back to the Future: Global Mineral Resources | 6959 | ||
Acknowledgments | 6959 | ||
References | 6959 | ||
Chapter 13.2: The Chemistry of Metal Transport and Deposition by Ore-Forming Hydrothermal Fluids | 6965 | ||
13.2.1. Introduction | 6965 | ||
13.2.1.1. Compositions of Ore-Forming Fluids | 6966 | ||
13.2.2. Hydrothermal Ore Solution Chemistry - The Main Dissolved Components | 6969 | ||
13.2.2.1 Water Solvent at Hydrothermal Conditions | 6969 | ||
13.2.2.2 NaCl – The Main Dissolved Electrolyte Component | 6971 | ||
13.2.2.3 Ion Hydration, Association, and Water Activity | 6971 | ||
13.2.2.4 Weak Acid/Base Equilibria in Hydrothermal Systems | 6972 | ||
13.2.3. Mineral Solubility in Water and Salt Solutions at High Temperature and Pressure | 6973 | ||
13.2.4. Ore Metal Transport and Deposition | 6976 | ||
13.2.4.1. Ore Fluids with Liquid-Like Densities | 6976 | ||
13.2.4.1.1. Ligands in hydrothermal ore solutions | 6976 | ||
13.2.4.1.2. Metal chloride complexing | 6977 | ||
13.2.4.1.3. Complexing with other halide ligands | 6978 | ||
13.2.4.1.4. Metal complexes with hydroxide and other oxygen electron donor ligands | 6979 | ||
13.2.4.1.5. Complexing with hydrosulfide/sulfide ligands | 6981 | ||
13.2.4.1.6. Thioanions | 6982 | ||
13.2.4.1.7. Complexing with other sulfur-containing ligands | 6983 | ||
13.2.4.1.8. Other complexing ligands | 6984 | ||
13.2.4.1.9. Ore fluids with gas-like density | 6984 | ||
13.2.5. Epilogue | 6986 | ||
Acknowledgments | 6986 | ||
References | 6986 | ||
Chapter 13.3: Stable Isotope Geochemistry of Mineral Deposits | 6995 | ||
13.3.1. Introduction | 6995 | ||
13.3.2. Fundamental Aspects of Stable Isotope Geochemistry | 6995 | ||
13.3.3. Stable Isotope Systematics | 6996 | ||
13.3.3.1. Delta Notation | 6996 | ||
13.3.3.2. Fractionation Equations | 6996 | ||
13.3.3.3. Reservoir Effects and Mass Balance Calculations | 6997 | ||
13.3.3.4. Water/Rock Ratio | 6998 | ||
13.3.4. Analytical Methods | 6998 | ||
13.3.5. Ore Deposit Types | 6998 | ||
13.3.5.1. Magmatic Sulfide and PGE Deposits | 6998 | ||
13.3.5.2. Porphyry Deposits | 6999 | ||
13.3.5.3. Skarn Deposits | 7001 | ||
13.3.5.4. Volcanogenic Massive Sulfide Deposits | 7004 | ||
13.3.5.5. Sedimentary-Exhalative and Mississippi Valley-Type Deposits | 7011 | ||
13.3.5.6. Precious-Metal Deposits: Epithermal, Carlin-Type, and Orogenic Au | 7013 | ||
13.3.5.7. Banded Iron Formation | 7014 | ||
13.3.6. Summary and Conclusions | 7016 | ||
Acknowledgments | 7018 | ||
References | 7018 | ||
Chapter 13.4: Dating and Tracing the History of Ore Formation | 7023 | ||
13.4.1. A Holistic Approach to Ore Geology | 7024 | ||
13.4.1.1. What We Know | 7024 | ||
13.4.1.2. Critical and Compromising Gaps | 7025 | ||
13.4.1.3. Meaningful Results | 7025 | ||
13.4.1.4. Meaningful Models | 7026 | ||
13.4.2. The Fourth Dimension - Time | 7026 | ||
13.4.2.1. The Transcendence of Time | 7026 | ||
13.4.2.2. Time Ties that Bind | 7027 | ||
13.4.3. Radiometric Clocks | 7027 | ||
13.4.3.1. What Geologic Clocks Measure | 7027 | ||
13.4.3.2. How Radiometric Clocks Work | 7028 | ||
13.4.3.2.1. Ages through single mineral clocks | 7029 | ||
13.4.3.2.2. Ages through isochrons | 7030 | ||
13.4.3.2.3. Conditions for isotopic closure | 7030 | ||
13.4.3.2.3.1. Thermal dependence | 7031 | ||
13.4.3.2.3.2. Chemical dependence | 7032 | ||
13.4.4. Radiometric Clocks for Ore Geology | 7033 | ||
13.4.4.1 U–Th–Pb (Uranium–Thorium–Lead) | 7033 | ||
13.4.4.1.1. Zircon (ZrSiO4) | 7033 | ||
13.4.4.1.2. Monazite (Ce, LREE, Th, U, Ca)PO4 | 7034 | ||
13.4.4.1.3. Uraninite (UO2) | 7034 | ||
13.4.4.2. Pb-Pb (Lead-Lead) | 7034 | ||
13.4.4.3. Pt-Os (Platinum-Osmium) | 7036 | ||
13.4.4.4. Rb-Sr (Rubidium-Strontium) | 7036 | ||
13.4.4.5. 40Ar/39Ar (Argon-Argon) | 7036 | ||
13.4.4.6. Sm-Nd (Samarium-Neodymium) | 7037 | ||
13.4.5 Rhenium–Osmium – A Clock for Sulfides | 7037 | ||
13.4.5.1. Where We Find Re and Os | 7037 | ||
13.4.5.2. Historical Background | 7037 | ||
13.4.5.2.1. Sample-spike equilibration | 7037 | ||
13.4.5.2.2 Negative TIMS | 7037 | ||
13.4.5.2.3. An interlaboratory age standard | 7038 | ||
13.4.5.2.4. Half-life hurdles - the 187Re decay constant | 7038 | ||
13.4.5.2.5. Stoichiometry of Os standards | 7038 | ||
13.4.5.3. The Last Steps for the First Chronometer for Ore Deposition | 7039 | ||
13.4.5.3.1. Comparison of Re-Os ages with other ages | 7039 | ||
13.4.5.3.2. Lack of effect of alteration on Re-Os systematics | 7039 | ||
13.4.5.3.3. Parent-daughter decoupling conundrum in molybdenite | 7039 | ||
13.4.5.3.4. Revival of model ages for geochronometry | 7040 | ||
13.4.5.4. Molybdenite Dating of a Young Porphyry Cu-(Mo) Deposit in Chile | 7040 | ||
13.4.5.4.1. Full circle back to the field | 7043 | ||
13.4.5.5. Molybdenite Dating of an Old Porphyry Cu-(Mo) Deposit in Northern Sweden | 7044 | ||
13.4.5.6. Low Level, Highly Radiogenic (LLHR) Sul | 7045 | ||
13.4.6. Re-Os in Nonsulfides | 7046 | ||
13.4.6.1. Chromite (FeCr2O4) | 7046 | ||
13.4.6.2. Magnetite (Fe3O4) | 7047 | ||
13.4.6.3. Native Gold | 7047 | ||
13.4.7. A Clock for Metal Release and Migration from Hydrocarbon Maturation | 7047 | ||
13.4.8. Future of Dating for Ore Geology and Mineral Exploration | 7048 | ||
Acknowledgments | 7049 | ||
References | 7049 | ||
Chapter 13.5: Fluid Inclusions in Hydrothermal Ore Deposits | 7055 | ||
13.5.1. Introduction | 7056 | ||
13.5.2. Mississippi Valley-Type Deposits | 7056 | ||
13.5.2.1. Composition of MVT Fluids | 7057 | ||
13.5.2.1.1. Major cations | 7057 | ||
13.5.2.1.2. Ore metals | 7057 | ||
13.5.2.2. MVT Fluid Sources | 7058 | ||
13.5.3. Volcanogenic Massive Sulfide (VMS) Deposits | 7058 | ||
13.5.3.1. General Fluid Characteristics | 7058 | ||
13.5.3.2. Composition of VMS Fluids | 7058 | ||
13.5.3.3. VMS Fluid Sources | 7059 | ||
13.5.4. Epithermal Gold and Silver Deposits | 7059 | ||
13.5.4.1. Overall Temperature and Salinity Ranges of Epithermal Fluids | 7060 | ||
13.5.4.2. Compositional Variations of Epithermal Fluids | 7060 | ||
13.5.4.2.1. Sulfidation state | 7060 | ||
13.5.4.2.2. Correlation between metals in ore and fluid salinity | 7060 | ||
13.5.4.2.3. Volatiles | 7061 | ||
13.5.4.2.4. Depth of formation | 7061 | ||
13.5.4.2.5. Source of mineralizing fluids | 7061 | ||
13.5.5. Porphyry Cu Deposits | 7062 | ||
13.5.5.1. Homogenization Temperature and Salinity Data | 7062 | ||
13.5.5.1.1. Variation in Th and salinity with alteration and mineralization | 7063 | ||
13.5.5.2. Compositions of Individual Fluid Inclusions | 7064 | ||
13.5.5.2.1. Copper content of ore fluids | 7064 | ||
13.5.5.3. Depth and Pressure of Ore Formation | 7065 | ||
13.5.6. Porphyry Mo Deposits | 7065 | ||
13.5.6.1. Homogenization Temperature and Salinity Data | 7065 | ||
13.5.6.2. Depth and Pressure of Ore Formation | 7066 | ||
13.5.6.3. Source of Metals in Porphyry Mo Deposits | 7066 | ||
13.5.7. Porphyry Sn-W Deposits | 7066 | ||
13.5.7.1. General Fluid Characteristics | 7066 | ||
13.5.7.2. Fluid Composition | 7067 | ||
13.5.7.3. Fluid Source | 7067 | ||
13.5.7.4. Precipitation Mechanism | 7067 | ||
13.5.8. Skarn Deposits | 7068 | ||
13.5.8.1. Homogenization Temperature and Salinity Data | 7068 | ||
13.5.8.2. Sources of Fluids | 7069 | ||
13.5.8.3. Pressures and Depths of Mineralization | 7069 | ||
13.5.9. Carlin-Type Au Deposits | 7069 | ||
13.5.9.1. Homogenization Temperature, Salinity, and Volatile Data | 7070 | ||
13.5.9.2. Pressure and Depth of Formation | 7070 | ||
13.5.9.3. Source of Mineralizing Fluids | 7070 | ||
13.5.10. Orogenic Gold Deposits | 7070 | ||
13.5.10.1. Compositional and Microthermometric Data | 7070 | ||
13.5.10.2. Compositions of Orogenic Gold Fluids | 7070 | ||
13.5.10.2.1. Major ions | 7071 | ||
13.5.10.2.2. Volatile components | 7071 | ||
13.5.10.3. Evidence of Immiscibility | 7072 | ||
13.5.10.4. Depths of Formation | 7072 | ||
13.5.10.5. Sources of Mineralizing Fluids | 7072 | ||
13.5.11. Concluding Remarks and Future Directions | 7073 | ||
Acknowledgments | 7073 | ||
References | 7073 | ||
Chapter 13.6: Melt Inclusions | 7079 | ||
13.6.1. Introduction | 7079 | ||
13.6.2. Formation of Melt Inclusions | 7080 | ||
13.6.3. Postentrapment Changes in Melt Inclusions | 7081 | ||
13.6.4. Analytical Techniques | 7084 | ||
13.6.4.1. Melt-Inclusion Rehomogenization | 7084 | ||
13.6.4.2. Electron Microprobe Analysis | 7085 | ||
13.6.4.3. Secondary Ion Mass Spectrometry | 7086 | ||
13.6.4.4. Laser Ablation ICP-MS | 7086 | ||
13.6.4.5. Spectroscopic Techniques | 7087 | ||
13.6.5. Information Obtainable from Melt Inclusions | 7087 | ||
13.6.5.1. Coexistence with other Phases | 7087 | ||
13.6.5.1.1. Immiscibility between silicate melt and aqueous fluid | 7089 | ||
13.6.5.1.2. Immiscibility between silicate melt and salt melts | 7089 | ||
13.6.5.1.3. Silicate melt-silicate melt immiscibil | 7090 | ||
13.6.5.2. Concentration of Volatiles | 7091 | ||
13.6.5.3. Constraints on P-T-X Conditions | 7091 | ||
13.6.5.4. Concentrations of Ore Metals | 7094 | ||
13.6.5.5. Melt Evolution during Magma Chamber Processes | 7094 | ||
13.6.5.6. Isotopic Information | 7095 | ||
13.6.6. Melt Inclusions in Mineralized Systems | 7095 | ||
13.6.6.1. Porphyry-Type Ore Deposits | 7095 | ||
13.6.6.1.1. Porphyry Cu (-Au, Mo) Deposits | 7095 | ||
13.6.6.1.2. Porphyry Mo deposits | 7100 | ||
13.6.6.1.3. Porphyry Sn deposits | 7100 | ||
13.6.6.2. Intrusion-Related Vein, Skarn, and Greisen Deposits | 7101 | ||
13.6.6.2.1. Sn-W deposits | 7101 | ||
13.6.6.2.2. Other intrusion-related ore deposits | 7101 | ||
13.6.6.3. Magmatic Oxide and Sulfide Deposits | 7102 | ||
13.6.6.4. Volcanogenic Massive Sulfide Deposits | 7102 | ||
13.6.6.5. Pegmatites | 7102 | ||
13.6.6.6. Alkali Complexes and Carbonatites | 7103 | ||
13.6.7. Synthesis and Conclusions | 7103 | ||
Acknowledgments | 7104 | ||
References | 7104 | ||
Chapter 13.7: Metamorphosed Hydrothermal Ore Deposits | 7111 | ||
13.7.1. Introduction | 7111 | ||
13.7.2. Characteristics of Metamorphosed Hydrothermal Ore Systems | 7112 | ||
13.7.2.1. Terminology | 7112 | ||
13.7.2.2. Hydrothermal Ore Deposits and Alteration Haloes | 7112 | ||
13.7.2.3. Effects of Metamorphism on Ore and Host Rocks | 7115 | ||
13.7.2.4. Lithological and Mineralogical Attributes of Metamorphosed Hydrothermal Systems | 7116 | ||
13.7.2.4.1. Metaexhalite, metainhalite, and ironstone | 7116 | ||
13.7.2.4.2. Hydrothermal alteration haloes | 7117 | ||
13.7.2.4.3. Mineral compositions as indicators | 7118 | ||
13.7.2.5. Remobilization and Melting as Agents of Chemical Transformation of Ore Systems | 7118 | ||
13.7.2.5.1. Remobilization of preexisting ore bodies | 7118 | ||
13.7.2.5.2. Melting of ore deposits | 7118 | ||
13.7.3. Geochemical Techniques Used to Study Metamorphosed Ore Deposits | 7119 | ||
13.7.3.1. Lithogeochemistry | 7119 | ||
13.7.3.2. Metamorphic Fluids and Fluid Inclusion Studies | 7119 | ||
13.7.3.3. Behavior of Light Stable Isotopes during Metamorphism | 7121 | ||
13.7.3.3.1. Sulfur | 7121 | ||
13.7.3.3.2. Boron | 7122 | ||
13.7.3.3.3. Oxygen | 7122 | ||
13.7.3.4. Behavior of Nontraditional Stable Isotopes during Metamorphism | 7122 | ||
13.7.4. From Case Examples to Conceptual Models and Exploration Tools | 7123 | ||
13.7.4.1. Vectoring to Ore through Metamorphic Petrology Applications | 7123 | ||
13.7.4.1.1. Protolithology as an essential step of lithogeochemical exploration | 7123 | ||
13.7.4.1.2. Chemographic approaches in the recognition of metamorphosed hydrothermal alteration | 7123 | ||
13.7.4.1.3. Application to metaaluminous and peraluminous gneisses | 7124 | ||
13.7.4.2. Knowledge, Knowledge Gaps, and the Importance of Geochemical Haloes | 7125 | ||
13.7.5. Conclusions | 7126 | ||
Acknowledgments | 7126 | ||
References | 7126 | ||
Chapter 13.8: Geochemistry of Magmatic Ore Deposits | 7131 | ||
13.8.1. Introduction | 7131 | ||
13.8.2. Trace Element Behavior | 7132 | ||
13.8.3. Fertility of Primary Magmas | 7133 | ||
13.8.4. Incompatible Element Deposits | 7136 | ||
13.8.4.1. Rare-Element Granites, Syenites, and Pegmatites | 7136 | ||
13.8.4.2. Carbonatite | 7137 | ||
13.8.5. Compatible Lithophile Element Deposits | 7138 | ||
13.8.5.1. Chromitite | 7138 | ||
13.8.5.2. Stratiform Magnetitite | 7140 | ||
13.8.5.3. Kiruna-Type Ores and Nelsonites | 7140 | ||
13.8.5.4. Tellnes-Type Ti Deposits | 7141 | ||
13.8.6. Magmatic Chalcophile Element Deposits | 7141 | ||
13.8.6.1. Sulfide Liquid Immiscibility | 7141 | ||
13.8.6.2. Sulfide Solubility | 7141 | ||
13.8.6.3. Chalcophile Element Partitioning | 7142 | ||
13.8.6.4. Sulfide Segregation | 7142 | ||
13.8.6.5. Crystallization of Sulfide Magmas | 7146 | ||
13.8.6.6. Precious Metal Sulfide Deposits | 7147 | ||
13.8.6.6.1. Classification | 7147 | ||
13.8.6.6.2. Offset reefs | 7148 | ||
13.8.6.6.3. Unconformity-hosted reefs | 7148 | ||
13.8.7. Conclusions | 7150 | ||
Acknowledgments | 7151 | ||
References | 7151 | ||
Chapter 13.9: Sediment-Hosted Zinc-Lead Mineralization: Proce | 7155 | ||
13.9.1. Introduction | 7156 | ||
13.9.2. Sedimentary `Exhalative&INS id= | 7157 | ||
13.9.2.1. Introduction | 7157 | ||
13.9.2.2. Tectonostratigraphic Setting | 7157 | ||
13.9.2.3. Structural Setting | 7157 | ||
13.9.2.4. Mineralization | 7158 | ||
13.9.2.5. Fluid Sources | 7160 | ||
13.9.2.6. Metal and Sulfur Sources | 7161 | ||
13.9.2.7. Secular Variation in the Abundance of SEDEX Deposits | 7162 | ||
13.9.2.8. Fluid Flow Mechanism | 7163 | ||
13.9.2.9. Genetic Model | 7164 | ||
13.9.3. Mississippi Valley-Type Mineralization | 7164 | ||
13.9.3.1. Introduction | 7164 | ||
13.9.3.2. Tectonostratigraphic Setting | 7165 | ||
13.9.3.3. Structural Setting | 7165 | ||
13.9.3.4. Mineralization | 7165 | ||
13.9.3.5. Fluid Sources | 7166 | ||
13.9.3.6. Metal and Sulfur Sources | 7167 | ||
13.9.3.7. Secular Variation in the Abundance of MVT Deposits | 7168 | ||
13.9.3.8. Fluid Flow Mechanism | 7169 | ||
13.9.3.9. Genetic Model | 7170 | ||
13.9.4. Irish-Type Zn-Pb Mineralization: A Transitional Ore Type | 7171 | ||
13.9.4.1. Introduction | 7171 | ||
13.9.4.2. Tectonostratigraphic Setting | 7172 | ||
13.9.4.3. Structural Setting | 7174 | ||
13.9.4.4. Mineralization | 7174 | ||
13.9.4.5. Fluid Sources | 7175 | ||
13.9.4.6. Metal and Sulfur Sources | 7176 | ||
13.9.4.7. Timing of Mineralization | 7177 | ||
13.9.4.8. Fluid Flow Mechanism | 7178 | ||
13.9.4.9. Genetic Model | 7178 | ||
13.9.5. Discussion | 7179 | ||
13.9.5.1. Irish Deposits as Carbonate-Replacement SEDEX Systems | 7179 | ||
13.9.5.2. Key Factors in the Genesis of SEDEX Deposits | 7179 | ||
13.9.5.3. Key Factors in the Genesis of MVT Deposits | 7180 | ||
13.9.5.4. Outstanding Questions | 7180 | ||
Acknowledgments | 7180 | ||
References | 7181 | ||
Chapter 13.10: Low-Temperature Sediment-Hosted Copper Deposits | 7187 | ||
13.10.1. Introduction | 7187 | ||
13.10.2. Geochemistry in the Genesis of SSC Minera | 7188 | ||
13.10.2.1. Characteristics of SSCs | 7188 | ||
13.10.2.2. A General Genetic Model for SSC Copper Deposition | 7191 | ||
13.10.2.2.1. Early concepts | 7191 | ||
13.10.2.2.2. A diagenetic explanation for SSCs | 7191 | ||
13.10.2.3. Copper Solubility | 7194 | ||
13.10.2.4. Solubilities of other Ore-Stage Metals | 7196 | ||
13.10.2.5. Sources of Copper | 7196 | ||
13.10.2.6. A Basin-Scale Genetic Model for SSCs | 7198 | ||
13.10.3. Closely Related Sediment-Hosted Copper Deposits | 7199 | ||
13.10.3.1. Redbed-Type Copper Deposits | 7199 | ||
13.10.3.2. Volcanic-Associated Redbed Deposits | 7200 | ||
13.10.4. Distantly Related Sediment-Hosted Deposit Types | 7201 | ||
13.10.5. Concluding Remarks | 7204 | ||
Acknowledgments | 7204 | ||
References | 7204 | ||
Chapter 13.11: Deep-Ocean Ferromanganese Crusts and Nodules | 7209 | ||
13.11.1. Introduction | 7209 | ||
13.11.1.1. Definitions and General Mechanisms of Formation | 7209 | ||
13.11.1.2. Distribution | 7210 | ||
13.11.1.3. Physical Properties | 7211 | ||
13.11.1.4. Review of Nodule and Crust Compositions | 7212 | ||
13.11.1.4.1. Mineralogy | 7212 | ||
13.11.1.4.2. Chemical composition | 7212 | ||
13.11.1.5. Age Dating | 7216 | ||
13.11.2. New Considerations | 7216 | ||
13.11.2.1. Concentrations of High-Tech Trace Metals in Nodules and Crusts | 7216 | ||
13.11.2.1.1. High field-strength elements, Ti, Zr, Hf, Nb, and & | 7216 | ||
13.11.2.1.2. Noble metals | 7216 | ||
13.11.2.1.3. REEs and yttrium | 7216 | ||
13.11.2.1.4. Vanadium, molybdenum, and tungsten | 7217 | ||
13.11.2.1.5. Tellurium | 7217 | ||
13.11.2.2. Mechanisms of Formation with Focus on High-Tech Metals | 7217 | ||
13.11.2.3. Biomineralization as a Mechanism of Crust and Nodule Formation | 7220 | ||
13.11.3. Paleoceanographic Records from Fe-Mn Crusts and Nodules | 7221 | ||
13.11.4. Exploration, Technology, and Resource Considerations | 7222 | ||
13.11.4.1. Mining Systems | 7222 | ||
13.11.4.2. Resources | 7222 | ||
13.11.4.3. Environmental Issues | 7223 | ||
13.11.5. Future Directions | 7224 | ||
Acknowledgments | 7225 | ||
References | 7225 | ||
Chapter 13.12: Geochemistry of a Marine Phosphate Deposit: A Signpost to Phosphogenesis | 7229 | ||
13.12.1. Introduction | 7229 | ||
13.12.2. Statement of the Problem | 7229 | ||
13.12.3. The MPM: Local Setting | 7231 | ||
13.12.4. Lithogenous Sediment Fraction | 7234 | ||
13.12.5. Seawater-Derived Trace Elements | 7236 | ||
13.12.5.1. Biogenous Trace Elements | 7238 | ||
13.12.5.2. Hydrogenous Trace Elements | 7238 | ||
13.12.5.3. A Circulation Model | 7241 | ||
13.12.6. Rare Earth Elements | 7242 | ||
13.12.7. Summary and Conclusions | 7244 | ||
Acknowledgments | 7245 | ||
References | 7245 | ||
Chapter 13.13: Sedimentary Hosted Iron Ores | 7249 | ||
13.13.1. Introduction | 7249 | ||
13.13.2. Definition and Classification of Iron-Formation | 7251 | ||
13.13.2.1. Precambrian Iron-Formation | 7252 | ||
13.13.2.2. Occurrence and Distribution of Precambrian Iron-Formation | 7253 | ||
13.13.2.3. BIF Ores | 7254 | ||
13.13.2.4. Mineralogy | 7255 | ||
13.13.2.5. Chemical Composition | 7255 | ||
13.13.2.6. Beneficiation of BIF/GIF | 7256 | ||
13.13.3. Enriched BIF-Hosted Iron Ores | 7256 | ||
13.13.3.1. Residual BIF-Hosted Iron Ore | 7257 | ||
13.13.3.2. Martite-Goethite BIF-Hosted Iron Ore | 7257 | ||
13.13.3.3. Martite Microplaty Hematite BIF-Hosted Iron Ore | 7258 | ||
13.13.3.3.1. Genetic models | 7260 | ||
13.13.3.4. Weathering of Martite-Goethite and Hematite BIF-hosted Iron Ores | 7265 | ||
13.13.3.5. Geochemistry of Martite-Goethite and Martite-microplaty Hematite Iron Ores | 7267 | ||
13.13.3.5.1. Major and minor elements | 7268 | ||
13.13.3.5.2. Rare earth elements | 7268 | ||
13.13.3.6. Detrital Iron Deposits | 7268 | ||
13.13.4. Ooidal Ironstones | 7270 | ||
13.13.4.1. Introduction | 7270 | ||
13.13.4.2. POI Genesis | 7271 | ||
13.13.4.2.1. Marine ironstones | 7271 | ||
13.13.4.2.2. Terrestrial ironstones | 7273 | ||
13.13.4.3. Comparative Evaluation of POI-Derived Iron Ore Mineralogy and Composition | 7275 | ||
13.13.4.4. Bog Iron Ores | 7279 | ||
13.13.4.4.1. General occurrence setting and early utilization | 7279 | ||
13.13.4.4.2. Bog iron ore exploitation | 7279 | ||
13.13.4.4.3. Types of bog iron ores | 7280 | ||
13.13.4.4.3.1. Lake ores | 7280 | ||
13.13.4.4.3.1.1. Stream/spring bog ores | 7280 | ||
13.13.4.4.3.2. Marsh or peat bog ores | 7281 | ||
13.13.4.4.3.2.1. Blackband ironstones | 7281 | ||
13.13.4.4.3.3. Bacterial association in bog ores | 7281 | ||
13.13.4.4.3.4. Bog ore iron oxides - mineralogy | 7283 | ||
13.13.5. Summary | 7284 | ||
Acknowledgments | 7285 | ||
References | 7285 | ||
Chapter 13.14: Geochemistry of Porphyry Deposits | 7293 | ||
13.14.1. Introduction | 7293 | ||
13.14.2. Geology, Alteration, and Mineralization | 7293 | ||
13.14.3. Tectonic Setting | 7296 | ||
13.14.4. Igneous Petrogenesis | 7296 | ||
13.14.5. Geochronology | 7299 | ||
13.14.6. Lead Isotopes | 7300 | ||
13.14.7. Fluid Inclusions | 7302 | ||
13.14.8. Conventional Stable Isotopes | 7303 | ||
13.14.8.1. Oxygen-Deuterium | 7303 | ||
13.14.8.2. Sulfur | 7303 | ||
13.14.8.3. Carbon-Oxygen | 7306 | ||
13.14.9. Nontraditional Stable Isotopes | 7306 | ||
13.14.9.1. Copper | 7306 | ||
13.14.9.2. Molybdenum | 7308 | ||
13.14.9.3. Iron | 7309 | ||
13.14.9.4. Summary | 7309 | ||
13.14.10. Ore-Forming Processes | 7309 | ||
13.14.11. Exploration Model | 7311 | ||
Acknowledgments | 7312 | ||
References | 7312 | ||
Chapter 13.15: Geochemistry of Hydrothermal Gold Deposits | 7319 | ||
13.15.1. Introduction | 7319 | ||
13.15.2. Epithermal Deposits | 7320 | ||
13.15.2.1. Introduction | 7320 | ||
13.15.2.2. Low-Sulfidation Epithermal Deposits | 7321 | ||
13.15.2.2.1. Trace elements and mineral associations | 7321 | ||
13.15.2.2.2. Ore fluid characteristics | 7322 | ||
13.15.2.2.3. Hydrothermal alteration | 7322 | ||
13.15.2.2.4. Geochemistry of the Midas LS deposit, Nevada | 7323 | ||
13.15.2.3. High-Sulfidation Epithermal Ores | 7326 | ||
13.15.2.3.1. Hydrothermal alteration | 7326 | ||
13.15.2.3.2. Trace elements and mineral associations | 7327 | ||
13.15.2.3.3. Ore-fluid composition | 7328 | ||
13.15.2.3.4. Light-stable isotopes | 7329 | ||
13.15.2.3.5. Reaction of volcanic gas condensate with quartz latite | 7329 | ||
13.15.2.3.6. Ore deposition | 7330 | ||
13.15.2.4. New Ideas about the Geochemistry of Epithermal Deposits | 7333 | ||
13.15.3. Carlin-Type Gold Deposits | 7334 | ||
13.15.3.1. Introduction | 7334 | ||
13.15.3.2. Age and Geologic Setting of CTD | 7335 | ||
13.15.3.3. Ore and Gangue Minerals | 7335 | ||
13.15.3.4. Geochemistry of Rocks and Pyrite | 7336 | ||
13.15.3.5. Composition of Ore Fluids | 7340 | ||
13.15.3.6. Carbonate Dissolution | 7340 | ||
13.15.3.7. Ore Fluid Composition and Precipitation Mechanisms | 7341 | ||
13.15.3.8. Element Substitution in Pyrite | 7342 | ||
13.15.3.9. Source(s) of Ore Fluid Components | 7343 | ||
13.15.4. Orogenic Gold Deposits | 7345 | ||
13.15.4.1. Introduction | 7345 | ||
13.15.4.1.1. General geologic setting and genetic model | 7345 | ||
13.15.4.2. Geochemistry and Mineralogy of Alteration and Ores | 7347 | ||
13.15.4.2.1. Ore mineral assemblages | 7347 | ||
13.15.4.2.2. Wall rock alteration | 7348 | ||
13.15.4.2.3. Geochemistry of ore-forming fluids | 7349 | ||
13.15.4.2.4. PTX constraints on ore deposition | 7351 | ||
13.15.4.3. Geochemistry of Type Examples | 7352 | ||
13.15.4.3.1. Phanerozoic metasedimentary-hosted Muruntau | 7352 | ||
13.15.4.3.2. Paleoproterozoic BIF-hosted Homestake | 7353 | ||
13.15.4.3.3. Late Archean Greenstone-hosted Golden Mile | 7354 | ||
13.15.5. Summary and Conclusions | 7354 | ||
Acknowledgments | 7355 | ||
References | 7355 | ||
Chapter 13.16: Silver Vein Deposits | 7361 | ||
13.16.1. Introduction | 7361 | ||
13.16.2. Silver-Lead-Zinc Veins | 7361 | ||
13.16.2.1. Cordilleran Vein Type Deposits | 7361 | ||
13.16.2.2 Silver–Lead–Zinc Veins in Clastic Metasedimentary Terranes | 7362 | ||
13.16.3 Five-Element (Ag–Ni–Co–As–Bi) Veins | 7362 | ||
13.16.4 Epithermal Ag–Au and Ag–Base Metal Veins | 7364 | ||
13.16.4.1. Low-Sulfidation Epithermal Deposi | 7364 | ||
13.16.4.2. High-Sulfidation Epithermal Depo | 7365 | ||
13.16.5. Silver-Bearing Veins Related to Tin Mineralization | 7366 | ||
13.16.6. Silver-Bearing Veins Related to Skarn Mineralization | 7366 | ||
13.16.7. Discussion | 7366 | ||
Acknowledgments | 7367 | ||
References | 7367 | ||
Chapter 13.17: Geochemistry of Placer Gold - A Case Study of the Witwatersrand Deposits | 7369 | ||
13.17.1. Introduction | 7369 | ||
13.17.2. Chemical and Physical Properties of Gold | 7369 | ||
13.17.3. Gold Abundances | 7370 | ||
13.17.4. Gold Compounds and Minerals | 7371 | ||
13.17.5. Aqueous Geochemistry of Gold at 25C | 7371 | ||
13.17.6. Gold in Surficial Environments | 7372 | ||
13.17.6.1. Gold Morphology in Placer Deposits | 7372 | ||
13.17.6.2. Compositional Changes in the Surficial Environment | 7373 | ||
13.17.6.3. Composition of Alluvial and Eluvial Gold Compared to Its Source Characteristics | 7376 | ||
13.17.7. Witwatersrand Gold - A Case Study | 7377 | ||
13.17.7.1. Introduction | 7377 | ||
13.17.7.2. Geological Background | 7377 | ||
13.17.7.3. Formation of the Ore Deposits | 7380 | ||
13.17.7.4. Chemical Composition of Witwatersrand Gold | 7381 | ||
13.17.7.4.1. The nature of the available data | 7381 | ||
13.17.7.4.2 Variation of gold chemistry within the Central Rand Group | 7384 | ||
13.17.7.4.2.1. Witwatersrand gold compositions at the deposit level - the km scale | 7384 | ||
13.17.7.4.2.2 Witwatersrand gold compositions within individual mines/deposits – the km–10 m scale | 7388 | ||
13.17.7.4.2.3. Witwatersrand gold compositions within individual hand specimens &nd | 7388 | ||
13.17.7.4.2.4. Witwatersrand gold compositions within individual thin sections - the cm scale | 7392 | ||
13.17.7.4.2.5. Witwatersrand gold compositions within individual gold grains - the micron scale | 7392 | ||
13.17.7.5. The Origins of Witwatersrand Gold | 7392 | ||
13.17.7.5.1. Gold chemistry and implications for the modified placer and hydrothermal models | 7392 | ||
13.17.7.5.2. Potential gold source regions | 7392 | ||
13.17.8. Conclusions | 7394 | ||
Acknowledgments | 7394 | ||
References | 7394 | ||
Chapter 13.18: Volcanogenic Massive Sulfide Deposits | 7399 | ||
13.18.1. Introduction | 7399 | ||
13.18.2. Distribution, Abundance, and Classification | 7401 | ||
13.18.3. Composition | 7401 | ||
13.18.4. General Genetic Model | 7403 | ||
13.18.5. Chemical Evolution of the Hydrothermal Fluids | 7404 | ||
13.18.5.1. Fluid-Mineral Equilibria | 7404 | ||
13.18.5.2. Metal Concentrations | 7407 | ||
13.18.5.3. Role of Phase Separation | 7408 | ||
13.18.5.4. Redox Controls on Ore Deposition | 7409 | ||
13.18.6. Metal Zoning and Trace Element Geochemistry | 7410 | ||
13.18.6.1. Metal Zoning | 7410 | ||
13.18.6.2. Trace Element Geochemistry | 7412 | ||
13.18.6.3. Sources of Trace Metals | 7413 | ||
13.18.7. Nonsulfide Gangue Minerals | 7414 | ||
13.18.8. Alteration Mineralogy and Geochemistry | 7415 | ||
13.18.9. Chemical Sediments | 7416 | ||
13.18.10. Sulfur Isotopes | 7417 | ||
13.18.11. Oxygen, Hydrogen, and Carbon Isotopes | 7418 | ||
13.18.12. Strontium and Lead Isotopes | 7419 | ||
13.18.13. Conclusions | 7419 | ||
Acknowledgments | 7420 | ||
References | 7421 | ||
Chapter 13.19: Uranium Ore Deposits | 7425 | ||
13.19.1. Introduction | 7425 | ||
13.19.2. The Need for Uranium | 7426 | ||
13.19.3. Geochemistry of Uranium | 7426 | ||
13.19.4. Uranium Deposits Through Time | 7428 | ||
13.19.5. Deposit Types | 7429 | ||
13.19.5.1. Unconformity-Related Deposits | 7429 | ||
13.19.5.1.1. The Athabasca Basin | 7430 | ||
13.19.5.1.2. The Kombolgie Basin | 7433 | ||
13.19.5.1.3. Karku, Russia | 7434 | ||
13.19.5.1.4. Otish Basin, Quebec | 7434 | ||
13.19.5.2. Sandstone Uranium Deposits | 7435 | ||
13.19.5.2.1. United States | 7436 | ||
13.19.5.2.2. Africa | 7437 | ||
13.19.5.2.3. Asia | 7437 | ||
13.19.5.3. Vein Deposits | 7438 | ||
13.19.5.3.1. Beaverlodge, Canada | 7438 | ||
13.19.5.4. Metasomatic Deposits | 7438 | ||
13.19.5.4.1. Na metasomatism-related deposits of | 7439 | ||
13.19.5.4.2. Valhalla, Australia | 7439 | ||
13.19.5.5. Breccia Complex Deposits | 7439 | ||
13.19.5.6. Intrusive Deposits | 7440 | ||
13.19.5.6.1. Alaskites | 7440 | ||
13.19.5.6.2. Peralkaline systems | 7441 | ||
13.19.5.6.2.1. The Ilmaussaq complex | 7441 | ||
13.19.5.6.2.2. Bokan Mountain (United States) | 7441 | ||
13.19.5.6.3. Peraluminous granites | 7441 | ||
13.19.5.7. Volcanic-Associated Deposits | 7442 | ||
13.19.5.7.1. Streltsovkoye caldera (Transbaikalia, Russia) | 7442 | ||
13.19.5.7.2. Macusani, Peru | 7443 | ||
13.19.5.8. Quartz-Pebble Conglomerate Deposits | 7443 | ||
13.19.5.8.1. Blind River-Elliot Lake district | 7443 | ||
13.19.5.8.2. The Witwatersrand Basin | 7444 | ||
13.19.5.9. Surficial Uranium Deposits | 7444 | ||
13.19.5.10. Collapse Breccia Pipe Deposits | 7445 | ||
13.19.5.11. Phosphorite Deposits | 7445 | ||
13.19.5.12. Black Shale and Seawater | 7446 | ||
13.19.6. Synopsis | 7446 | ||
Acknowledgments | 7446 | ||
References | 7446 | ||
Chapter 13.20: Iron Oxide(-Cu-Au-REE-P-Ag-U-Co) Systems | 7451 | ||
13.20.1. Introduction | 7451 | ||
13.20.1.1. Semantics and Postulated Origins | 7452 | ||
13.20.2. Geologic Context for IOCG Systems | 7453 | ||
13.20.2.1. Distribution in Space and Time | 7453 | ||
13.20.2.2. Geologic Settings | 7454 | ||
13.20.2.2.1. Association with igneous rocks (or lack thereof) | 7454 | ||
13.20.2.2.2. Framework lithologies and paleoclimate | 7455 | ||
13.20.3. Synopsis of Deposit Features | 7455 | ||
13.20.3.1. Deposit Types | 7455 | ||
13.20.3.1.1. Magnetite- and/or hematite-dominated deposits | 7456 | ||
13.20.3.1.2. Fe oxide-poor Cu(-Au/Ag) deposits of proposed affinity to IOCG systems | 7458 | ||
13.20.3.1.3. Possible modern analogues | 7458 | ||
13.20.3.2. Grade, Size, and Form | 7458 | ||
13.20.3.3. Ore Mineralogy and Paragenesis | 7459 | ||
13.20.3.4. Minor Element Contents and Mineralogy: U-Th, REE, Co-Ni-V, Cl-F-Br, B | 7460 | ||
13.20.4. Hydrothermal Alteration and System-scale Zoning | 7461 | ||
13.20.4.1. Types of Hydrothermal Alteration | 7461 | ||
13.20.4.1.1. Sodic to calcic alteration types | 7461 | ||
13.20.4.1.2. Carbonate-hosted alteration: Skarn and Fe oxide replacement | 7462 | ||
13.20.4.1.3. K-rich alteration: High-temperature and low-temperature types | 7462 | ||
13.20.4.1.4. Hydrolytic (acid) alteration | 7463 | ||
13.20.4.2. System- to Regional-Scale Spatial and Temporal Patterns | 7463 | ||
13.20.4.3. Extent of Metasomatism and Comparison with Porphyry/Alkaline Cu Systems | 7464 | ||
13.20.5. Petrologic and Geochemical Characteristics | 7465 | ||
13.20.5.1. Conditions of Formation | 7465 | ||
13.20.5.1.1. Depth | 7465 | ||
13.20.5.1.2. Temperature | 7466 | ||
13.20.5.1.3. Fluid inclusion compositions | 7466 | ||
13.20.5.1.4. Oxidation state, sulfidation state, and total sulfur | 7467 | ||
13.20.5.2. Tracer Studies and Sources of Components | 7467 | ||
13.20.5.2.1. Light stable isotopes: H, O, S, C, and B | 7468 | ||
13.20.5.2.2. Radiogenic isotopes: Sr, Nd, Pb, and Os | 7469 | ||
13.20.5.2.3. Halogens and noble gases: Ratios and isotopes | 7470 | ||
13.20.6. Summary of the IOCG Clan, Likely Origins, and the terrestrial Hydrothermal Environment | 7470 | ||
13.20.6.1. Discussion of Genesis | 7471 | ||
13.20.6.2. IOCG Systems and the Terrestrial Hydrothermal Environment | 7472 | ||
Acknowledgments | 7472 | ||
References | 7472 | ||
Chapter 13.21: Geochemistry of the Rare-Earth Element, Nb, Ta, Hf, and Zr Deposits | 7479 | ||
13.21.1. Introduction | 7479 | ||
13.21.1.1. Uses of Rare Elements | 7480 | ||
13.21.1.2. Rare-Element Mineralogy | 7481 | ||
13.21.2. Geochemistry of Rare Elements | 7481 | ||
13.21.2.1. Magmatic Behavior and Processes | 7485 | ||
13.21.2.1.1. Concentrations of rare elements in magmatic rocks | 7485 | ||
13.21.2.1.2. Partial melting and fractional crystallization | 7485 | ||
13.21.2.1.3. Solubility of rare elements in carbonatite melts | 7486 | ||
13.21.2.1.4. Solubility of rare elements in silicate melts | 7486 | ||
13.21.2.1.5. Fluid-melt partitioning of rare elements | 7487 | ||
13.21.2.2. Hydrothermal Behavior and Processes | 7487 | ||
13.21.2.2.1. Concentrations of rare metals in natural fluids | 7487 | ||
13.21.2.2.2. Aqueous complexation and mineral solubility | 7488 | ||
13.21.2.2.2.1. Aqueous complexation of the REE | 7488 | ||
13.21.2.2.2.2. Speciation calculations and REE transport in hydrothermal environments | 7489 | ||
13.21.2.2.3. REE mineral solubility | 7489 | ||
13.21.2.2.4. Zirconium | 7490 | ||
13.21.2.2.5. Tantalum and niobium | 7490 | ||
13.21.3. Deposit Characteristics | 7490 | ||
13.21.3.1. Introduction | 7490 | ||
13.21.3.2. Deposits in Alkaline Igneous Provinces | 7490 | ||
13.21.3.2.1. Carbonatites and genetically related rocks | 7490 | ||
13.21.3.2.2. Silicate-hosted deposits | 7493 | ||
13.21.3.2.2.1. Khibiny and Lovozero | 7493 | ||
13.21.3.2.2.2. Ilimaussaq | 7493 | ||
13.21.3.2.2.3. Thor Lake (the Nechalacho deposit) | 7494 | ||
13.21.3.2.2.4. Strange Lake | 7494 | ||
13.21.3.3. Peraluminous Granite- and Pegmatite-Hosted Deposits | 7495 | ||
13.21.3.3.1. Peraluminous granite-hosted deposits | 7495 | ||
13.21.3.3.2. Peraluminous pegmatite-hosted deposits | 7495 | ||
13.21.3.4. Supergene Deposits | 7496 | ||
13.21.3.4.1. Saprolite deposits | 7496 | ||
13.21.3.4.2. Laterite deposits | 7496 | ||
13.21.3.4.3. Reworked laterite deposits | 7496 | ||
13.21.3.4.4. Ion-adsorbed clay deposits | 7496 | ||
13.21.3.5. Placer Deposits | 7497 | ||
13.21.4. Genesis of HFSE Deposits | 7497 | ||
13.21.4.1. Magmatic Controls of Carbonatite Deposits | 7497 | ||
13.21.4.2. Hydrothermal Controls of Carbonatite Deposits | 7498 | ||
13.21.4.3. Magmatic Controls of Alkaline Silicate Environments | 7498 | ||
13.21.4.4. Hydrothermal Controls of Alkaline Silicate Environments | 7499 | ||
13.21.4.5. Magmatic Controls of Peraluminous Environments | 7499 | ||
13.21.4.6. Hydrothermal Controls of Peraluminous Environments | 7500 | ||
13.21.5. Commonalities of Rare-Element Mineralization | 7500 | ||
Acknowledgments | 7500 | ||
References | 7500 | ||
Relevant Websites | 7504 | ||
Chapter 13.22: Geochemistry of Evaporite Ores in an Earth-Scale Climatic and Tectonic Framework | 7505 | ||
13.22.1. Introduction | 7505 | ||
13.22.2. Extractable Economic Salts (Excluding Halite and CaSO4 Salts) | 7505 | ||
13.22.3. Sodium Carbonate (Soda-Ash: Trona) | 7506 | ||
13.22.4. Sodium Sulfate (Salt-Cake) | 7507 | ||
13.22.5. Borate and Lithium Occurrences | 7509 | ||
13.22.6. Climatic and Tectonic Controls on Nonmarine Salts | 7516 | ||
13.22.7. Potash Salts | 7518 | ||
References | 7527 | ||
Chapter 13.23: Gem Deposits | 7531 | ||
13.23.1. Introduction | 7531 | ||
13.23.2. Diamond | 7532 | ||
13.23.2.1. Morphology | 7532 | ||
13.23.2.2. Crystal Chemistry and Diamond Types | 7532 | ||
13.23.2.3. Environment of Diamond Formation and Associated Inclusion Suites | 7533 | ||
13.23.2.3.1. Diamond-forming reactions and mantle metasomatism | 7533 | ||
13.23.2.3.2. Inclusion suites and diamond-forming environments | 7533 | ||
13.23.2.3.3. Pressure and temperature constraints | 7534 | ||
13.23.2.4. Stable Isotopes of Diamond and Diamond Inclusions | 7535 | ||
13.23.2.5. Dating | 7535 | ||
13.23.2.6. Model | 7536 | ||
13.23.3. Ruby and Sapphire | 7536 | ||
13.23.3.1. Introduction | 7536 | ||
13.23.3.2. Distribution and Classification of Gem Corundum Deposits | 7537 | ||
13.23.3.3. Recent Studies | 7537 | ||
13.23.3.3.1. Gem corundum in marble | 7538 | ||
13.23.3.3.2. Gem corundum in volcanic rocks | 7538 | ||
13.23.3.3.3. Sapphire in lamprophyric dikes | 7538 | ||
13.23.3.3.4. Metasomatic sapphire | 7538 | ||
13.23.3.3.5. Gem corundum in placers | 7538 | ||
13.23.3.4. Exploration | 7539 | ||
13.23.4. Emerald | 7539 | ||
13.23.4.1. Introduction | 7539 | ||
13.23.4.2. The Crystal Chemistry of Beryl | 7539 | ||
13.23.4.3. The Geochemistry of Be, Cr, and V | 7542 | ||
13.23.4.4. Deposit Models | 7542 | ||
13.23.4.4.1. Emerald deposits related to granitic intrusions | 7542 | ||
13.23.4.4.2. The Colombian model | 7543 | ||
13.23.4.4.3. Regional metamorphism and tectonometamorphic processes | 7543 | ||
13.23.4.5. Genetic Classification | 7545 | ||
13.23.4.6. Exploration | 7546 | ||
13.23.5. Non-Emerald Gem Beryl | 7547 | ||
13.23.6. Chrysoberyl | 7547 | ||
13.23.7. Tanzanite | 7549 | ||
13.23.8. Tsavorite | 7549 | ||
13.23.9. Topaz | 7550 | ||
13.23.10. Jade | 7550 | ||
13.23.10.1. Introduction | 7550 | ||
13.23.10.2. Jadeitite | 7551 | ||
13.23.10.3. Nephrite | 7551 | ||
Acknowledgments | 7552 | ||
References | 7552 | ||
Chapter 13.24: Exploration Geochemistry | 7559 | ||
13.24.1. Introduction | 7559 | ||
13.24.1.1. Role of Geochemistry in Mineral Exploration | 7559 | ||
13.24.1.2. Geochemical Processes and Terrains | 7559 | ||
13.24.2. The Primary Environment | 7560 | ||
13.24.3. The Secondary Environment | 7563 | ||
13.24.3.1. Weathering and Dispersion | 7563 | ||
13.24.3.2. Arid and Deeply Weathered Terrains | 7565 | ||
13.24.3.3. Transported Cover | 7566 | ||
13.24.3.4. Glaciated Terrains | 7569 | ||
13.24.3.5. Other Example Techniques | 7571 | ||
13.24.3.5.1. Heavy indicator minerals | 7571 | ||
13.24.3.5.2. Hydrogeochemistry | 7571 | ||
13.24.3.5.3. Biogeochemistry | 7574 | ||
13.24.3.5.4. Vapor and gas geochemistry | 7574 | ||
13.24.4. Regional Geochemical Mapping | 7575 | ||
13.24.5. Analysis | 7577 | ||
13.24.6. Geochemical Data Interpretation | 7580 | ||
Acknowledgments | 7582 | ||
References | 7582 | ||
e9780080983004v14 | 7587 | ||
Front Cover | 7587 | ||
Archaeology and Anthropology | 7590 | ||
Copyright | 7592 | ||
In Memoriam | 7594 | ||
Heinrich Dieter Holland (1927–2012) | 7596 | ||
Karl Karekin Turekian (1927–2013) | 7598 | ||
References | 7600 | ||
Dedication | 7602 | ||
Contents | 7604 | ||
Executive Editors’ Foreword to the Second Edition | 7606 | ||
Contributors | 7610 | ||
Volume Editor’s Introduction | 7612 | ||
References | 7613 | ||
Chapter 14.1: K/Ar and 40Ar/39Ar Isotopic Dating Techniques as Applied to Young Volcanic Rocks, Particularly Those Associated with Hominin Lo | 7614 | ||
14.1.1. Introduction | 7614 | ||
14.1.2. Basis of the K/Ar and 40Ar/39Ar Dating Techniques | 7614 | ||
14.1.3. Suitable Materials for Dating | 7618 | ||
14.1.4. Size Limitations | 7619 | ||
14.1.5. The Omo-Turkana Basin Sequence | 7620 | ||
14.1.6. Results from Afar, Ethiopia | 7625 | ||
14.1.7. Conclusions | 7626 | ||
Acknowledgments | 7627 | ||
References | 7627 | ||
Chapter 14.2: Luminescence Dating Methods | 7630 | ||
14.2.1. Luminescence Dating | 7630 | ||
14.2.1.1. Introduction | 7630 | ||
14.2.1.2. Production of Luminescence Signals | 7631 | ||
14.2.1.3. Preparation of Samples | 7633 | ||
14.2.1.4. Determining De | 7634 | ||
14.2.1.5. Combining De Datasets | 7635 | ||
14.2.1.6. Dose Rate Determination | 7637 | ||
14.2.1.7. Problems | 7638 | ||
14.2.1.8. Single-Grain Measurements | 7639 | ||
14.2.2. Applications | 7640 | ||
14.2.2.1. General Impact | 7640 | ||
14.2.2.2. The MSA in Southern Africa | 7640 | ||
14.2.2.3. The Aterian and Earlier Stone Industries in North Africa | 7643 | ||
14.2.2.4. Human Arrival in Australia | 7645 | ||
14.2.3. Summary | 7645 | ||
Acknowledgments | 7646 | ||
References | 7646 | ||
Chapter 14.3: Radiocarbon: Calibration to Absolute Time Scale | 7650 | ||
Glossary | 7650 | ||
14.3.1. Introduction | 7650 | ||
14.3.2. Variable Atmospheric 14C Content | 7650 | ||
14.3.3. Radiocarbon Calibration Curve | 7651 | ||
14.3.4. Calibration and Calibration Programs | 7653 | ||
14.3.5. Calibration in Archaeological Studies | 7653 | ||
References | 7655 | ||
Chapter 14.4: Radiocarbon: Archaeological Applications | 7658 | ||
14.4.1. Introduction | 7658 | ||
14.4.2. Late Paleolithic | 7658 | ||
14.4.3. Neolithic | 7659 | ||
14.4.4. Development of Metal Use | 7659 | ||
14.4.5. Bronze Age | 7659 | ||
14.4.6. Iron Age | 7660 | ||
14.4.7. Egyptian Chronologies | 7660 | ||
14.4.8. New World Archaeology | 7660 | ||
14.4.9. Australia | 7661 | ||
14.4.10. Polynesia | 7661 | ||
14.4.11. Chemistry | 7662 | ||
14.4.12. Bone Dating | 7662 | ||
14.4.13. Radiocarbon Dating of Art Works and Historical Objects | 7662 | ||
14.4.14. Understanding Radiocarbon Dates | 7663 | ||
14.4.14.1. The Significance of Radiocarbon Reservoir Effects | 7664 | ||
14.4.14.2. Regional and Site-Specific Differences in Atmospheric 14C | 7664 | ||
14.4.15. Bayesian Modeling | 7664 | ||
References | 7664 | ||
Chapter 14.5: The Molecular Clock | 7668 | ||
14.5.1. Introduction | 7668 | ||
14.5.2. Historical Overview | 7668 | ||
14.5.3. A Numerical Example: The Chimp-Human Common Ancestor | 7669 | ||
14.5.4. Difficulties with the Molecular Clock | 7669 | ||
14.5.4.1. Uncertainties | 7669 | ||
14.5.4.2. Purifying Selection | 7670 | ||
14.5.4.3. Variation in Rates | 7670 | ||
14.5.5. Coping with an Imperfect Clock | 7671 | ||
14.5.5.1. Excluding Variant Lineages | 7671 | ||
14.5.5.2. Relaxed Clocks | 7672 | ||
14.5.6. Using Multiple Genes | 7672 | ||
14.5.7. Conclusions | 7673 | ||
References | 7673 | ||
Chapter 14.6: Correlation: Volcanic Ash, Obsidian | 7676 | ||
14.6.1. Introduction | 7676 | ||
14.6.2. Some Relatively Common Types of Natural Glass and Their Compositions | 7677 | ||
14.6.3. Field Occurrence | 7679 | ||
14.6.4. Sample Preparation | 7680 | ||
14.6.5. Analytical Techniques | 7681 | ||
14.6.5.1. Electron Probe Microanalysis | 7681 | ||
14.6.5.2. Laser Ablation, Inductively Coupled Plasma Mass Spectrometry | 7681 | ||
14.6.5.3. X-Ray Fluorescence | 7682 | ||
14.6.6. Handling Analyses | 7682 | ||
14.6.6.1. Analyses of Individual Shards and | 7682 | ||
14.6.6.2. Analyses of Bulk Samples | 7683 | ||
14.6.7. Recalculation of Analyses | 7683 | ||
14.6.8. Sets of Analyses | 7684 | ||
14.6.8.1. Unimodal Ash Layers | 7684 | ||
14.6.8.2. Bimodal Ash Layers | 7685 | ||
14.6.8.3.Ash Layers with Linear Arrays of Analyses | 7685 | ||
14.6.9. The Problem of Alkali Content | 7687 | ||
14.6.10. Comparison of Analyses | 7689 | ||
14.6.11. Examples of Uses of Volcanic Glass in Archaeological Studies | 7689 | ||
14.6.11.1. Determining Sources of Obsidian | 7689 | ||
14.6.11.2. Correlation of Archaeological Sites | 7690 | ||
14.6.11.3. Primary Dates on Archaeological Material | 7691 | ||
14.6.11.4. Indirect Dates and Climatic Information through Correlations to Marine Cores | 7691 | ||
Acknowledgments | 7692 | ||
References | 7692 | ||
Chapter 14.7: Cosmogenic Nuclide Burial Dating in Archaeology and Paleoanthropology | 7694 | ||
14.7.1. Introduction | 7694 | ||
14.7.2. Cosmogenic Nuclides | 7695 | ||
14.7.2.1. Cosmogenic Nuclide Production | 7695 | ||
14.7.2.2. Production Rates at the Surface | 7695 | ||
14.7.2.2.1. Latitude | 7696 | ||
14.7.2.2.2. Altitude | 7696 | ||
14.7.2.2.3. Time | 7696 | ||
14.7.2.3. Production Rates at Depth | 7696 | ||
14.7.2.3.1. Neutrons | 7696 | ||
14.7.2.3.2. Muons | 7696 | ||
14.7.2.3.2.1. Negative muon capture | 7697 | ||
14.7.2.3.2.2. Fast muons | 7697 | ||
14.7.2.4. Cosmogenic Nuclide Buildup in Rocks | 7697 | ||
14.7.2.4.1. Exposure | 7698 | ||
14.7.2.4.2. Erosion | 7698 | ||
14.7.3. Burial Dating | 7698 | ||
14.7.3.1. Burial Dating Theory | 7699 | ||
14.7.3.2. Simple Burial Dating | 7699 | ||
14.7.3.2.1. Example: Sima del Elefante, Atapuerca | 7700 | ||
14.7.3.3. Min/Max Methods | 7701 | ||
14.7.3.3.1. Example: Rietputs Formation, lower Vaal River | 7702 | ||
14.7.3.4. Isochron Dating | 7702 | ||
14.7.3.4.1. Example: Sundays River | 7703 | ||
14.7.3.5. Uncertainties and limits of burial dating | 7704 | ||
14.7.4. Applications to Archaeology and Paleoanthropology | 7705 | ||
14.7.4.1. Simple Burial Dating: Zhoukoudian, China | 7705 | ||
14.7.4.2. Min/Max Burial Dating: Rietputs Formation, South Africa | 7706 | ||
14.7.4.3. Min/Max Burial Dating: Attirampakkam, India | 7707 | ||
14.7.4.4. Problematic Burial Dates | 7708 | ||
14.7.4.4.1. Sterkfontein, South Africa | 7708 | ||
14.7.4.4.2. Luangwa Valley, Zambia | 7708 | ||
14.7.5. Summary | 7709 | ||
References | 7709 | ||
Chapter 14.8: Marine Sediment Records of African Climate Change: Progress and Puzzles | 7712 | ||
14.8.1. Introduction | 7712 | ||
14.8.2. Marine Sediments as Recorders of Terrestrial Climate Change | 7713 | ||
14.8.2.1. Eolian Dust Supply, Composition, and Grain Size | 7713 | ||
14.8.2.2. River Runoff, Paleohydrology, and Vegetation Proxies | 7716 | ||
14.8.3. Marine Sediment Records of African Paleoclimate: Progress and Puzzles | 7718 | ||
14.8.3.1. Summary of North African Climate Evolution Since the Last Glacial Maximum | 7718 | ||
14.8.3.2. Fidelity of Aridity Proxies: Dustiness or Gustiness? | 7718 | ||
14.8.3.3. An Abrupt or Gradual End of the AHP near 5k | 7719 | ||
14.8.4. Summary and Future Directions | 7719 | ||
References | 7720 | ||
Chapter 14.9: History of Water in the Middle East and North Africa | 7722 | ||
14.9.1. Introduction | 7722 | ||
14.9.1.1. Geographical Characteristics and Distribution of the Middle East and North Africa | 7722 | ||
14.9.1.2. Present-Day Synoptic System in the Middle East | 7723 | ||
14.9.1.3. Present-Day Synoptic System in North Africa | 7724 | ||
14.9.2. Paleoclimate of the Middle East and Northeast Africa | 7725 | ||
14.9.2.1. Paleoclimate of the Middle East and Northeast Africa Based on Deep Sea Sediments from the East | 7725 | ||
14.9.2.2. Paleoclimate of the Middle East and North Africa Based on Speleothems and Lake Records | 7727 | ||
14.9.2.2.1. Speleothems | 7727 | ||
14.9.2.3. Eastern Mediterranean Speleothem Records | 7728 | ||
14.9.2.4. Desert Speleothems | 7729 | ||
14.9.2.4.1. Origin of rainfall for the desert speleothems | 7731 | ||
14.9.2.4.2. The MIS 6/5 transition | 7732 | ||
14.9.2.5. Arabian Speleothems | 7732 | ||
14.9.2.5.1. Lakes | 7733 | ||
14.9.2.6. Eastern Mediterranean and North African Lakes | 7734 | ||
14.9.2.7. The Connection between `Wet´ Episodes in Northeast Africa-Middle East and H | 7735 | ||
14.9.3. Conclusions | 7736 | ||
References | 7737 | ||
Chapter 14.10: The Carbon, Oxygen, and Clumped Isotopic Composition of Soil Carbonate in Archeology | 7742 | ||
14.10.1. Introduction | 7742 | ||
14.10.2. Paleosol Carbonate Recognition | 7742 | ||
14.10.3. Limitations for Archeologists | 7744 | ||
14.10.4. Seasonality of Formation and Isotopic Equilibrium | 7744 | ||
14.10.5. Carbon Isotopes in Soil Carbonate | 7745 | ||
14.10.5.1. Plants and Climate | 7745 | ||
14.10.5.2. The Soil Diffusion Model | 7745 | ||
14.10.5.3. Reconstructing the Fraction Woody Cover | 7747 | ||
14.10.5.4. The Radiation of C4 Plants and Evolution of Hominids | 7748 | ||
14.10.5.4.1. Phase I (>8.4Ma): A mainly C3 world | 7750 | ||
14.10.5.4.2. Phase II (8.4-7Ma): C4 grasses appear | 7750 | ||
14.10.5.4.3. Phase III (7-2.7Ma): Expansion of C4 grasses | 7750 | ||
14.10.5.4.4. Phase IV (2.7Ma to present): Development of modern savannas in most of East Africa | 7750 | ||
14.10.6. Clumped Isotopes in Soil Carbonate | 7750 | ||
14.10.6.1. Principles and Methods | 7750 | ||
14.10.6.2. Soil Temperature Considerations | 7751 | ||
14.10.6.3. Examples of Use | 7751 | ||
14.10.7. Oxygen in Soil Carbonate | 7752 | ||
14.10.7.1. Temperature Effects | 7752 | ||
14.10.7.2. Composition of Precipitation | 7752 | ||
14.10.7.3. Soil Water Evaporation | 7752 | ||
14.10.7.4. The Late Cenozoic Oxygen Isotope Shift | 7753 | ||
14.10.8. Integrity of the Isotopic Record from Soil Carbonate | 7753 | ||
14.10.9. Environmental Reconstruction on Short Timescales and Future Directions | 7754 | ||
Acknowledgments | 7754 | ||
References | 7754 | ||
Chapter 14.11: Microanalytical Isotope Chemistry: Applications for Archaeology | 7758 | ||
14.11.1. History of Micromilling Technology | 7758 | ||
14.11.2. Applications of Micromilling Devices toward the Enhancement of Sampling Strategies and Derivation of High-Resolution Records | 7760 | ||
14.11.2.1. Mollusks | 7760 | ||
14.11.2.1.1. From seasonality of site occupation to seasonality of temperature | 7760 | ||
14.11.2.2. Fish Otoliths | 7760 | ||
14.11.2.3. Derivation of Seasonality of Temperature in Continental &INS id= | 7761 | ||
14.11.2.4. Seasonality of Site Occupation | 7763 | ||
14.11.2.5. Coral | 7763 | ||
14.11.2.6. Speleothems | 7763 | ||
14.11.2.7. Teeth | 7764 | ||
14.11.2.8. Trees | 7765 | ||
14.11.3. Future Advances and Directions | 7766 | ||
14.11.4. Conclusions | 7766 | ||
14.11.5. Partial List of Applications of Micromilling in Archaeology | 7766 | ||
14.11.5.1. Mollusks | 7766 | ||
14.11.5.2. Otoliths | 7766 | ||
14.11.5.3. Bone | 7766 | ||
14.11.5.4. Speleothems | 7766 | ||
14.11.5.5. Teeth | 7766 | ||
14.11.5.6. Hair | 7766 | ||
Acknowledgments | 7766 | ||
References | 7767 | ||
Chapter 14.12: Stable Isotope Evidence for Hominin Environments in Africa | 7770 | ||
14.12.1. Introduction | 7771 | ||
14.12.2. Carbon Isotopes in Plants | 7771 | ||
14.12.3. Ecology of Mixed C3 and C4 Ecosystems | 7772 | ||
12.13.3.1. Isotopes and Shade: Quantification of Woody Cover | 7772 | ||
14.12.3.2. Paleosols as Recorders of Paleoenvironments in African Hominin Sites | 7774 | ||
14.12.4. Paleotemperature | 7775 | ||
14.12.4.1. Temperature as a Variable in Human Evolution | 7775 | ||
14.12.4.2. Paleotemperature from Delta47 in Paleosols | 7775 | ||
14.12.5. Diet History of Mammals | 7776 | ||
14.12.5.1. Isotopes Distinguish Between Grazing and Browsing | 7776 | ||
14.12.5.2. Diet Histories of Nonprimates Associated with Hominins | 7776 | ||
14.12.5.2.1. Overall faunal preferences | 7776 | ||
14.12.5.3. Diets of Early Human Relatives | 7777 | ||
14.12.6. Summary and Future Directions | 7777 | ||
Acknowledgments | 7779 | ||
References | 7779 | ||
Chapter 14.13: Geochemistry of Ancient Metallurgy: Examples from Africa and Elsewhere | 7782 | ||
14.13.1. Introduction | 7782 | ||
14.13.2. Chemistry of Ancient Metallurgy | 7784 | ||
14.13.3. Geochemistry Methods in Archaeometallurgy: Some Common Examples | 7787 | ||
14.13.4. Geochemistry Applications in Ancient Metallurgy | 7789 | ||
14.13.4.1. One of the Earliest Beginnings of Metallurgy in the World: The Case of the Balkans | 7789 | ||
14.13.4.2. The Unresolved Case of the Earliest African Metallurgy: Contributions of Geochemistry | 7790 | ||
14.13.4.3. Getting to Grips with the Full Ancient Metal Production Chain: Technology, Organization of Production, and Social | 7791 | ||
14.13.4.4. Going Beyond System Generated Constraints in Ancient Metal Smelting | 7793 | ||
14.13.4.5. Provenancing Artifacts Using NAA and Lead Isotope Analysis | 7793 | ||
14.13.4.6. Reading Gender Specialization in Ancient Metallurgy: Contributions of Geochemistry | 7795 | ||
14.13.4.7. Geochemistry for Conservation: Oranjemund Shipwreck | 7797 | ||
14.13.5. Conclusion | 7800 | ||
Acknowledgments | 7800 | ||
References | 7800 | ||
Chapter 14.14: Elemental and Isotopic Analysis of Ancient Ceramics and Glass | 7804 | ||
14.14.1. Introduction | 7804 | ||
14.14.2. Considerations on Archeological Ceramic Studies | 7805 | ||
14.14.2.1. Pottery in Archeology | 7805 | ||
14.14.2.2. Pottery and Provenance | 7805 | ||
14.14.2.3. Pottery Analyses | 7806 | ||
14.14.2.3.1. Ceramic petrography and mineralogy | 7806 | ||
14.14.2.3.2. Chemical analysis of ceramics | 7806 | ||
14.14.2.3.2.1. Analytical techniques and methodology | 7806 | ||
14.14.2.3.2.2. A summary of useful elements in ceramic provenance studies | 7806 | ||
14.14.2.3.2.2.1. Cr, Ni, and Co | 7807 | ||
14.14.2.3.2.2.2. Zr (Hf) | 7807 | ||
14.14.2.3.2.2.3. Y | 7807 | ||
14.14.2.3.2.2.4. Ti | 7807 | ||
14.14.2.3.2.2.5. Sr | 7807 | ||
14.14.2.3.2.2.6. K, Rb | 7807 | ||
14.14.2.3.2.2.7. Ba | 7807 | ||
14.14.2.3.2.2.8. Fe | 7807 | ||
14.14.2.3.2.2.9. Th (U) | 7807 | ||
14.14.2.3.3. Data treatment in ceramic provenancing | 7808 | ||
14.14.2.4. Case Studies in Pottery Analysis | 7808 | ||
14.14.3. Considerations on Archeological Glass Analysis | 7810 | ||
14.14.3.1. Glass in Archeology | 7810 | ||
14.14.3.2. Glass and Provenance | 7811 | ||
14.14.3.3. Glass Analyses | 7813 | ||
14.14.3.3.1. Glass provenance and elemental analysis | 7813 | ||
14.14.3.3.1.1. Glass flux types: sodium, potassium, magnesium, phosphorous | 7813 | ||
14.14.3.3.1.2. Glass network former and stabilizer: silica, lime, alumina | 7813 | ||
14.14.3.3.1.3. Glass colorants: antimony, manganese, copper, cobalt, tin, etc. | 7813 | ||
14.14.3.3.1.4. Glass provenance: other minor and trace elements | 7813 | ||
14.14.3.3.2. Glass provenance and isotopes | 7814 | ||
14.14.3.3.2.1. Oxygen isotopes | 7814 | ||
14.14.3.3.2.2. Lead isotopes | 7814 | ||
14.14.3.3.2.3. Strontium isotopes | 7815 | ||
14.14.3.3.2.4. Neodymium isotopes | 7815 | ||
14.14.3.4. Case Study in Glass Analysis | 7815 | ||
References | 7816 | ||
Chapter 14.15: Synchrotron Methods: Color in Paints and Minerals | 7822 | ||
14.15.1. Introduction | 7823 | ||
14.15.1.1. Role of Color in Archaeology | 7823 | ||
14.15.1.2. What Kind of Information Is Looked for When Searching for Investigating Color in Paints or Minerals? | 7824 | ||
14.15.1.3. From the Color Cause of Ancient Materials to Archaeological Information | 7824 | ||
14.15.1.4. Physical and Chemical Causes of Color Important in Terms of Archaeological Materials | 7824 | ||
14.15.2. Studies of Ancient Pigments, Paints, and Minerals | 7824 | ||
14.15.2.1. Definition of Pigments, Colorants, and Paints | 7824 | ||
14.15.2.2. Relevant Archaeological and Historical Pigments | 7824 | ||
14.15.2.3. Relevant Archaeological and Historical Minerals, Biominerals, Colored Stones, and Gemstones | 7825 | ||
14.15.3. History of Their Study and Current Trends | 7828 | ||
14.15.3.1. First Archaeometric Laboratory Studies | 7828 | ||
14.15.3.2. General Approach for Studying Archaeomaterials | 7830 | ||
14.15.3.3. Neutron Sources | 7831 | ||
14.15.3.4. Ion Beam Analyses | 7831 | ||
14.15.3.5. Current Trends | 7831 | ||
14.15.4. Overview of Synchrotron-Based Method Used for the Study of Pigments, Paints, and Minerals | 7831 | ||
14.15.4.1. Why Use Synchrotron-Based X-Ray Methods to Study Color of Archaeomaterials? | 7831 | ||
14.15.4.2. Chemical Analysis | 7832 | ||
14.15.4.3. Structural Investigations | 7833 | ||
14.15.4.4. Molecular Studies | 7834 | ||
14.15.4.5. Spectroscopic Studies | 7834 | ||
14.15.4.6. Noninvasive Depth-Resolved Investigations | 7836 | ||
14.15.4.7. Synchrotrons with Important Activities in the Field of CH Studies | 7837 | ||
14.15.5. Case Studies | 7837 | ||
14.15.5.1. Identification of Pigments and Minerals | 7837 | ||
14.15.5.1.1. Black Paleolithic paints in the Lascaux cave (Dordogne): use of different Mn oxides with diffe | 7837 | ||
14.15.5.1.2. Color shades investigated in Roman frescoes by SR-micro-XRD mapping | 7839 | ||
14.15.5.1.3. Possible differentiation of different lead whites in successive paint layers of the Isenheim A | 7839 | ||
14.15.5.1.4. Special Zn-containing pigments found in Indian miniature paintings long before European paintings: | 7839 | ||
14.15.5.1.5. Color reconstruction of a hidden face painted by Vincent Van Gogh using fast macro-XRF mapping combined with mic | 7841 | ||
14.15.5.2. Early Synthesis of Colored Matter in Ancient Civilizations | 7841 | ||
14.15.5.2.1. Early chemical synthesis to manufacture ancient Egyptian make& | 7841 | ||
14.15.5.2.2. Different production processes for two different synthetic pigments used in ancient Egypt | 7843 | ||
14.15.5.2.3. Synthetic Chinese purple pigment (Han purple) used on Qin terracotta warriors: technology transfer from Egypt to | 7844 | ||
14.15.5.2.4. A hybrid synthetic pigment with amazing stability over time: Maya blue in pre-Columbian Mesoamerica | 7844 | ||
14.15.5.3. Study of Paint and Pigment Discoloration | 7845 | ||
14.15.5.3.1. Hiding or discoloration of prehistoric paints in limestone caves (Large cave of Arcy-sur-Cure, | 7845 | ||
14.15.5.3.2. From fossil bone to turquoise imitation | 7846 | ||
14.15.5.3.3. Blackening of Pompeian wall paintings | 7847 | ||
14.15.5.3.4. Discoloration of smalt used as pigment in paintings | 7848 | ||
14.15.6. Conclusion and Trends | 7848 | ||
References | 7849 | ||
Chapter 14.16: Geochemical Methods of Establishing Provenance and Authenticity of Mediterranean Marbles | 7854 | ||
14.16.1. Foreword | 7854 | ||
14.16.2. Types of Fakes | 7855 | ||
14.16.3. Determining Marble Provenance | 7856 | ||
14.16.3.1. Analytical Techniques | 7856 | ||
14.16.3.1.1. Stable isotope methodology | 7857 | ||
14.16.3.1.2. Electron paramagnetic resonance/electron spin resonance | 7857 | ||
14.16.3.1.3. A note on the use of CL in marbles | 7859 | ||
14.16.3.2. Case Studies | 7859 | ||
14.16.3.2.1. Excavations at Cyrene | 7859 | ||
14.16.3.2.2. Cyrene revisited | 7859 | ||
14.16.3.2.3. Deconstructing Antonia | 7860 | ||
14.16.3.2.4. Aphrodite | 7862 | ||
14.16.3.2.5. Aphrodite in the National Gallery of Art | 7863 | ||
14.16.4. Testing Authenticity | 7864 | ||
14.16.5. Summary | 7864 | ||
References | 7865 | ||
Chapter 14.17: Biblical Events and Environments - Authentification of Controversial Archaeological Artifacts | 7868 | ||
14.17.1. Introduction | 7868 | ||
14.17.1.1. Archaeological Forgery | 7868 | ||
14.17.1.2. Patina | 7869 | ||
14.17.1.3. Oxygen and Carbon Isotopic Composition | 7869 | ||
14.17.2. The James Ossuary | 7870 | ||
14.17.2.1. The Ossuary | 7870 | ||
14.17.2.2. Authenticity Studies | 7871 | ||
14.17.3. Jehoash Inscription | 7874 | ||
14.17.3.1. The Tablet | 7874 | ||
14.17.3.2. Authenticity Studies | 7874 | ||
14.17.3.2.1. Petrography | 7875 | ||
14.17.3.2.2. Oxygen isotopic composition | 7875 | ||
14.17.4. The Ivory Pomegranate | 7877 | ||
14.17.4.1. The Pomegranate | 7877 | ||
14.17.4.2. Authenticity Study | 7877 | ||
14.17.4.2.1. The inscription | 7877 | ||
14.17.4.2.2. The patina | 7878 | ||
14.17.4.2.2.1. Stable isotope analysis | 7878 | ||
14.17.5. Iron Age Ostraca | 7878 | ||
14.17.5.1. The ostraca | 7878 | ||
14.17.5.2. Authenticity Examination | 7879 | ||
14.17.5.2.1. Micromorphologic and petrographic examination | 7879 | ||
14.17.5.2.2. Oxygen and carbon isotopic composition | 7879 | ||
14.17.6. Dust | 7881 | ||
14.17.7. Conclusions | 7881 | ||
References | 7882 | ||
Chapter 14.18: Trace Evidence: Glass, Paint, Soil, and Bone | 7884 | ||
14.18.1. Introduction | 7885 | ||
14.18.2. Elemental Analysis Techniques | 7886 | ||
14.18.3. Man-Made Matrices | 7887 | ||
14.18.3.1. Glass | 7887 | ||
14.18.3.2. Elemental Analysis of Glass | 7887 | ||
14.18.3.3. Paint | 7887 | ||
14.18.3.4. Elemental Analysis of Paint | 7887 | ||
14.18.4. Natural Matrices | 7888 | ||
14.18.4.1. Soil | 7888 | ||
14.18.4.2. Properties of Soil | 7888 | ||
14.18.4.3. Soil Sampling and Sample Preparation | 7888 | ||
14.18.4.4. Analysis of Soil | 7889 | ||
14.18.4.5. Elemental Analysis of Soil | 7889 | ||
14.18.4.6. Bones and Teeth | 7890 | ||
14.18.4.7. Properties of Bone | 7891 | ||
14.18.4.8. Bone Sampling and Sample Preparation | 7891 | ||
14.18.4.9. Analysis of Bone | 7891 | ||
14.18.4.10. Elemental Analysis of Bone | 7892 | ||
14.18.5. Interpretation | 7893 | ||
14.18.6. Conclusion | 7894 | ||
References | 7894 | ||
Chapter 14.19: Stable Isotopes in Forensics Applications | 7898 | ||
14.19.1. Stable Isotope Geochemistry as a Science-Based Forensic Application | 7899 | ||
14.19.1.1. Overview | 7899 | ||
14.19.1.2. A Framework for Applying Stable Isotope Analysis in Forensic Investigations | 7899 | ||
14.19.2. Nonspatial Applications of Stable Isotope Analysis | 7900 | ||
14.19.2.1. Synthetic Drugs | 7900 | ||
14.19.2.1.1. Amphetamine-type stimulants | 7901 | ||
14.19.2.1.2. Performance-enhancing steroids | 7901 | ||
14.19.2.2. Manufactured Explosives | 7902 | ||
14.19.2.2.1. Peroxide-based explosives | 7902 | ||
14.19.2.2.2. An industrial explosive: ammonium nitrate | 7903 | ||
14.19.2.2.3. A militarygrade explosive: pentaerythritol tetranitrate | 7904 | ||
14.19.2.3. Packaging Materials | 7905 | ||
14.19.2.4. Crude Oils and Petroleum Products | 7905 | ||
14.19.3. Spatial Applications of Stable Isotope Analysis | 7905 | ||
14.19.3.1. Water Isotopes and the Isoscapes Foundational Approach | 7906 | ||
14.19.3.2. Waters, Beverages, and the Isoscapes Potential | 7907 | ||
14.19.4. Plant-Related Forensic Applications of Stable Isotope Analysis | 7908 | ||
14.19.4.1. Economic Adulteration of Foods | 7908 | ||
14.19.4.2. Food Origin Authentication | 7910 | ||
14.19.4.3. Controlled Substances Produced from Plants | 7911 | ||
14.19.4.3.1. Cocaine and heroin | 7911 | ||
14.19.4.3.2. Marijuana | 7912 | ||
14.19.4.4. Wood and other Plant Product Isotope Records | 7913 | ||
14.19.4.4.1. Arson - isotopic comparisons | 7913 | ||
14.19.4.4.2. Flavor compounds - adulteration detection | 7913 | ||
14.19.4.4.3. Plant and wood products - geographical region of origin | 7913 | ||
14.19.5. Human-Related Forensic Applications of Stable Isotope Analysis | 7914 | ||
14.19.5.1. Dietary Patterns | 7914 | ||
14.19.5.2. Spatial Patterns | 7915 | ||
14.19.5.2.1. Body water, geography, and metabolism | 7915 | ||
14.19.5.2.2. Carbonates and phosphates in teeth and bones | 7915 | ||
14.19.5.2.3. Proteins in bone and hair | 7916 | ||
14.19.5.3. Provenancing Unidentified Homicide Victims | 7917 | ||
14.19.5.4. Human Diseases | 7918 | ||
14.19.6. Animal-Related (Nonhuman) Forensic Applications of Stable Isotope Analysis | 7918 | ||
14.19.6.1. Animal Migration and Movement | 7918 | ||
14.19.6.2. Provenance of Trade Goods | 7920 | ||
14.19.7. Archaeological and Gem Origin Investigations Utilizing Stable Isotope Analysis | 7921 | ||
14.19.7.1. Sculpture Source: Monuments, Statues, and Artifacts | 7921 | ||
14.19.7.2. Gem Origins | 7922 | ||
14.19.7.2.1. Rubies and sapphires | 7922 | ||
14.19.7.2.2. Emeralds | 7922 | ||
14.19.7.2.3. Diamonds | 7922 | ||
14.19.8. Isotope Geochemists as Contributors to the Forensic Sciences | 7922 | ||
14.19.8.1. Addressing the Needs of the NRC Report | 7922 | ||
14.19.8.2. Isotopes in the Courts | 7923 | ||
14.19.8.3. Concluding Points | 7923 | ||
References | 7923 | ||
Chapter 14.20: Reconstructing Aquatic Resource Exploitation in Human Prehistory Using Lipid Biomarkers and Stable Isotopes | 7932 | ||
14.20.1. Introduction | 7932 | ||
14.20.2. Reconstructing Diet and Economy fr | 7933 | ||
14.20.3. The Lipid Composition of Aquatic Fats and Oils | 7934 | ||
14.20.4. Early Attempts to Detect Aquatic Lipids in the Archeological Record | 7934 | ||
14.20.5. New Aquatic Resource Biomarkers | 7935 | ||
14.20.5.1. Vicinal Dihydroxy Acids | 7935 | ||
14.20.5.2. Isoprenoid Fatty Acids | 7936 | ||
14.20.5.3. omega-(o-Alkylphenyl)alkanoic Acids | 7936 | ||
14.20.6. Stable Isotope Proxies | 7938 | ||
14.20.6.1. Carbon Isotopes | 7938 | ||
14.20.6.2. Hydrogen Isotopes | 7939 | ||
14.20.7. Experimental Approaches and Protocols | 7939 | ||
14.20.7.1. Thermal Degradation Experiments to Demonstrate Formation of Novel Lipid Biomarkers During the Heating of Unsaturat | 7939 | ||
14.20.7.2. High Sensitivity GC/MS Protocols for the Detection of Marine Lipid Biomarkers | 7940 | ||
14.20.7.3. Determination of Compound-Specific delta13C and deltaD Values of Individual n-Alkanoic Acids of Marine Organism | 7944 | ||
14.20.8. Detecting Evidence for Marine Product Processing in Prehistory Using Biomarker and Stable Isotope Proxies | 7945 | ||
14.20.8.1. Southern Brazil | 7945 | ||
14.20.8.2. South Africa | 7946 | ||
14.20.8.3. Northern and Northwestern Europe | 7947 | ||
14.20.8.4. The Arctic | 7948 | ||
14.20.9. Conclusions | 7948 | ||
Acknowledgments | 7950 | ||
References | 7950 | ||
Chapter 14.21: Investigating Ancient Diets Using Stable Isotopes in Bioapatites | 7954 | ||
14.21.1. Introduction | 7954 | ||
14.21.2. A Few Basics | 7954 | ||
14.21.2.1. Isotopes | 7954 | ||
14.21.2.2. Bioapatite | 7955 | ||
14.21.3. Development of the Field | 7955 | ||
14.21.3.1. Photosynthesis | 7955 | ||
14.21.3.2. From Plants to Animals | 7956 | ||
14.21.3.3. Enter Anthropology | 7957 | ||
14.21.3.4. Apatite as a Recorder on Geological Timescales | 7957 | ||
14.21.4. Practical Issues | 7958 | ||
14.21.4.1. Sampling and Analysis via Acid Hydrolysis | 7958 | ||
14.21.4.2. Diet-Tissue Relationships | 7958 | ||
14.21.4.3. Signal Attenuation | 7959 | ||
14.21.4.4. Coupling Apatite and Collagen - A | 7960 | ||
14.21.5. Applications | 7961 | ||
14.21.5.1. Anthropology | 7961 | ||
14.21.5.1.1. Holocene archaeology | 7961 | ||
14.21.5.1.2. Anthropology in the deeper past | 7963 | ||
14.21.5.2. Paleontology and Paleoecology | 7963 | ||
14.21.5.2.1. Carbon isotopes for paleodiet and p& | 7963 | ||
14.21.5.2.2. Oxygen isotopes in mammalian e | 7964 | ||
14.21.6. Conclusions | 7964 | ||
References | 7965 | ||
Chapter 14.22: Human Physiology in Relation to Isotopic Studies of Ancient and Modern Humans | 7970 | ||
14.22.1. Introduction | 7970 | ||
14.22.2. Molecular Constituents of Human T | 7970 | ||
14.22.2.1. Amino Acids | 7970 | ||
14.22.2.2. Proteins | 7972 | ||
14.22.2.3. Lipids | 7972 | ||
14.22.2.4. Methane | 7972 | ||
14.22.2.5. Inorganic Species in Solution | 7973 | ||
14.22.2.5.1. Processing of phosphate | 7973 | ||
14.22.3. Tissues Preserved Postmortem | 7974 | ||
14.22.3.1. Bone | 7974 | ||
14.22.3.1.1. Composition and structure | 7974 | ||
14.22.3.1.1.1. The mineral components of teeth and bone | 7974 | ||
14.22.3.1.1.2. Collagen | 7975 | ||
14.22.3.1.1.3. Noncollagenous proteins and other molecules | 7976 | ||
14.22.3.1.2. Structure of bone | 7976 | ||
14.22.3.1.3. Modeling and remodeling | 7977 | ||
14.22.3.2. Teeth: Composition, Structure, and Ontogeny | 7978 | ||
14.22.3.3. Hair | 7979 | ||
14.22.3.4. Lipids | 7979 | ||
14.22.4. Homeostasis, Mineral Stability | 7979 | ||
14.22.4.1. Calcium Phosphate Saturation | 7979 | ||
14.22.4.2. Osmoregulation, Water Stress | 7980 | ||
14.22.5. Nutritional and Metabolic Diseases | 7980 | ||
References | 7980 | ||
Chapter 14.23: Hair as a Geochemical Recorder: Ancient to Modern | 7984 | ||
14.23.1. Introduction | 7984 | ||
14.23.1.1. Structure and Composition of Hair | 7984 | ||
14.23.1.2. Hair Growth | 7985 | ||
14.23.2. Survival of Hair in Archaeological and Forensic Contexts | 7986 | ||
14.23.3. Studies of Isotope Ratios in Animal Hair | 7988 | ||
14.23.3.1. Animal Hair from Controlled, Experimental Situations | 7988 | ||
14.23.3.2. Animal Hair as an Environmental Sampling Tool | 7989 | ||
14.23.4. Anthropological Studies on Modern and Historically Collected Hair | 7992 | ||
14.23.5. Health and Medical Applications of Hair Analysis | 7994 | ||
14.23.5.1. Human Dietary Preferences and Stable Isotope Ratios | 7994 | ||
14.23.5.2. Use of Isotope Ratios to Investigate Nitrogen Balance during Pregnancy | 7995 | ||
14.23.5.3. Investigation of Stable Isotope Ratios as a Means of Diagnosing Eating Disorders | 7995 | ||
14.23.5.4. Trace Element Analysis of Human Hair as a Tool for Health Investigation | 7996 | ||
14.23.6. Archaeological Hair | 7996 | ||
14.23.6.1. The Americas | 7997 | ||
14.23.6.2. The Nile Valley | 7998 | ||
14.23.6.3. Other Regions | 7998 | ||
14.23.7. Applications to Forensic Investigations | 7998 | ||
14.23.7.1. Unidentified Human Remains | 7998 | ||
14.23.7.2. Wildlife Forensics and Isotopic Analysis of Hair | 7999 | ||
14.23.8. Geography and Temporal Dynamics in Hair Oxygen Isotope Ratios | 7999 | ||
14.23.9. Future Directions | 8001 | ||
References | 8001 | ||
e9780080983004v15 | 8007 | ||
Front Cover | 8007 | ||
Analytical Geochemistry/Inorganic Instrument Analysis | 8010 | ||
Copyright | 8011 | ||
In Memoriam | 8012 | ||
Heinrich Dieter Holland (1927–2012) | 8014 | ||
Karl Karekin Turekian (1927–2013) | 8016 | ||
References | 8018 | ||
Dedication | 8020 | ||
Contents | 8022 | ||
Executive Editors’ Foreword To The Second Edition | 8024 | ||
Contributors | 8028 | ||
Volume Editor’s Introduction | 8030 | ||
The First Step: Sampling Strategies and Getting Ready for the Lab | 8030 | ||
Uncertainties, Reference Materials, and Isotope Dilution | 8030 | ||
Digesting and Preparing Samples in the Clean Lab | 8031 | ||
Analyzing the Sample: Photons and Atomic Masses | 8031 | ||
Measuring Photons | 8032 | ||
Measuring Mass | 8033 | ||
Analyzing the Planet: New Developments | 8034 | ||
Chapter 15.1: Basic Considerations: Sampling, the Key for a Successful Applied Geochemical Survey for Mineral Exploration and Environ | 8036 | ||
15.1.1. Introduction and Background Information | 8036 | ||
15.1.2. Design of a Geochemical Sampling Campaign | 8037 | ||
15.1.2.1. Desk Study | 8037 | ||
15.1.2.2. Orientation Survey | 8037 | ||
15.1.2.2.1. Orientation survey in mineral exploration | 8037 | ||
15.1.2.2.2. Orientation survey in a contaminated land investigation | 8038 | ||
15.1.2.3. Regional Geochemical Survey | 8039 | ||
15.1.2.4. Follow-up Geochemical Survey | 8040 | ||
15.1.2.5. Detailed Geochemical Survey | 8040 | ||
15.1.2.5.1. Detailed rock or soil geochemical survey | 8041 | ||
15.1.2.5.2. Detailed contaminated land investigation | 8041 | ||
15.1.2.5.2.1. Systematic versus random sampling | 8043 | ||
15.1.2.5.2.2. Square block sampling | 8043 | ||
15.1.2.5.2.3. Composite versus spot sampling | 8043 | ||
15.1.3. Randomization of Samples | 8044 | ||
15.1.4. Quality Control - Duplicate Field Samples and Control Samples | 8044 | ||
15.1.5. Sampling | 8045 | ||
15.1.5.1. Stream Sediment Sampling | 8045 | ||
15.1.5.1.1. Wet sieving | 8047 | ||
15.1.5.1.2. Dry sieving | 8047 | ||
15.1.5.1.3. Without sieving | 8047 | ||
15.1.5.2. Overbank or Floodplain Sediment Sampling | 8047 | ||
15.1.5.3. Stream and Ground-Water Sampling | 8050 | ||
15.1.5.4. Rock Sampling | 8052 | ||
15.1.5.5. Soil Sampling | 8053 | ||
15.1.5.5.1. Soil sampling for mineral exploration | 8053 | ||
15.1.5.5.2. Soil sampling for environmental investigations | 8054 | ||
15.1.5.5.2.1. Urban soil sampling | 8055 | ||
15.1.5.5.2.2. Soil sampling for contaminated land investigations | 8057 | ||
15.1.5.6. House-Dust Sampling | 8057 | ||
15.1.5.7. Attic-Dust Sampling | 8058 | ||
15.1.5.8. Road Dust Sampling | 8059 | ||
15.1.6. Sampling in the Laboratory | 8059 | ||
15.1.7. Conclusions | 8062 | ||
Acknowledgments | 8062 | ||
References | 8063 | ||
Chapter 15.2: Error Propagation | 8068 | ||
15.2.1. Introduction | 8068 | ||
15.2.2. Accuracy, Precision, and Types of Errors | 8068 | ||
15.2.2.1. Accuracy and Precision | 8068 | ||
15.2.2.2. Errors | 8068 | ||
15.2.3. Statistical Treatment of Random Errors | 8069 | ||
15.2.3.1. Mean and Standard Deviation | 8069 | ||
15.2.3.2. Weighted Averages | 8070 | ||
15.2.3.3. Variance, Covariance, and Correlated Errors | 8070 | ||
15.2.4. Probability Distributions | 8071 | ||
15.2.4.1. Normal/Gaussian Distribution | 8071 | ||
15.2.4.1.1. Log-normal distribution | 8072 | ||
15.2.4.2. Poisson Distribution | 8072 | ||
15.2.5. Calibration Curves, Blank Standar | 8073 | ||
15.2.5.1. Calibration Curves | 8073 | ||
15.2.5.2. Blank Standard Deviation, Blank Standard | 8073 | ||
15.2.6. Error Propagation | 8075 | ||
15.2.6.1. Random Error Propagations | 8075 | ||
15.2.6.2. Systematic Error Propagations | 8076 | ||
15.2.5.3. Use of Least Squares to Estimate | 8074 | ||
Acknowledgments | 8077 | ||
References | 8077 | ||
Chapter 15.3: Reference Materials in Geochemical and Environmental Research | 8078 | ||
15.3.1. Introduction | 8079 | ||
15.3.2. ISO Guidelines and IAG Certification Protocol | 8079 | ||
15.3.3. Rock Reference Materials | 8081 | ||
15.3.3.1. Preparation | 8081 | ||
15.3.3.2. Frequently Used Rock Reference Materials | 8082 | ||
15.3.3.2.1. USGS basalts and andesites | 8083 | ||
15.3.3.2.2. Certified reference materials | 8083 | ||
15.3.3.2.3. Reference materials from diverse providers | 8084 | ||
15.3.3.2.4. Reference materials for platinum-group elements | 8085 | ||
15.3.4. Environmental Reference Materials | 8085 | ||
15.3.4.1. Soils and Sediments | 8085 | ||
15.3.4.2. Dust and Air Particulates and Nanoparticles | 8086 | ||
15.3.4.3. Water Reference Materials | 8086 | ||
15.3.4.4. Biota | 8086 | ||
15.3.5. Microanalytical Reference Materials | 8086 | ||
15.3.5.1. Synthetic Reference Glasses | 8087 | ||
15.3.5.1.1. NIST SRM reference glasses | 8087 | ||
15.3.5.1.2. BAM-certified reference glasses | 8087 | ||
15.3.5.1.3. USGS GS reference glasses | 8087 | ||
15.3.5.2. Natural Reference Glasses | 8091 | ||
15.3.5.2.1. Geologic USGS reference glasses | 8091 | ||
15.3.5.2.2. Geologic MPI-DING reference glasses | 8092 | ||
15.3.5.2.3. Geologic NRCG reference glasses | 8092 | ||
15.3.5.3. Mineral Reference Materials | 8092 | ||
15.3.5.3.1. Zircon | 8092 | ||
15.3.5.3.2. Sulfide reference materials | 8093 | ||
15.3.5.3.3. Other mineral reference materials | 8093 | ||
15.3.6. Isotopic Reference Materials | 8093 | ||
15.3.6.1. Radiogenic Isotopic Reference Materials | 8093 | ||
15.3.6.1.1. Strontium isotopes | 8093 | ||
15.3.6.1.2. Barium isotopes | 8093 | ||
15.3.6.1.3. Cerium isotopes | 8093 | ||
15.3.6.1.4. Neodymium isotopes | 8094 | ||
15.3.6.1.5. Hafnium isotopes | 8094 | ||
15.3.6.1.6. Osmium isotopes | 8094 | ||
15.3.6.1.7. Lead isotopes | 8094 | ||
15.3.6.2. Radioactive Isotopic Reference Materials | 8094 | ||
15.3.6.2.1. Uranium isotopes | 8095 | ||
15.3.6.2.2. Thorium isotopes | 8095 | ||
15.3.6.3. Stable Isotopic Reference Materials | 8095 | ||
15.3.6.3.1. Hydrogen isotopes | 8095 | ||
15.3.6.3.2. Lithium isotopes | 8097 | ||
15.3.6.3.3. Boron isotopes | 8097 | ||
15.3.6.3.4. Carbon isotopes | 8097 | ||
15.3.6.3.5. Nitrogen isotopes | 8097 | ||
15.3.6.3.6. Oxygen isotopes | 8097 | ||
15.3.6.3.7. Magnesium isotopes | 8097 | ||
15.3.6.3.8. Silicon isotopes | 8098 | ||
15.3.6.3.9. Sulfur isotopes | 8098 | ||
15.3.6.3.10. Chlorine isotopes | 8098 | ||
15.3.6.3.11. Calcium isotopes | 8098 | ||
15.3.6.3.12. Titanium isotopes | 8098 | ||
15.3.6.3.13. Vanadium isotopes | 8098 | ||
15.3.6.3.14. Chromium isotopes | 8098 | ||
15.3.6.3.15. Iron isotopes | 8098 | ||
15.3.6.3.16. Nickel isotopes | 8098 | ||
15.3.6.3.17. Copper isotopes | 8099 | ||
15.3.6.3.18. Zinc isotopes | 8099 | ||
15.3.6.3.19. Germanium isotopes | 8099 | ||
15.3.6.3.20. Selenium isotopes | 8099 | ||
15.3.6.3.21. Rubidium isotopes | 8099 | ||
15.3.6.3.22. Strontium isotopes | 8099 | ||
15.3.6.3.23. Zirconium isotopes | 8099 | ||
15.3.6.3.24. Molybdenum isotopes | 8099 | ||
15.3.6.3.25. Cadmium isotopes | 8099 | ||
15.3.6.3.26. Silver isotopes | 8099 | ||
15.3.6.3.27. Antimony isotopes | 8099 | ||
15.3.6.3.28. Tungsten isotopes | 8099 | ||
15.3.6.3.29. Rhenium isotopes | 8100 | ||
15.3.6.3.30. Mercury isotopes | 8100 | ||
15.3.6.3.31. Thallium isotopes | 8100 | ||
15.3.7. GeoReM Database | 8100 | ||
15.3.8. Successes and Needs | 8100 | ||
Acknowledgments | 8101 | ||
References | 8101 | ||
Chapter 15.4: Application of Isotope Dilution in Geochemistry | 8106 | ||
15.4.1. Introduction | 8106 | ||
15.4.2. Applications of Isotope Dilution | 8106 | ||
15.4.2.1. Determination of Accurate and Precise Element Concentrations | 8106 | ||
15.4.2.2. `Double Spiking´ for IC Measurements | 8107 | ||
15.4.3. Principles of Isotope Dilution | 8107 | ||
15.4.3.1. Derivation of the Principal ID Equation | 8107 | ||
15.4.3.2. Criteria for Spiking Samples | 8109 | ||
15.4.3.3. The Optimum Spike-Sample Ratio | 8109 | ||
15.4.3.4. Sources of Uncertainty in ID Measurements | 8110 | ||
15.4.4. Applying Isotope Dilution | 8111 | ||
15.4.4.1. Preparation and Calibration of Spikes | 8111 | ||
15.4.4.2. Mixed Spikes Versus Single Spikes | 8112 | ||
15.4.4.3. Simultaneous IC and ID: `Spike Stripping´ | 8113 | ||
15.4.5. Double and Triple Spiking | 8115 | ||
15.4.6. Conclusions | 8119 | ||
Acknowledgments | 8120 | ||
References | 8120 | ||
Chapter 15.5: Sample Digestion Methods | 8122 | ||
15.5.1. Introduction | 8122 | ||
15.5.2. General Considerations | 8123 | ||
15.5.2.1. Mineral Acids | 8123 | ||
15.5.2.1.1. Hydrofluoric acid | 8123 | ||
15.5.2.1.2. Nitric acid | 8123 | ||
15.5.2.1.3. Aqua regia | 8123 | ||
15.5.2.1.4. Hydrochloric acid | 8124 | ||
15.5.2.1.5. Perchloric acid | 8124 | ||
15.5.2.1.6. Sulfuric acid | 8124 | ||
15.5.2.1.7. Phosphoric acid | 8124 | ||
15.5.2.2. Digestion Vessel Materials | 8124 | ||
15.5.2.3. Contamination from the Digestion Process | 8124 | ||
15.5.2.4. Assessing a Digestion Procedure | 8125 | ||
15.5.3. Sample Digestion Methods | 8126 | ||
15.5.3.1. Open Vessel Acid Digestions | 8126 | ||
15.5.3.2. Closed Vessel Digestions | 8127 | ||
15.5.3.3. Microwave Digestions | 8128 | ||
15.5.3.4. Partial Dissolutions | 8130 | ||
15.5.3.5. Dry Ashing Techniques | 8130 | ||
15.5.3.6. Alkali Fusions | 8132 | ||
15.5.3.6.1. Fusion with NaOH and KOH | 8132 | ||
15.5.3.6.2. Sinter or fusion with Na2O2 | 8132 | ||
15.5.3.6.3. Fusion with LiBO2 and Li2B4O7 | 8133 | ||
15.5.3.6.4. Fusion with Na2CO3 | 8133 | ||
15.5.3.7. Fire Assays | 8134 | ||
15.5.3.7.1. Lead fire assay | 8134 | ||
15.5.3.7.2. Nickel fire assay | 8134 | ||
15.5.3.8. Carius Tube and High-Pressure Asher (HPA-S) | 8135 | ||
15.5.3.9. Direct Fusion of Rock Powder | 8136 | ||
15.5.3.9.1. Flux-free fusion glasses | 8137 | ||
15.5.3.9.2. Lithium-borate fusion glass | 8137 | ||
15.5.4. Summary and Overview | 8138 | ||
Acknowledgments | 8138 | ||
References | 8138 | ||
Chapter 15.6: Developments in Clean Lab Practices | 8146 | ||
15.6.1. Introduction | 8146 | ||
15.6.2. Detection and Quantification Limits | 8148 | ||
15.6.2.1. Limit of Detection | 8148 | ||
15.6.2.2. Procedural Blank | 8149 | ||
15.6.3. Design of a Clean Room | 8149 | ||
15.6.3.1. Materials | 8150 | ||
15.6.3.2. Clean Air Supply | 8150 | ||
15.6.3.3. Pure Water Supply | 8150 | ||
15.6.4. Clean Lab Equipment, Labware&I | 8151 | ||
15.6.4.1. Laminar Flow Boxes | 8151 | ||
15.6.4.2. Labware | 8151 | ||
15.6.4.3. Sub boiling Distillation of Reagents | 8153 | ||
15.6.5. Examples of Low-Level Blank &I | 8154 | ||
15.6.5.1. Uranium-Lead Zircon Dating | 8154 | ||
15.6.5.2. Strontium Isotopic Analysis by Micromilling | 8154 | ||
15.6.5.3. Palladium-Silver Analysis of Meteorites | 8155 | ||
15.6.5.4. High-Field-Strength Elements and Tungsten | 8155 | ||
15.6.5.5. Rhenium-Osmium Systematics | 8155 | ||
15.6.5.6. Trace Element Contamination-Lithium Example | 8156 | ||
15.6.6. Concluding Remarks | 8156 | ||
Acknowledgments | 8156 | ||
References | 8156 | ||
Chapter 15.7: Basics of Ion Exchange Chromatography for Selected Geological Applications | 8158 | ||
15.7.1. Introduction | 8159 | ||
15.7.2. Basic Principles of Ion Chromatography | 8159 | ||
15.7.2.1. Stationary Phase-Mobile Phase | 8159 | ||
15.7.2.2. Structure of Ion Exchange Resins | 8159 | ||
15.7.2.3. Ion Exchange Processes | 8160 | ||
15.7.2.3.1. The equilibrium distribution coefficient (Kd) | 8160 | ||
15.7.2.3.2. The batch method | 8161 | ||
15.7.2.3.3. The column method | 8161 | ||
15.7.2.3.4. Retention volume and retention time | 8162 | ||
15.7.2.3.5. The ion exchange capacity of a resin | 8162 | ||
15.7.2.3.6. The capacity factor | 8163 | ||
15.7.2.3.7. Selectivity, separation factor, and selectivity coefficient: the efficiency of an ion exchange column to separate | 8163 | ||
15.7.2.3.8. Minimizing peak broadening: van Deemter&INS id= | 8163 | ||
15.7.3. Cation Exchange Versus Anion Exchange Chromatography | 8164 | ||
15.7.3.1. Structure and Basic Functionality of Cation and Anion Exchange Resins | 8164 | ||
15.7.3.2. Equilibrium Distribution Coefficients (Kd) for Cation and Anion Exchange Resins | 8165 | ||
15.7.3.2.1. Equilibrium distribution coefficients for strong cation exchange resins | 8165 | ||
15.7.3.2.2. Equilibrium distribution coefficients for strong anion exchange resins | 8165 | ||
15.7.4. Applications of Anion and Cation Exchange Chromatography for Element Enrichment and Purification Prior to High-&INS i | 8166 | ||
15.7.4.1. General Considerations for Chromatographic Ion Exchange Separation Procedures | 8166 | ||
15.7.4.2. General Laboratory Procedure of Ion Exchange Separations | 8173 | ||
15.7.4.3. The Setup of Ion Exchange Separation Methods: Calibration and Verification of Accuracy | 8173 | ||
15.7.4.4. Procedural Blanks | 8174 | ||
15.7.4.5. Selected Examples of Geological Applications Using Strong Cation or Anion Exchange Resin in Combina | 8174 | ||
15.7.4.5.1. Lithium | 8178 | ||
15.7.4.5.2. Chromium | 8178 | ||
15.7.4.5.3. Iron | 8178 | ||
15.7.4.5.4. Silver | 8178 | ||
15.7.4.5.5. Neodymium | 8179 | ||
15.7.5. Concluding Remarks | 8179 | ||
References | 8179 | ||
Chapter 15.8: Separation Methods Based on Liquid-Liquid Extraction, Extraction Chromatography, and Other Miscellaneous Solid Phase Extraction | 8182 | ||
15.8.1. Introduction | 8182 | ||
15.8.2. Separations by Liquid-Liquid Extraction | 8183 | ||
15.8.2.1. General | 8183 | ||
15.8.2.2. Experimental | 8184 | ||
15.8.2.3. Some Examples of Geochemical Applications | 8184 | ||
15.8.3. Extraction Chromatography | 8186 | ||
15.8.3.1. General Principle | 8186 | ||
15.8.3.2. Preparation of EXC Materials and Columns | 8187 | ||
15.8.3.2.1. General | 8187 | ||
15.8.3.2.2. EXC materials | 8187 | ||
15.8.3.2.2.1. Inert support | 8187 | ||
15.8.3.2.2.2. Loading of the stationary phase onto the inert support | 8188 | ||
15.8.3.2.3. Column packing | 8188 | ||
15.8.3.3. Examples of EXC Materials | 8188 | ||
15.8.3.3.1. Sr resin | 8188 | ||
15.8.3.3.2. Pb resin | 8190 | ||
15.8.3.3.3. TRU resin | 8190 | ||
15.8.3.3.4. RE resin | 8190 | ||
15.8.3.3.5. TEVA resin | 8192 | ||
15.8.3.3.6. UTEVA resin | 8192 | ||
15.8.3.3.7. Actinide (DIPEX) resin | 8192 | ||
15.8.3.3.8. Ln resin | 8193 | ||
15.8.3.3.9. `DGA resins | 8194 | ||
15.8.3.3.10. Miscellaneous, not marketed EXC materials | 8194 | ||
15.8.3.3.10.1. BPHA resin | 8194 | ||
15.8.3.3.10.2. DIBK resin | 8194 | ||
15.8.3.4. Some Examples of Applications in Geochemistry and Cosmochemistry | 8194 | ||
15.8.3.5. Advantages and Limitations of Extraction Chromatography | 8197 | ||
15.8.3.5.1. Advantages | 8197 | ||
15.8.3.5.2. Limitations | 8200 | ||
Chapter 15.9: Principles of Atomic Spectroscopy | 8206 | ||
15.9.1. Introduction and Terminology | 8206 | ||
15.9.2. Some History | 8206 | ||
15.9.3. Principles of Atomic Spectroscopy: Electromagnetic Radiation | 8207 | ||
15.9.4. Origin of Atomic Spectra | 8209 | ||
15.9.5. Analytical Applications of Atomic Spectroscopy | 8210 | ||
15.9.5.1. Atomic Absorption | 8210 | ||
15.9.5.2. Atomic Emission | 8210 | ||
15.9.5.3. X-Ray Fluorescence | 8211 | ||
15.9.5.4. Mass Spectrometry | 8212 | ||
15.9.5.5. Mass Spectrometer Ion Source | 8212 | ||
15.9.5.6. Mass Dispersion Devices | 8213 | ||
15.9.5.6.1. Magnetic sector | 8213 | ||
15.9.5.6.2. Quadrupole mass analyzer | 8213 | ||
15.9.6. Characteristics of Analytical Atomic Spectrometry Instruments | 8213 | ||
15.9.6.1. Atomic Absorption Spectrometry | 8214 | ||
15.9.6.2. X-Ray Fluorescence Analysis | 8214 | ||
15.9.6.3. Inductively Coupled Plasma-Atomic Emission Spectrometry | 8214 | ||
15.9.6.4. Inductively Coupled Plasma-Mass Spectrometry | 8215 | ||
References | 8215 | ||
Chapter 15.10: x-Ray Fluorescence Spectroscopy for Geochemistry | 8216 | ||
15.10.1. Principles | 8217 | ||
15.10.1.1. Introduction | 8217 | ||
15.10.1.2. x-Ray Generation | 8217 | ||
15.10.1.3. Primary and Fluorescent x-Ray | 8218 | ||
15.10.1.4. Excitation Energy | 8218 | ||
15.10.1.5. x-Ray Absorption and Analytic Depth | 8219 | ||
15.10.1.6. x-Ray Safety | 8219 | ||
15.10.2. Instrumentation | 8219 | ||
15.10.2.1. Measuring Systems | 8219 | ||
15.10.2.1.1. Wavelength dispersive system | 8219 | ||
15.10.2.1.2. Energy dispersive system | 8220 | ||
15.10.2.1.3. Portable handheld device | 8220 | ||
15.10.2.2. x-Ray Source | 8220 | ||
15.10.2.3. x-Ray Detection | 8220 | ||
15.10.2.4. x-Ray Filter | 8221 | ||
15.10.2.5. Pulse-Height Analyzer | 8221 | ||
15.10.2.6. Measuring Atmosphere | 8221 | ||
15.10.2.7. Analyzing Crystal for WDS | 8221 | ||
15.10.3. Sample Preparation | 8221 | ||
15.10.3.1. Criteria of Specimen | 8221 | ||
15.10.3.2. Direct Measurement | 8222 | ||
15.10.3.3. Specimen with Sample Pulverization | 8222 | ||
15.10.3.3.1. Sample pulverization | 8222 | ||
15.10.3.3.2. Loose powder analyses | 8223 | ||
15.10.3.3.3. Pressed powder pellet analyses | 8223 | ||
15.10.3.3.4. Fused glass bead analyses | 8223 | ||
15.10.3.4. Liquid Sample | 8223 | ||
15.10.3.5. Contamination | 8224 | ||
15.10.4. Qualitative Analysis | 8224 | ||
15.10.4.1. x-Ray Spectrum | 8224 | ||
15.10.4.2. Special Lines | 8224 | ||
15.10.4.3. Spectral Interference | 8224 | ||
15.10.4.4. Peak Identification | 8224 | ||
15.10.5. Quantitative Analysis | 8224 | ||
15.10.5.1. x-Ray Intensity | 8224 | ||
15.10.5.1.1. Analytic line | 8224 | ||
15.10.5.1.2. XRF intensity for determination | 8225 | ||
15.10.5.1.3. Standardization for routine analysis | 8225 | ||
15.10.5.1.4. x-Ray statistics | 8225 | ||
15.10.5.2. Standardless Determination | 8225 | ||
15.10.5.3. Determination with Calibration Standard | 8225 | ||
15.10.5.3.1. Criteria of standard specimen | 8225 | ||
15.10.5.3.2. Commercial standards | 8226 | ||
15.10.5.3.3. Synthetic standards | 8226 | ||
15.10.5.3.4. In-house standards | 8226 | ||
15.10.5.3.5. Calibration curve | 8226 | ||
15.10.5.4. Matrix Effect | 8227 | ||
15.10.5.5. Matrix Corrections | 8227 | ||
15.10.5.5.1. Standard addition | 8227 | ||
15.10.5.5.2. Internal standard | 8227 | ||
15.10.5.5.3. Correction with scattering x-rays | 8227 | ||
15.10.5.5.4. Model calculations | 8228 | ||
15.10.5.6. Validation | 8228 | ||
15.10.5.7. Applications | 8228 | ||
15.10.6. Further Techniques | 8228 | ||
15.10.6.1. Total Reflection for Surface Analysis | 8228 | ||
15.10.6.2. Chemical Shift for Chemical State Analysis | 8228 | ||
References | 8229 | ||
Chapter 15.11: Raman and Nuclear Resonant Spectroscopy in Geosciences | 8230 | ||
15.8.4. Other Element-Specific SPE Materials | 8201 | ||
15.8.4.1. A Few Selected Examples of Chelating Resins | 8201 | ||
15.8.4.1.1. Boron-specific resin | 8201 | ||
15.8.4.1.2. Precious elements specific resins | 8201 | ||
15.8.4.1.3. Chelating resins for trace element separation from (sea-) water | 8201 | ||
15.8.4.2. SPE Materials Based on Macrocyclic Ligands | 8202 | ||
15.8.5. Suggestions for Future Trends | 8202 | ||
References | 8203 | ||
15.11.1. Introduction | 8230 | ||
15.11.1.1. Interaction of Electromagnetic Waves and Matter | 8231 | ||
15.11.1.2. General Raman and Nuclear Resonant Spectroscopy | 8232 | ||
15.11.1.3. Electronic Structures and Crystal Field Theory | 8232 | ||
15.11.2. Raman Spectroscopy | 8233 | ||
15.11.2.1. Theory of Vibrational Properties of Molecules and Crystals | 8233 | ||
15.11.2.2. Instrumentation for Raman Experiments | 8234 | ||
15.11.2.2.1. Raman microprobe and mapping | 8234 | ||
15.11.2.2.2. Raman spectroscopy in high P-T DAC | 8235 | ||
15.11.2.2.3. Raman instrumentation for planetary e&/ | 8236 | ||
15.11.2.3. Raman Analyses of Planetary Materials | 8236 | ||
15.11.2.3.1. Phase identification in natural and synthetic samples | 8237 | ||
15.11.2.3.2. Raman microprobe study of meteorites and lunar samples | 8237 | ||
15.11.2.3.3. Laboratory studies at high P-T | 8237 | ||
15.11.3. Synchrotron MS and NRIXS | 8238 | ||
15.11.3.1. Mössbauer Effect and MS | 8239 | ||
15.11.3.1.1. Chemical shift | 8239 | ||
15.11.3.1.2. Quadrupole splitting | 8239 | ||
15.11.3.1.3. Magnetic hyperfine splitting | 8239 | ||
15.11.3.1.4. Recoil-free fraction | 8240 | ||
15.11.3.2. Mössbauer Instrumentation Using Conventional and Synchrotron Sources | 8240 | ||
15.11.3.3. Nuclear Resonant Scattering Using Synchrotron Radiation | 8240 | ||
15.11.3.4. Geophysical Applications | 8242 | ||
15.11.3.4.1. Sound velocities of iron alloys by NRIXS | 8243 | ||
15.11.3.4.2. Electronic spin and valence states by SMS | 8243 | ||
15.11.4. Prospective Directions | 8243 | ||
Acknowledgments | 8244 | ||
References | 8244 | ||
Chapter 15.12: Synchrotron x-Ray Spectroscopic Analysis | 8248 | ||
15.12.1. Introduction | 8248 | ||
15.12.2. High-Energy Synchrotrons | 8248 | ||
15.12.3. Synchrotron Radiation Sources | 8250 | ||
15.12.4. Synchrotron Beamlines | 8251 | ||
15.12.5. XAFS Analysis | 8252 | ||
15.12.6. XRM Analysis | 8254 | ||
15.12.6.1. XRM Instrumentation | 8255 | ||
15.12.6.2. XRF Microanalysis | 8255 | ||
15.12.6.3. Micro-XAFS Analysis | 8257 | ||
15.12.6.4. Micro-XRD Analysis | 8260 | ||
15.12.7. Computed Microtomography | 8260 | ||
15.12.8. Surface and Interface Methods | 8262 | ||
15.12.9. Other Synchrotron Methods | 8263 | ||
15.12.10. Future Directions | 8263 | ||
Acknowledgments | 8263 | ||
References | 8263 | ||
Chapter 15.13: Transmission Electron Microscope-Based Spectroscopy | 8266 | ||
Glossary | 8266 | ||
15.13.1. Introduction | 8266 | ||
15.13.2. TEM Design Considerations | 8268 | ||
15.13.2.1. TEM or STEM | 8268 | ||
15.13.2.2. Thermionic and Field Emission Electron Sources | 8269 | ||
15.13.2.3. Energy Spread and Monochromators | 8269 | ||
15.13.2.4. Operating Voltage | 8269 | ||
15.13.3. Energy-Dispersive x-Ray Spectroscopy and Electron Energy-Loss Spectroscopy Instrumentation | 8270 | ||
15.13.3.1. EDS Detectors | 8270 | ||
15.13.3.2. Electron Energy-Loss Spectrometers | 8270 | ||
15.13.4. Sample Preparation | 8270 | ||
15.13.5. Energy-Dispersive x-Ray Spectroscopy Examples | 8272 | ||
15.13.6. Electron Energy-Loss Spectroscopy Examples | 8277 | ||
References | 8279 | ||
Chapter 15.4: Laser-Induced Breakdown Spectroscopy | 8280 | ||
15.14.1. Introduction and Overview | 8280 | ||
15.14.2. The LIBS Analysis | 8281 | ||
15.14.3. LIBS Fundamentals | 8282 | ||
15.14.3.1. The Physics of LIBS | 8282 | ||
15.14.3.2. Spatial Resolution | 8287 | ||
15.14.3.3. The Chemistry of LIBS | 8288 | ||
15.14.3.3.1. Quantitative LIBS | 8288 | ||
15.14.3.3.2. Qualitative LIBS | 8289 | ||
15.14.4. Laboratory, Field-Portable, and Standoff LIBS Analysis | 8290 | ||
15.14.5. Example Applications of LIBS for Natural Material Analysis | 8291 | ||
15.14.5.1. Minerals and Rocks | 8291 | ||
15.14.5.2. Sediment and Soil Analysis | 8293 | ||
15.14.5.3. Fluid Analysis | 8295 | ||
15.14.5.4. Fluid Inclusion Analysis | 8296 | ||
15.14.5.5. Material Analysis under Water | 8297 | ||
15.14.5.6. Environmental Applications | 8297 | ||
15.14.5.7. Archaeological Applications | 8298 | ||
15.14.5.8. Extraterrestrial Analysis | 8298 | ||
15.14.5.9. Isotopic Analysis by LIBS | 8299 | ||
15.14.6. Statistical Signal Processing for LIBS | 8299 | ||
15.14.7. Conclusions - The Path Forward | 8301 | ||
Acknowledgments | 8302 | ||
References | 8302 | ||
Chapter 15.15: Nuclear Spectroscopy | 8308 | ||
15.15.1. Introduction | 8308 | ||
15.15.2. The Discovery of Radioactivity | 8309 | ||
15.15.3. The Atomic Nucleus, Isotopes, and Radionuclides | 8309 | ||
15.15.4. Radioactive Decay | 8310 | ||
15.15.4.1. Modes of Radioactive Decay | 8310 | ||
15.15.4.2. Radioactive Decay Law | 8310 | ||
15.15.4.3. Units of Radioactivity | 8311 | ||
15.15.4.4. Growth and Decay of Radioactive Products | 8311 | ||
15.15.5. Nuclear Reactions | 8312 | ||
15.15.5.1. The Reaction Process | 8312 | ||
15.15.5.2. Compound Nucleus Formation | 8313 | ||
15.15.5.3. Reaction Cross Sections | 8313 | ||
15.15.5.4. Excitation Functions | 8314 | ||
15.15.5.5. Growth of Radioactive Products During Irradiation | 8314 | ||
15.15.6. Irradiation Sources | 8314 | ||
15.15.6.1. Nuclear Reactors | 8315 | ||
15.15.6.2. Neutron Generators | 8316 | ||
15.15.6.3. Charged-Particle Accelerators | 8316 | ||
15.15.6.4. Radioisotopic Sources | 8316 | ||
15.15.7. Interactions Between Radiation and Matter | 8316 | ||
15.15.7.1. Interaction of Heavy-Charged Particles with Matter | 8316 | ||
15.15.7.2. Interaction of Electrons and Positrons with Matter | 8317 | ||
15.15.7.3. Interaction of Gamma Rays with Matter | 8317 | ||
15.15.7.4. Interaction of Neutrons with Matter | 8318 | ||
15.15.8. Radiation Detection and Measurement | 8318 | ||
15.15.8.1. General Properties of Detectors | 8319 | ||
15.15.8.2. Scintillation Detectors | 8319 | ||
15.15.8.3. Semiconductor Detectors | 8320 | ||
15.15.8.4. Shielding and Compton Suppression | 8320 | ||
15.15.9. Applications for Nuclear Spectroscopy | 8321 | ||
15.15.9.1. Activation Analysis | 8321 | ||
15.15.9.1.1. Preparation of samples and standards for activation analysis | 8321 | ||
15.15.9.1.2. Irradiation and counting techniques | 8322 | ||
15.15.9.1.3. Activation analysis with thermal neutrons | 8322 | ||
15.15.9.1.4. Activation analysis with epithermal neutrons | 8323 | ||
15.15.9.1.5. Activation analysis with fast neutrons | 8323 | ||
15.15.9.1.6. Activation analysis using prompt gamma rays | 8323 | ||
15.15.9.1.7. Activation analysis with charged-particle beams | 8323 | ||
15.15.9.1.8. Activation analysis with high-energy photons | 8324 | ||
15.15.9.1.9. Activation analysis with radiochemical separation | 8324 | ||
15.15.9.1.10. Activation analysis with gamma-gamma coincidence | 8324 | ||
15.15.9.2. Other Applications of Nuclear Spectroscopy | 8325 | ||
References | 8325 | ||
Chapter 15.16: Stable Isotope Techniques for Gas Source Mass Spectrometry | 8326 | ||
15.16.1. Introduction | 8326 | ||
15.16.2. Mass Spectrometers | 8326 | ||
15.16.2.1. Inlet System | 8327 | ||
15.16.2.1.1. Dual inlet | 8327 | ||
15.16.2.1.2. Continuous flow inlet | 8328 | ||
15.16.2.2. Ion Source | 8330 | ||
15.16.2.3. Analyzer | 8331 | ||
15.16.2.4. Collector Assembly | 8332 | ||
15.16.3. Standardization | 8333 | ||
15.16.4. Methods of Analysis | 8334 | ||
15.16.4.1. Hydrogen | 8334 | ||
15.16.4.1.1. Reduction methods | 8335 | ||
15.16.4.1.2. Equilibration methods | 8335 | ||
15.16.4.2. Carbon | 8335 | ||
15.16.4.3. Nitrogen | 8336 | ||
15.16.4.4. Oxygen | 8336 | ||
15.16.4.5. Sulfur | 8337 | ||
15.16.4.6. Chlorine | 8338 | ||
15.16.5. Laser Absorption Spectrometry | 8338 | ||
References | 8340 | ||
Chapter 15.17: Inductively Coupled Plasma Mass Spectrometers | 8344 | ||
15.17.1. Introduction | 8346 | ||
15.17.2. Sample Preparation | 8348 | ||
15.17.3. Sample Introduction and Ion Production | 8349 | ||
15.17.4. Sampler/Skimmer Interface | 8352 | ||
15.17.5. ICP-MS with Quadrupole Mass Spectrometers | 8352 | ||
15.17.6. Spectral Overlaps in ICP-MS | 8352 | ||
15.17.6.1. Elemental Ions | 8353 | ||
15.17.6.2. Molecular Ions | 8353 | ||
15.17.6.3. Doubly Charged Ions | 8354 | ||
15.17.6.4. Recognizing that Spectral Overlaps Exist | 8354 | ||
15.17.6.5. Preventing Errors in Reported Concentrations Due to Spectral Overlaps | 8355 | ||
15.17.6.5.1. Choice of isotopes to measure | 8355 | ||
15.17.6.5.2. Mathematical corrections: Elemental ions | 8355 | ||
15.17.6.5.3. Mathematical corrections: Molecular ions | 8355 | ||
15.17.6.5.4. Desolvation system to reduce solvent-related ion signals | 8356 | ||
15.17.6.5.5. Modifying plasma conditions to reduce molecular ion signals: Addition of molecular gas to the plasma | 8356 | ||
15.17.6.5.7. Chemical separation prior to analysis | 8356 | ||
15.17.6.5.8. Kinetic energy discrimination using a collision/reaction cell | 8356 | ||
15.17.6.5.9. Ion-molecule reactions using a collision/reaction cell | 8357 | ||
15.17.6.5.6. Modifying plasma conditions to reduce signals from ions with high ionization energy: &INS id= | 8356 | ||
15.17.7. Collision/Reaction Cells to Overcome Spectral Overlaps in ICP-Quadrupole MS | 8357 | ||
15.17.7.1. Kinetic Energy Discrimination | 8357 | ||
15.17.7.2. Ion-Molecule Reactions | 8359 | ||
15.17.8. ICP-MS Instrument Designs with a Quadrupole Mass Analyzer | 8359 | ||
15.17.9. ICP-Sector Field Mass Spectrometers | 8360 | ||
15.17.9.1. ICP-SFMS with Sequential Detection | 8360 | ||
15.17.10. ICP-MS Instruments with Simultaneous Detection of the Mass Spectrum | 8365 | ||
15.17.10.1. ICP-Sector Field Mass Spectrometer with Simultaneous Imaging Detector | 8365 | ||
15.17.10.2. ICP-Time of Flight Mass Spectrometer | 8365 | ||
15.17.11. Multicollector Inductively Coupled Plasma Mass Spectrometers | 8365 | ||
15.17.11.1. Spectral Overlaps | 8367 | ||
15.17.11.2. Fixed or Variable Position Detectors | 8367 | ||
15.17.11.3. Static and Dynamic Measurements | 8367 | ||
15.17.11.4. Mass Discrimination (Mass Bias) | 8368 | ||
15.17.11.4.1. Double or triple spike correction for mass bias | 8368 | ||
15.17.11.4.2. Internal standardization using an analyte isotope pair that is constant in nature | 8368 | ||
15.17.11.4.3. Internal standardization using a pair of isotopes of another element | 8368 | ||
15.17.11.4.4. Internal standardization with isotopes of another element using a regression approach | 8368 | ||
15.17.11.4.5. External standard sample standard bracketing | 8369 | ||
15.17.11.4.6. Isotope ratio uncertainties | 8369 | ||
15.17.11.5. Applications | 8369 | ||
References | 8369 | ||
Chapter 15.18: Thermal Ionization Mass Spectrometry | 8372 | ||
15.18.1. Introduction | 8372 | ||
15.18.2. Why TIMS Survives | 8372 | ||
15.18.2.1. Strengths | 8372 | ||
15.18.2.2. Weaknesses | 8373 | ||
15.18.3. Thermal Ionization | 8374 | ||
15.18.4. The Physical TIMS Instrument | 8376 | ||
15.18.4.1. The Ion Source | 8376 | ||
15.18.4.2. The Mass Analyzer | 8377 | ||
15.18.4.3. Ion Detectors | 8380 | ||
15.18.4.3.1. Faraday detectors | 8380 | ||
15.18.4.3.2. Ion multipliers | 8382 | ||
15.18.5. Measuring Isotope Ratios by TIMS | 8384 | ||
15.18.5.1. Noise | 8384 | ||
15.18.5.2. Time Variability of the Ion Beam | 8384 | ||
15.18.5.3. Correcting for Amplifier and Faraday Cup Gains | 8384 | ||
15.18.5.4. Fractionation Correction | 8385 | ||
15.18.6. Conclusions and Future Prospects | 8387 | ||
Acknowledgments | 8388 | ||
References | 8388 | ||
Chapter 15.19: Noble Gas Mass Spectrometry | 8390 | ||
15.19.1. Introduction | 8390 | ||
15.19.1.1. Noble Gases in Geochemistry and Cosmochemistry | 8390 | ||
15.19.2. Characteristics of Noble Gas Mass Spectrometry | 8391 | ||
15.19.3. Types of Samples, Noble Gas Extraction and Purification | 8392 | ||
15.19.3.1. Solid Samples | 8393 | ||
15.19.3.1.1. Total extraction of noble gases from solid samples | 8394 | ||
15.19.3.1.2. Stepwise noble gas extraction from solid samples | 8394 | ||
15.19.3.2. Liquid Samples | 8395 | ||
15.19.3.3. Gaseous Samples | 8395 | ||
15.19.3.4. Noble Gas Purification | 8396 | ||
15.19.3.5. Separation of Noble Gases from Each Other | 8397 | ||
15.19.3.6. Nitrogen Analysis by Static Mass Spectrometry | 8397 | ||
15.19.4. Ionization, Mass Separation, and Ion Detection | 8397 | ||
15.19.4.1. Ionization | 8397 | ||
15.19.4.2. Mass Separation and Detection | 8397 | ||
15.19.5. Calibration | 8399 | ||
15.19.5.1. Spiking | 8399 | ||
15.19.5.2. Peak-Height Comparison | 8399 | ||
15.19.5.3. Standards | 8400 | ||
15.19.5.4. The Isotopic Composition of Noble Gases in Air | 8400 | ||
15.19.6. Blank and Interference Corrections | 8402 | ||
15.19.6.1. Noble Gas Blanks | 8402 | ||
15.19.6.2. Interference Corrections | 8402 | ||
15.19.7. Mass Spectrometer Memory and Ion Pumping | 8403 | ||
15.19.8. Outlook | 8404 | ||
Acknowledgments | 8405 | ||
References | 8405 | ||
Chapter 15.20: Accelerator Mass Spectrometry | 8410 | ||
15.20.1. Introduction | 8410 | ||
15.20.2. The AMS Instrument | 8410 | ||
15.20.3. Ion Source | 8411 | ||
15.20.4. Injection Magnet and Bouncer | 8412 | ||
15.20.5. Tandem Particle Accelerator and Stripper | 8413 | ||
15.20.6. High-Energy Particle Analysis | 8413 | ||
15.20.7. Particle Detection | 8414 | ||
15.20.8. Development of Smaller Machines | 8414 | ||
15.20.8.1. The 0.5-1MV `Small&INS i | 8414 | ||
15.20.8.2. 200kV Machines | 8415 | ||
15.20.8.3. The `Single-Stage&INS id= | 8416 | ||
15.20.9. Conclusion | 8416 | ||
References | 8417 | ||
Chapter 15.21: Ion Microscopes and Microprobes | 8420 | ||
15.21.1. Overview | 8420 | ||
15.21.2. Primary Ion Beams | 8421 | ||
15.21.2.1. Primary Ions | 8421 | ||
15.21.2.2. Primary Column and Beam Characteristics | 8422 | ||
15.21.3. Secondary Ions | 8423 | ||
15.21.3.1. Ion Yields | 8424 | ||
15.21.4. Mass Spectrometry | 8424 | ||
15.21.4.1. History of Ion Microprobe Mass Spectrometers | 8425 | ||
15.21.4.2. Nuclidic Mass Resolution | 8425 | ||
15.21.4.3. Mass Spectra | 8426 | ||
15.21.4.4. Energy Spectra | 8426 | ||
15.21.5. Instrumentation | 8427 | ||
15.21.5.1. Cameca Ion Microscopes (ims 7f and 1280 Series) | 8427 | ||
15.21.5.2. Cameca NanoSIMS | 8427 | ||
15.21.5.3. SHRIMP | 8428 | ||
15.21.6. Measurement | 8431 | ||
15.21.6.1. Detection | 8431 | ||
15.21.6.2. Ion Counting | 8431 | ||
15.21.6.3. 2D Detectors | 8432 | ||
15.21.6.4. Faraday Cups | 8432 | ||
15.21.6.5. Multiple Collection | 8433 | ||
15.21.7. Chemical Analysis | 8434 | ||
15.21.7.1. Volatile Abundance Measurements | 8434 | ||
15.21.8. Stable Isotope Analysis | 8435 | ||
15.21.8.1. Isotope Mass Fractionation | 8435 | ||
15.21.8.2. Sulfur Isotope Analysis | 8435 | ||
15.21.8.3. Oxygen Isotope Analysis | 8436 | ||
15.21.8.4. Carbon and Nitrogen | 8436 | ||
15.21.8.5. Lithium and Boron | 8437 | ||
15.21.8.6. Hydrogen | 8437 | ||
15.21.9. Radiogenic Isotopes | 8437 | ||
15.21.9.1. U-Th-Pb Geochronology | 8438 | ||
15.21.9.2. U-Th Disequilibrium | 8439 | ||
15.21.9.3. 176Lu-176Hf in Zircon | 8440 | ||
15.21.9.4. 40K-40Ca Measurements | 8440 | ||
15.21.9.5. Short-Lived Radionuclides | 8440 | ||
15.21.10. Isotopic Anomalies | 8441 | ||
15.21.10.1. Refractory Inclusions | 8441 | ||
15.21.10.2. Presolar Grains | 8441 | ||
15.21.11. Future Developments and Issues | 8442 | ||
References | 8442 | ||
Chapter 15.22: Time-of-Flight Secondary Ion Mass Spectrometry, Secondary Neutral Mass Spectrometry, and Resonance Ionization Mass Spectrometry | 8446 | ||
15.22.1. Introduction | 8446 | ||
15.22.2. Time-of-Flight Secondary Ion Mass Spectrometry | 8447 | ||
15.22.2.1. Technical Principles | 8447 | ||
15.22.2.2. Sample Considerations | 8448 | ||
15.22.2.3. Matrix Effects | 8448 | ||
15.22.2.4. Data Analysis | 8449 | ||
15.22.2.5. Quantification | 8450 | ||
15.22.2.6. Some Neat Little Tricks | 8450 | ||
15.22.2.7. Summary: Strengths and Weaknesses | 8450 | ||
15.22.2.8. Applications: Inorganic Geochemistry | 8451 | ||
15.22.2.8.1. TOF-SIMS in cosmochemistry | 8451 | ||
15.22.2.8.2. TOF-SIMS in space | 8451 | ||
15.22.3. Organic TOF-SIMS | 8451 | ||
15.22.3.1. Applications: Organic Geochemistry | 8452 | ||
15.22.3.1.1. TOF-SIMS in geomicrobiology | 8452 | ||
15.22.3.1.2. TOF-SIMS in organic cosmochemistry | 8452 | ||
15.22.4. New Developments of TOF-SIMS | 8453 | ||
15.22.4.1. Combination with DC Primary Ion Beams | 8453 | ||
15.22.5. Postionization | 8454 | ||
15.22.5.1. Resonance Ionization Mass Spectrometers | 8454 | ||
15.22.5.1.1. Noble gas resonance ionization mass spectrometers | 8455 | ||
15.22.5.1.2. Sputter-initiated resonance ionization mass spectrometry - Resonant ionization of sputte | 8456 | ||
15.22.5.2. Laser SNMS Applications | 8456 | ||
15.22.5.2.1. Isotopic and elemental analysis | 8456 | ||
15.22.5.3. Nonresonant Laser Postionization | 8457 | ||
References | 8457 | ||
Chapter 15.23: Laser Ablation ICP-MS and Laser Fluorination GS-MS | 8460 | ||
15.23.1. Introduction | 8460 | ||
15.23.2. Laser Processing | 8460 | ||
15.23.2.1. Bandgap Energies of Solid Materials | 8460 | ||
15.23.2.2. Laser Radiation and Photon-Substrate Coupling | 8461 | ||
15.23.2.3. Stoichiometric Laser Sampling | 8462 | ||
15.23.2.4. Commercial Laser Ablation Systems | 8463 | ||
15.23.3. Laser Ablation ICP-MS Methodology | 8464 | ||
15.23.3.1. Plasma Source Mass Spectrometry | 8464 | ||
15.23.3.1.1. Quadrupole ICP-MS | 8465 | ||
15.23.3.1.2. High-resolution ICP-MS | 8466 | ||
15.23.3.1.3. Multicollector ICP-MS | 8467 | ||
15.23.3.2. Quantitative LA-ICP-MS Analysis | 8467 | ||
15.23.3.2.1. Spectral matrix effects | 8467 | ||
15.23.3.2.2. Nonspectral matrix effects | 8468 | ||
15.23.3.2.3. Laser-induced elemental fractionations | 8469 | ||
15.23.3.2.4. Recent and future advances in LA-ICP-MS technology and applications | 8470 | ||
15.23.4. Laser Fluorination Mass Spectr | 8470 | ||
15.23.4.1. The Advent of Laser Fluorinati | 8470 | ||
15.23.4.2. Fundamental LF-GS-MS | 8471 | ||
15.23.4.3. Applications of Laser Fluorination M | 8472 | ||
15.23.5. Conclusions | 8472 | ||
References | 8473 | ||
Chapter 15.24: Geoneutrino Detection | 8478 | ||
15.24.1. Introduction | 8478 | ||
15.24.2. Neutrino Physics | 8478 | ||
15.24.2.1. Neutrinos in the Standard Model | 8478 | ||
15.24.2.2. Neutrino Reactions | 8480 | ||
15.24.3. Neutrino Detector Technologies | 8482 | ||
15.24.3.1. Charge Collection | 8482 | ||
15.24.3.2. Cherenkov Light | 8482 | ||
15.24.3.3. Scintillation Light | 8483 | ||
15.24.3.4. Liquid Scintillator Antineutrino Detector Design | 8483 | ||
15.24.4. Existing and Planned Geoneutrino Detectors | 8484 | ||
15.24.4.1. KamLAND | 8484 | ||
15.24.4.2. Borexino | 8485 | ||
15.24.4.3. SNO | 8485 | ||
15.24.4.4. Future Geoneutrino Detectors | 8485 | ||
15.24.5. Desired Future Developments | 8486 | ||
15.24.5.1. Global Survey | 8486 | ||
15.24.5.2. Directionality | 8486 | ||
15.24.5.3. Detection of 40K Geoneutrinos | 8487 | ||
15.24.6. Conclusions | 8487 | ||
References | 8487 | ||
e9780080983004v16 | 8490 | ||
Front Cover | 8490 | ||
Indexes | 8493 | ||
Copyright | 8494 | ||
In Memoriam | 8495 | ||
Heinrich Dieter Holland (1927–2012) | 8497 | ||
Karl Karekin Turekian (1927–2013) | 8499 | ||
References | 8501 | ||
Volume Editors | 8503 | ||
Contents | 8511 | ||
Contents of All Volumes | 8513 | ||
Contributors of All Volumes | 8529 | ||
Index | 8543 |