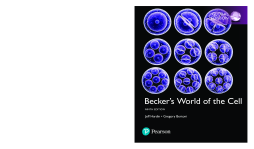
BOOK
Becker's World of the Cell, Global Edition
Jeff Hardin | Gregory Paul Bertoni | Lewis J. Kleinsmith
(2017)
Additional Information
Book Details
Abstract
For courses in cell biology.
Explore the world of the cell
Widely praised for its strong biochemistry coverage and clear, easy-to-follow explanations and figures, Becker’s World of the Cell provides a beautifully-illustrated, up-to-date introduction to cell biology concepts, processes, and applications. Informed by many years of classroom experience in the sophomore-level cell biology course, the dramatically-revised Ninth Edition introduces molecular genetics concepts earlier in the text and includes more extensive coverage of key techniques in each chapter. Becker’s World of the Cell provides accessible and authoritative descriptions of all major principles, as well as unique scientific insights into visualization and applications of cell and molecular biology.
MasteringBiology™not included. Students, if MasteringBiology is a recommended/mandatory component of the course, please ask your instructor for the correct ISBN and course ID. MasteringBiology should only be purchased when required by an instructor. Instructors, contact your Pearson representative for more information.
MasteringBiology is an online homework, tutorial, and assessment program designed to work with this text to engage students and improve results. Interactive, self-paced tutorials provide individualized coaching to help students stay on track. With a wide range of activities available, students can actively learn, understand, and retain even the most difficult concepts.
Table of Contents
Section Title | Page | Action | Price |
---|---|---|---|
Front Cover | Front Cover | ||
About the Authors | 3 | ||
Brief Contents | 4 | ||
Detailed Contents | 5 | ||
Preface | 18 | ||
Acknowledgments | 24 | ||
Chapter 1: A Preview of Cell Biology | 25 | ||
1.1: The Cell Theory: A Brief History | 26 | ||
Advances in Microscopy Allowed Detailed Studies of Cells | 26 | ||
The Cell Theory Applies to All Organisms | 26 | ||
1.2: The Emergence of Modern Cell Biology | 27 | ||
The Cytological Strand Deals with Cellular Structure | 29 | ||
The Biochemical Strand Studies the Chemistry of Biological Structure and Function | 33 | ||
The Genetic Strand Focuses on Information Flow | 35 | ||
1.3: How Do We Know What We Know? | 38 | ||
Biological “Facts” May Turn Out to Be Incorrect | 38 | ||
Experiments Test Specific Hypotheses | 38 | ||
Model Organisms Play a Key Role in Modern Cell Biology Research | 39 | ||
Well-Designed Experiments Alter Only One Variable at a Time | 41 | ||
Summary of Key Points | 42 | ||
Problem Set | 43 | ||
Key Technique: Using Immunofluorescence to Identify Specific Cell Components | 32 | ||
Human Connections: The Immortal Cells of Henrietta Lacks | 40 | ||
Chapter 2: The Chemistry of the Cell | 45 | ||
2.1: The Importance of Carbon | 46 | ||
Carbon-Containing Molecules Are Stable | 47 | ||
Carbon-Containing Molecules Are Diverse | 48 | ||
Carbon-Containing Molecules Can Form Stereoisomers | 49 | ||
2.2: The Importance of Water | 50 | ||
Water Molecules Are Polar | 51 | ||
Water Molecules Are Cohesive | 52 | ||
Water Has a High Temperature-Stabilizing Capacity | 52 | ||
Water Is an Excellent Solvent | 52 | ||
2.3: The Importance of Selectively Permeable Membranes | 55 | ||
A Membrane Is a Lipid Bilayer with Proteins Embedded in It | 55 | ||
Lipid Bilayers Are Selectively Permeable | 56 | ||
2.4: The Importance of Synthesis by Polymerization | 56 | ||
Macromolecules Are Critical for Cellular Form and Function | 56 | ||
Cells Contain Three Different Kinds of Macromolecular Polymers | 58 | ||
Macromolecules Are Synthesized by Stepwise Polymerization of Monomers | 59 | ||
2.5: The Importance of Self-Assembly | 60 | ||
Noncovalent Bonds and Interactions Are Important in the Folding of Macromolecules | 61 | ||
Many Proteins Spontaneously Fold into Their Biologically Functional State | 61 | ||
Molecular Chaperones Assist the Assembly of Some Proteins | 62 | ||
Self-Assembly Also Occurs in Other Cellular Structures | 62 | ||
The Tobacco Mosaic Virus Is a Case Study in Self-Assembly | 62 | ||
Self-Assembly Has Limits | 63 | ||
Hierarchical Assembly Provides Advantages for the Cell | 63 | ||
Summary of Key Points | 64 | ||
Problem Set | 65 | ||
Key Technique: Determining the Chemical Fingerprint of a Cell Using Mass Spectrometry | 50 | ||
Human Connections: Taking a Deeper Look: Magnetic Resonance Imaging (MRI) | 54 | ||
Chapter 3: The Macromolecules of the Cell | 66 | ||
3.1: Proteins | 67 | ||
The Monomers Are Amino Acids | 67 | ||
The Polymers Are Polypeptides and Proteins | 69 | ||
Several Kinds of Bonds and Interactions Are Important in Protein Folding and Stability | 70 | ||
Protein Structure Depends on Amino Acid Sequence and Interactions | 73 | ||
3.2: Nucleic Acids | 82 | ||
The Monomers Are Nucleotides | 82 | ||
The Polymers Are DNA and RNA | 83 | ||
A DNA Molecule Is a Double-Stranded Helix | 85 | ||
3.3: Polysaccharides | 86 | ||
The Monomers Are Monosaccharides | 86 | ||
The Polymers Are Storage and Structural Polysaccharides | 88 | ||
Polysaccharide Structure Depends on the Kinds of Glycosidic Bonds Involved | 90 | ||
3.4: Lipids | 90 | ||
Fatty Acids Are the Building Blocks of Several Classes of Lipids | 91 | ||
Triacylglycerols Are Storage Lipids | 93 | ||
Phospholipids Are Important in Membrane Structure | 93 | ||
Glycolipids Are Specialized Membrane Components | 94 | ||
Steroids Are Lipids with a Variety of Functions | 94 | ||
Terpenes Are Formed from Isoprene | 95 | ||
Summary of Key Points | 95 | ||
Problem Set | 96 | ||
Human Connections: Aggregated Proteins and Alzheimer’s | 71 | ||
Key Technique: Using X-Ray Crystallography to Determine Protein Structure | 80 | ||
Chapter 4: Cells and Organelles | 99 | ||
4.1: Where Did the First Cells Come From? | 99 | ||
Simple Organic Molecules May Have Formed Abiotically in the Young Earth | 99 | ||
RNA May Have Been the First Informational Molecule | 100 | ||
Liposomes May Have Defined the First Primitive Protocells | 101 | ||
4.2: Properties and Strategies of Cells | 101 | ||
All Organisms Are Bacteria, Archaea, or Eukaryotes | 101 | ||
There Are Several Limitations on Cell Size | 102 | ||
Bacteria, Archaea, and Eukaryotes Differ from Each Other in Many Ways | 104 | ||
4.3: The Eukaryotic Cell in Overview: Structure and Function | 107 | ||
The Plasma Membrane Defines Cell Boundaries and Retains Contents | 107 | ||
The Nucleus Is the Information Center of the Eukaryotic Cell | 108 | ||
Mitochondria and Chloroplasts Provide Energy for the Cell | 109 | ||
The Endosymbiont Theory Proposes That Mitochondria and Chloroplasts Were Derived From Bacteria | 111 | ||
The Endomembrane System Synthesizes Proteins for a Variety of Cellular Destinations | 113 | ||
Other Organelles Also Have Specific Functions | 116 | ||
Ribosomes Synthesize Proteins in the Cytoplasm | 119 | ||
The Cytoskeleton Provides Structure to the Cytoplasm | 119 | ||
The Extracellular Matrix and Cell Walls Are Outside the Plasma Membrane | 122 | ||
4.4: Viruses, Viroids, and Prions: Agents That Invade Cells | 123 | ||
A Virus Consists of a DNA or RNA Core Surrounded by a Protein Coat | 123 | ||
Viroids Are Small, Circular RNA Molecules That Can Cause Plant Diseases | 124 | ||
Prions Are Infectious Protein Molecules | 125 | ||
Summary of Key Points | 125 | ||
Problem Set | 126 | ||
Human Connections: When Cellular “Breakdown” Breaks Down | 116 | ||
Key Technique: Using Centrifugation to Isolate Organelles | 120 | ||
Chapter 5: Bioenergetics: The Flow of Energy in the Cell | 128 | ||
5.1: The Importance of Energy | 129 | ||
Cells Need Energy to Perform Six Different Kinds of Work | 129 | ||
Organisms Obtain Energy Either from Sunlight or from the Oxidation of Chemical Compounds | 130 | ||
Energy Flows Through the Biosphere Continuously | 131 | ||
The Flow of Energy Through the Biosphere Is Accompanied by a Flow of Matter | 133 | ||
5.2: Bioenergetics | 133 | ||
Understanding Energy Flow Requires Knowledge of Systems, Heat, and Work | 133 | ||
The First Law of Thermodynamics States That Energy Is Conserved | 134 | ||
The Second Law of Thermodynamics States That Reactions Have Directionality | 136 | ||
Entropy and Free Energy Are Two Means of Assessing Thermodynamic Spontaneity | 137 | ||
5.3: Understanding ∆G and Keq | 140 | ||
The Equilibrium Constant Keq Is a Measure of Directionality | 140 | ||
∆G Can Be Calculated Readily | 141 | ||
The Standard Free Energy Change Is ∆G Measured Under Standard Conditions | 141 | ||
Summing Up: The Meaning of ∆G´ and ∆G˚´ | 144 | ||
Free Energy Change: Sample Calculations | 145 | ||
Jumping Beans Provide a Useful Analogy for Bioenergetics | 146 | ||
Life Requires Steady-State Reactions That Move Toward Equilibrium Without Ever Getting There | 147 | ||
Summary of Key Points | 148 | ||
Problem Set | 149 | ||
Human Connections: The “Potential” of Food to Provide Energy | 135 | ||
Key Technique: Measuring How Molecules Bind to One Another Using Isothermal Titration Calorimetry | 142 | ||
Chapter 6: Enzymes: The Catalysts of Life | 151 | ||
6.1: Activation Energy and the Metastable State | 152 | ||
Before a Chemical Reaction Can Occur, the Activation Energy Barrier Must Be Overcome | 152 | ||
The Metastable State Is a Result of the Activation Barrier | 153 | ||
Catalysts Overcome the Activation Energy Barrier | 153 | ||
6.2: Enzymes as Biological Catalysts | 154 | ||
Most Enzymes Are Proteins | 154 | ||
Substrate Binding, Activation, and Catalysis Occur at the Active Site | 157 | ||
Ribozymes Are Catalytic RNA Molecules | 159 | ||
6.3: Enzyme Kinetics | 161 | ||
Monkeys and Peanuts Provide a Useful Analogy for Understanding Enzyme Kinetics | 161 | ||
Most Enzymes Display Michaelis–Menten Kinetics | 162 | ||
What Is the Meaning of Vmax and Km? | 163 | ||
Why Are Km and Vmax Important to Cell Biologists? | 164 | ||
The Double-Reciprocal Plot Is a Useful Means of Visualizing Kinetic Data | 164 | ||
Enzyme Inhibitors Act Either Irreversibly or Reversibly | 165 | ||
6.4: Enzyme Regulation | 168 | ||
Allosteric Enzymes Are Regulated by Molecules Other than Reactants and Products | 168 | ||
Allosteric Enzymes Exhibit Cooperative Interactions Between Subunits | 170 | ||
Enzymes Can Also Be Regulated by the Addition or Removal of Chemical Groups | 170 | ||
Summary of Key Points | 172 | ||
Problem Set | 173 | ||
Human Connections: ACE Inhibitors: Enzyme Activity as the Difference Between Life and Death | 160 | ||
Key Technique: Determining Km and Vmax Using Enzyme Assays | 166 | ||
Chapter 7: Membranes: Their Structure, Function, and Chemistry | 176 | ||
7.1: The Functions of Membranes | 177 | ||
Membranes Define Boundaries and Serve as Permeability Barriers | 177 | ||
Membranes Are Sites of Specific Proteins and Therefore of Specific Functions | 177 | ||
Membrane Proteins Regulate the Transport of Solutes | 178 | ||
Membrane Proteins Detect and Transmit Electrical and Chemical Signals | 178 | ||
Membrane Proteins Mediate Cell Adhesion and Cell-to-Cell Communication | 178 | ||
7.2: Models of Membrane Structure: An Experimental Perspective | 179 | ||
Overton and Langmuir: Lipids Are Important Components of Membranes | 179 | ||
Gorter and Grendel: The Basis of Membrane Structure Is a Lipid Bilayer | 179 | ||
Davson and Danielli: Membranes Also Contain Proteins | 180 | ||
Robertson: All Membranes Share a Common Underlying Structure | 180 | ||
Further Research Revealed Major Shortcomings of the Davson–Danielli Model | 180 | ||
Singer and Nicolson: A Membrane Consists of a Mosaic of Proteins in a Fluid Lipid Bilayer | 181 | ||
Unwin and Henderson: Most Membrane Proteins Contain Transmembrane Segments | 181 | ||
Recent Findings Suggest Membranes Are Organized into Microdomains | 182 | ||
7.3: Membrane Lipids: The “Fluid” Part of the Model | 183 | ||
Membranes Contain Several Major Classes of Lipids | 183 | ||
Fatty Acids Are Essential to Membrane Structure and Function | 185 | ||
Thin-Layer Chromatography Is an Important Technique for Lipid Analysis | 185 | ||
Membrane Asymmetry: Most Lipids Are Distributed Unequally Between the Two Monolayers | 186 | ||
The Lipid Bilayer Is Fluid | 187 | ||
Membranes Function Properly Only in the Fluid State | 187 | ||
Most Organisms Can Regulate Membrane Fluidity | 190 | ||
Lipid Rafts Are Localized Regions of Membrane Lipids That Are Involved in Cell Signaling | 191 | ||
7.4: Membrane Proteins: The “Mosaic” Part of the Model | 192 | ||
The Membrane Consists of a Mosaic of Proteins: Evidence from Freeze-Fracture Microscopy | 192 | ||
Membranes Contain Integral, Peripheral, and Lipid-Anchored Proteins | 192 | ||
Membrane Proteins Can Be Isolated and Analyzed | 195 | ||
Determining the Three-Dimensional Structure of Membrane Proteins Is Becoming Easier | 198 | ||
Molecular Biology Has Contributed Greatly to Our Understanding of Membrane Proteins | 199 | ||
Membrane Proteins Have a Variety of Functions | 199 | ||
Membrane Proteins Are Oriented Asymmetrically Across the Lipid Bilayer | 200 | ||
Many Membrane Proteins and Lipids Are Glycosylated | 200 | ||
Membrane Proteins Vary in Their Mobility | 202 | ||
The Erythrocyte Membrane Contains an Interconnected Network of Membrane-Associated Proteins | 204 | ||
Summary of Key Points | 205 | ||
Problem Set | 206 | ||
Key Technique: SDS–Polyacrylamide Gel Electrophoresis (SDS-PAGE) of Membrane Proteins | 196 | ||
Chapter 8: Transport Across Membranes: Overcoming the Permeability Barrier | 209 | ||
8.1: Cells and Transport Processes | 210 | ||
Solutes Cross Membranes by Simple Diffusion, Facilitated Diffusion, and Active Transport | 210 | ||
The Movement of a Solute Across a Membrane Is Determined by Its Concentration Gradient or Its Electrochemical Potential | 210 | ||
The Erythrocyte Plasma Membrane Provides Examples of Transport Mechanisms | 212 | ||
8.2: Simple Diffusion: Unassisted Movement Down the Gradient | 212 | ||
Diffusion Always Moves Solutes Toward Equilibrium | 213 | ||
Osmosis Is the Diffusion of Water Across a Selectively Permeable Membrane | 213 | ||
Simple Diffusion Is Typically Limited to Small, Nonpolar Molecules | 215 | ||
The Rate of Simple Diffusion Is Directly Proportional to the Concentration Gradient | 216 | ||
8.3: Facilitated Diffusion: Protein-Mediated Movement Down the Gradient | 216 | ||
Carrier Proteins and Channel Proteins Facilitate Diffusion by Different Mechanisms | 217 | ||
Carrier Proteins Alternate Between Two Conformational States | 217 | ||
Carrier Proteins Are Analogous to Enzymes in Their Specificity and Kinetics | 217 | ||
Carrier Proteins Transport Either One or Two Solutes | 218 | ||
The Erythrocyte Glucose Transporter and Anion Exchange Protein Are Examples of Carrier Proteins | 218 | ||
Channel Proteins Facilitate Diffusion by Forming Hydrophilic Transmembrane Channels | 221 | ||
8.4: Active Transport: Protein-Mediated Movement Up the Gradient | 225 | ||
The Coupling of Active Transport to an Energy Source May Be Direct or Indirect | 225 | ||
Direct Active Transport Depends on Four Types of Transport ATPases | 226 | ||
Indirect Active Transport Is Driven by Ion Gradients | 228 | ||
8.5: Examples of Active Transport | 229 | ||
Direct Active Transport: The Na+/K+ Pump Maintains Electrochemical Ion Gradients | 229 | ||
Indirect Active Transport: Sodium Symport Drives the Uptake of Glucose | 230 | ||
The Bacteriorhodopsin Proton Pump Uses Light Energy to Transport Protons | 232 | ||
8.6: The Energetics of Transport | 233 | ||
For Uncharged Solutes, the ∆G of Transport Depends Only on the Concentration Gradient | 233 | ||
For Charged Solutes, the ∆G of TransportDepends on the Electrochemical Potential | 234 | ||
Summary of Key Points | 235 | ||
Problem Set | 236 | ||
Key Technique: Expression of Heterologous Membrane Proteins in Frog Oocytes | 220 | ||
Human Connections: Membrane Transport, Cystic Fibrosis, and the Prospects for Gene Therapy | 222 | ||
Chapter 9: Chemotrophic Energy Metabolism: Glycolysis and Fermentation | 239 | ||
9.1: Metabolic Pathways | 240 | ||
9.2: ATP: The Primary Energy Molecule in Cells | 240 | ||
ATP Contains Two Energy-Rich Phosphoanhydride Bonds | 240 | ||
ATP Hydrolysis Is Highly Exergonic Due to Several Factors | 241 | ||
ATP Is Extremely Important in Cellular Energy Metabolism | 242 | ||
9.3: Chemotrophic Energy Metabolism | 244 | ||
Biological Oxidations Usually Involve the Removal of Both Electrons and Protons and Are Highly Exergonic | 244 | ||
Coenzymes Such as NAD+ Serve as Electron Acceptors in Biological Oxidations | 245 | ||
Most Chemotrophs Meet Their Energy Needs by Oxidizing Organic Food Molecules | 245 | ||
Glucose Is One of the Most Important Oxidizable Substrates in Energy Metabolism | 246 | ||
The Oxidation of Glucose Is Highly Exergonic | 246 | ||
Glucose Catabolism Yields Much More Energy in the Presence of Oxygen Than in Its Absence | 246 | ||
Based on Their Need for Oxygen, Organisms Are Aerobic, Anaerobic, or Facultative | 246 | ||
9.4: Glycolysis: ATP Generation Without the Involvement of Oxygen | 247 | ||
Glycolysis Generates ATP by Catabolizing Glucose to Pyruvate | 247 | ||
9.5: Fermentation | 250 | ||
In the Absence of Oxygen, Pyruvate Undergoes Fermentation to Regenerate NAD | 250 | ||
Fermentation Taps Only a Fraction of the Substrate’s Free Energy but Conserves That Energy Efficiently as ATP | 252 | ||
Cancer Cells Ferment Glucose to Lactate Even in the Presence of Oxygen | 252 | ||
9.6: Alternative Substrates for Glycolysis | 253 | ||
Other Sugars and Glycerol Are Also Catabolized by the Glycolytic Pathway | 253 | ||
Polysaccharides Are Cleaved to Form Sugar Phosphates That Also Enter the Glycolytic Pathway | 254 | ||
9.7: Gluconeogenesis | 255 | ||
9.8: The Regulation of Glycolysis and Gluconeogenesis | 259 | ||
Key Enzymes in the Glycolytic and Gluconeogenic Pathways Are Subject to Allosteric Regulation | 259 | ||
Fructose-2,6-Bisphosphate Is an Important Regulator of Glycolysis and Gluconeogenesis | 260 | ||
9.9: Novel Roles for Glycolytic Enzymes | 261 | ||
Summary of Key Points | 262 | ||
Problem Set | 263 | ||
Key Technique: Using Isotopic Labeling to Determine the Fate of Atoms in a Metabolic Pathway | 256 | ||
Human Connections: What Happens to the Sugar? | 258 | ||
Chapter 10: Chemotrophic Energy Metabolism: Aerobic Respiration | 266 | ||
10.1: Cellular Respiration: Maximizing ATP Yields | 267 | ||
Aerobic Respiration Yields Much More Energy than Fermentation Does | 267 | ||
Respiration Includes Glycolysis, Pyruvate Oxidation, the Citric Acid Cycle, Electron Transport, and ATP Synthesis | 267 | ||
10.2: The Mitochondrion: Where the Action Takes Place | 267 | ||
Mitochondria Are Often Present Where the ATP Needs Are Greatest | 269 | ||
Are Mitochondria Interconnected Networks Rather than Discrete Organelles? | 269 | ||
The Outer and Inner Membranes Define Two Separate Compartments and Three Regions | 270 | ||
Mitochondrial Functions Occur in or on Specific Membranes and Compartments | 271 | ||
In Bacteria, Respiratory Functions Are Localized to the Plasma Membrane and the Cytoplasm | 273 | ||
10.3: The Citric Acid Cycle: Oxidation in the Round | 274 | ||
Pyruvate Is Converted to Acetyl Coenzyme A by Oxidative Decarboxylation | 275 | ||
The Citric Acid Cycle Begins with the Entry of Acetate as Acetyl CoA | 275 | ||
Two Oxidative Decarboxylations Then Form NADH and Release CO2 | 276 | ||
Direct Generation of GTP (or ATP) Occurs at One Step in the Citric Acid Cycle | 276 | ||
The Final Oxidative Reactions of the Citric Acid Cycle Generate FADH2 and NADH | 276 | ||
Summing Up: The Products of the Citric Acid Cycle Are CO2, ATP, NADH, and FADH2 | 278 | ||
Several Citric Acid Cycle Enzymes Are Subject to Allosteric Regulation | 279 | ||
The Citric Acid Cycle Also Plays a Central Role in the Catabolism of Fats and Proteins | 279 | ||
The Citric Acid Cycle Serves as a Source of Precursors for Anabolic Pathways | 282 | ||
The Glyoxylate Cycle Converts Acetyl CoA to Carbohydrates | 282 | ||
10.4: Electron Transport: Electron Flow from Coenzymes to Oxygen | 284 | ||
The Electron Transport System Conveys Electrons from Reduced Coenzymes to Oxygen | 284 | ||
The Electron Transport System Consists of Five Kinds of Carriers | 285 | ||
The Electron Carriers Function in a Sequence Determined by Their Reduction Potentials | 286 | ||
Most of the Carriers Are Organized into Four Large Respiratory Complexes | 288 | ||
The Respiratory Complexes Move Freely Within the Inner Membrane | 290 | ||
10.5: The Electrochemical Proton Gradient: Key to Energy Coupling | 292 | ||
Electron Transport and ATP Synthesis Are Coupled Events | 292 | ||
Coenzyme Oxidation Pumps Enough Protons to Form Three ATP Molecules per NADH and Two ATP Molecules per FADH2 | 293 | ||
The Chemiosmotic Model Is Affirmed by an Impressive Array of Evidence | 293 | ||
10.6: ATP Synthesis: Putting It All Together | 295 | ||
F1 Particles Have ATP Synthase Activity | 295 | ||
Proton Translocation Through Fo Drives ATP Synthesis by F1 | 297 | ||
ATP Synthesis by FoF1 Involves Physical Rotation of the Gamma Subunit | 298 | ||
The Chemiosmotic Model Involves Dynamic Transmembrane Proton Traffic | 300 | ||
10.7: Aerobic Respiration: Summing It All Up | 301 | ||
The Maximum ATP Yield of Aerobic Respiration Is 38 ATP Molecules per Glucose | 302 | ||
Aerobic Respiration Is a Highly Efficient Process | 303 | ||
Summary of Key Points | 303 | ||
Problem Set | 304 | ||
Key Technique: Visualizing Cellular Structures with Three-Dimensional Electron Microscopy | 272 | ||
Human Connections A Diet Worth Dying For? | 296 | ||
Chapter 11: Phototrophic Energy Metabolism: Photosynthesis | 307 | ||
11.1: An Overview of Photosynthesis | 308 | ||
The Energy Transduction Reactions Convert Solar Energy to Chemical Energy | 308 | ||
The Carbon Assimilation Reactions Fix Carbon by Reducing Carbon Dioxide | 308 | ||
The Chloroplast Is the Photosynthetic Organelle in Eukaryotic Cells | 310 | ||
Chloroplasts Are Composed of Three Membrane Systems | 310 | ||
11.2: Photosynthetic Energy Transduction I: Light Harvesting | 313 | ||
Chlorophyll Is Life’s Primary Link to Sunlight | 313 | ||
Accessory Pigments Further Expand Access to Solar Energy | 314 | ||
Light-Gathering Molecules Are Organized into Photosystems and Light-Harvesting Complexes | 315 | ||
Oxygenic Phototrophs Have Two Types of Photosystems | 316 | ||
11.3: Photosynthetic Energy Transduction II: NADPH Synthesis | 316 | ||
Photosystem II Transfers Electrons from Water to a Plastoquinone | 316 | ||
The Cytochrome b6/f Complex Transfers Electrons from a Plastoquinol to Plastocyanin | 319 | ||
Photosystem I Transfers Electrons from Plastocyanin to Ferredoxin | 319 | ||
Ferredoxin-NADP+ Reductase Catalyzes the Reduction of NADP | 319 | ||
11.4: Photosynthetic Energy Transduction III: ATP Synthesis | 322 | ||
The ATP Synthase Complex Couples Transport of Protons Across the Thylakoid Membrane to ATP Synthesis | 322 | ||
Cyclic Photophosphorylation Allows a Photosynthetic Cell to Balance NADPH and ATP Synthesis | 322 | ||
A Summary of the Complete Energy Transduction System | 323 | ||
Bacteria Use a Photosynthetic Reaction Center and Electron Transport System Similar to Those in Plants | 323 | ||
11.5: Photosynthetic Carbon Assimilation I: The Calvin Cycle | 324 | ||
Carbon Dioxide Enters the Calvin Cycle by Carboxylation of Ribulose-1,5-Bisphosphate | 325 | ||
3-Phosphoglycerate Is Reduced to Form Glyceraldehyde-3-Phosphate | 325 | ||
Regeneration of Ribulose-1,5-Bisphosphate Allows Continuous Carbon Assimilation | 327 | ||
The Complete Calvin Cycle and Its Relation to Photosynthetic Energy Transduction | 327 | ||
11.6: Regulation of the Calvin Cycle | 328 | ||
The Calvin Cycle Is Highly Regulated to Ensure Maximum Efficiency | 328 | ||
Rubisco Activase Regulates Carbon Fixation by Rubisco | 328 | ||
11.7: Photosynthetic Carbon Assimilation II: Carbohydrate Synthesis | 329 | ||
Glucose-1-Phosphate Is Synthesized from Triose Phosphates | 329 | ||
The Biosynthesis of Sucrose Occurs in the Cytosol | 330 | ||
The Biosynthesis of Starch Occurs in the Chloroplast Stroma | 330 | ||
Photosynthesis Also Produces Reduced Nitrogen and Sulfur Compounds | 330 | ||
11.8: Rubisco’s Oxygenase Activity Decreases Photosynthetic Efficiency | 331 | ||
The Glycolate Pathway Returns Reduced Carbon from Phosphoglycolate to the Calvin Cycle | 331 | ||
C4 Plants Minimize Photorespiration by Confining Rubisco to Cells Containing High Concentrations of CO2 | 333 | ||
CAM Plants Minimize Photorespiration and Water Loss by Opening Their Stomata Only at Night | 335 | ||
Summary of Key Points | 336 | ||
Problem Set | 337 | ||
Key Technique: Determining Absorption and Action Spectra via Spectrophotometry | 312 | ||
Human Connections: How do Plants Put on Sunscreen? | 320 | ||
Chapter 12: The Endomembrane System | 338 | ||
12.1: The Endoplasmic Reticulum | 339 | ||
The Two Basic Kinds of Endoplasmic Reticulum Differ in Structure and Function | 340 | ||
Rough ER Is Involved in the Biosynthesis and Processing of Proteins | 340 | ||
Smooth ER Is Involved in Drug Detoxification, Carbohydrate Metabolism, Calcium Storage, and Steroid Biosynthesis | 341 | ||
The ER Plays a Central Role in the Biosynthesis of Membranes | 343 | ||
12.2: The Golgi Apparatus | 343 | ||
The Golgi Apparatus Consists of a Series of Membrane-Bounded Cisternae | 343 | ||
Two Models Account for the Flow of Lipids and Proteins Through the Golgi Apparatus | 344 | ||
12.3: Roles of the ER and Golgi Apparatus in Protein Glycosylation | 345 | ||
Initial Glycosylation Occurs in the ER | 345 | ||
Further Glycosylation Occurs in the Golgi Apparatus | 346 | ||
12.4: Roles of the ER and Golgi Apparatus in Protein Trafficking | 348 | ||
ER-Specific Proteins Contain Retention and Retrieval Tags | 348 | ||
Golgi Apparatus Proteins May Be Sorted According to the Lengths of Their Membrane-Spanning Domains | 349 | ||
Targeting of Soluble Lysosomal Proteins to Endosomes and Lysosomes Is a Model for Protein Sorting in the TGN | 349 | ||
12.5: Exocytosis and Endocytosis: Transporting Material Across the Plasma Membrane | 351 | ||
Secretory Pathways Transport Molecules to the Exterior of the Cell | 351 | ||
Exocytosis Releases Intracellular Molecules Outside the Cell | 352 | ||
Endocytosis Imports Extracellular Molecules by Forming Vesicles from the Plasma Membrane | 353 | ||
12.6: Coated Vesicles in Cellular Transport Processes | 360 | ||
Clathrin-Coated Vesicles Are Surrounded by Lattices Composed of Clathrin and Adaptor Protein | 360 | ||
The Assembly of Clathrin Coats Drives the Formation of Vesicles from the Plasma Membrane and TGN | 361 | ||
COPI- and COPII-Coated Vesicles Travel Between the ER and Golgi Apparatus Cisternae | 362 | ||
SNARE Proteins Mediate Fusion Between Vesicles and Target Membranes | 362 | ||
12.7: Lysosomes and Cellular Digestion | 364 | ||
Lysosomes Isolate Digestive Enzymes from the Rest of the Cell | 364 | ||
Lysosomes Develop from Endosomes | 364 | ||
Lysosomal Enzymes Are Important for Several Different Digestive Processes | 365 | ||
Lysosomal Storage Diseases Are Usually Characterized by the Accumulation of Indigestible Material | 367 | ||
12.8: The Plant Vacuole: A Multifunctional Organelle | 368 | ||
12.9: Peroxisomes | 368 | ||
Most Peroxisomal Functions Are Linked to Hydrogen Peroxide Metabolism | 369 | ||
Plant Cells Contain Types of Peroxisomes Not Found in Animal Cells | 370 | ||
Peroxisome Biogenesis Can Occur by Division of Preexisting Peroxisomes or by Vesicle Fusion | 370 | ||
Summary of Key Points | 371 | ||
Problem Set | 372 | ||
Key Technique: Visualizing Vesicles at the Cell Surface Using Total Internal Reflection (TIRF) Microscopy | 354 | ||
Human Connections: It’s All in the Family | 357 | ||
Chapter 13: Cytoskeletal Systems | 375 | ||
13.1: Major Structural Elements of the Cytoskeleton | 376 | ||
Eukaryotes Have Three Basic Types of Cytoskeletal Elements | 376 | ||
Bacteria Have Cytoskeletal Systems That Are Structurally Similar to Those in Eukaryotes | 376 | ||
The Cytoskeleton Is Dynamically Assembled and Disassembled | 376 | ||
13.2: Microtubules | 378 | ||
Two Types of Microtubules Are Responsible for Many Functions in the Cell | 378 | ||
Tubulin Heterodimers Are the Protein Building Blocks of Microtubules | 378 | ||
Microtubules Can Form as Singlets, Doublets, or Triplets | 379 | ||
Microtubules Form by the Addition of Tubulin Dimers at Their Ends | 379 | ||
Addition of Tubulin Dimers Occurs More Quickly at the Plus Ends of Microtubules | 381 | ||
Drugs Can Affect the Assembly and Stability of Microtubules | 381 | ||
GTP Hydrolysis Contributes to the Dynamic Instability of Microtubules | 382 | ||
Microtubules Originate from Microtubule-Organizing Centers Within the Cell | 383 | ||
MTOCs Organize and Polarize Microtubules Within Cells | 384 | ||
Microtubule Stability Is Tightly Regulated in Cells by a Variety of Microtubule-Binding Proteins | 386 | ||
13.3: Microfilaments | 388 | ||
Actin Is the Protein Building Block of Microfilaments | 389 | ||
Different Types of Actin Are Found in Cells | 389 | ||
G-Actin Monomers Polymerize into F-Actin Microfilaments | 389 | ||
Specific Drugs Affect Polymerization of Microfilaments | 390 | ||
Cells Can Dynamically Assemble Actin into a Variety of Structures | 390 | ||
Actin-Binding Proteins Regulate the Polymerization, Length, and Organization of Microfilaments | 390 | ||
Proteins That Link Actin to Membranes | 393 | ||
Phospholipids and Rho Family GTPases Regulate Where and When Actin-Based Structures Assemble | 394 | ||
13.4: Intermediate Filaments | 396 | ||
Intermediate Filament Proteins Are Tissue Specific | 397 | ||
Intermediate Filaments Assemble from Fibrous Subunits | 398 | ||
Intermediate Filaments Confer Mechanical Strength on Tissues | 398 | ||
The Cytoskeleton Is a Mechanically Integrated Structure | 399 | ||
Summary of Key Points | 399 | ||
Problem Set | 400 | ||
Key Technique: Studying the Dynamic Cytoskeleton | 386 | ||
Human Connections: When Actin Kills | 395 | ||
Chapter 14: Cellular Movement: Motility and Contractility | 401 | ||
14.1: Microtubule-Based Movement Inside Cells: Kinesins and Dyneins | 403 | ||
Motor Proteins Move Cargoes Along MTs During Axonal Transport | 403 | ||
Classic Kinesins Move Toward the Plus Ends of Microtubules | 404 | ||
Kinesins Are a Large Family of Proteins | 404 | ||
Dyneins Are Found in Axonemes and the Cytosol | 404 | ||
Microtubule Motors Direct Vesicle Transport and Shape the Endomembrane System | 408 | ||
14.2: Microtubule-Based Cell Motility: Cilia and Flagella | 408 | ||
Cilia and Flagella Are Common Motile Appendages of Eukaryotic Cells | 408 | ||
Cilia and Flagella Consist of an Axoneme Connected to a Basal Body | 409 | ||
Doublet Sliding Within the Axoneme Causes Cilia and Flagella to Bend | 410 | ||
14.3: Microfilament-Based Movement Inside Cells: Myosins | 411 | ||
Myosins Are a Large Family of Actin-Based Motors with Diverse Roles in Cell Motility | 411 | ||
Many Myosins Move Along Actin Filaments in Short Steps | 413 | ||
14.4: Microfilament-Based Motility: Muscle Cells in Action | 413 | ||
Skeletal Muscle Cells Contain Thin and Thick Filaments | 413 | ||
Sarcomeres Contain Ordered Arrays of Actin, Myosin, and Accessory Proteins | 414 | ||
The Sliding-Filament Model Explains Muscle Contraction | 416 | ||
Cross-Bridges Hold Filaments Together, and ATP Powers Their Movement | 418 | ||
The Regulation of Muscle Contraction Depends on Calcium | 418 | ||
The Coordinated Contraction of Cardiac Muscle Cells Involves Electrical Coupling | 421 | ||
Smooth Muscle Is More Similar to Nonmuscle Cells than to Skeletal Muscle | 422 | ||
14.5: Microfilament-Based Motility in Nonmuscle Cells | 423 | ||
Cell Migration via Lamellipodia Involves Cycles of Protrusion, Attachment,Translocation, and Detachment | 423 | ||
Chemotaxis Is a Directional Movement in Response to a Graded Chemical Stimulus | 425 | ||
Amoeboid Movement Involves Cycles of Gelation and Solation of Actin | 425 | ||
Actin-Based Motors Move Components Within the Cytosol of Some Cells | 426 | ||
Summary of Key Points | 426 | ||
Problem Set | 427 | ||
Key Technique: Watching Motors Too Small to See | 406 | ||
Human Connections: Dyneins Help Us Tell Left from Right | 412 | ||
Chapter 15: Beyond the Cell: Cell Adhesions, Cell Junctions, and Extracellular Structures | 429 | ||
15.1: Cell-Cell Junctions | 430 | ||
Adhesive Junctions Link Adjoining Cells | 430 | ||
Transient Cell-Cell Adhesions Are Importantf or Many Cellular Events | 433 | ||
Tight Junctions Prevent the Movement of Molecules Across Cell Layers | 434 | ||
Gap Junctions Allow Direct Electrical and Chemical Communication Between Cells | 438 | ||
15.2: The Extracellular Matrix of Animal Cells | 439 | ||
Collagens Are Responsible for the Strength of the Extracellular Matrix | 439 | ||
Elastins Impart Elasticity and Flexibility to the Extracellular Matrix | 440 | ||
Collagen and Elastin Fibers Are Embedded in a Matrix of Proteoglycans | 441 | ||
Free Hyaluronate Lubricates Joints and Facilitates Cell Migration | 443 | ||
Adhesive Glycoproteins Anchor Cells to the Extracellular Matrix | 443 | ||
Fibronectins Bind Cells to the ECM and Guide Cellular Movement | 443 | ||
Laminins Bind Cells to the Basal Lamina | 444 | ||
Integrins Are Cell Surface Receptors That Bind ECM Components | 444 | ||
The Dystrophin/Dystroglycan Complex Stabilizes Attachments of Muscle Cells to the ECM | 448 | ||
15.3: The Plant Cell Surface | 449 | ||
Cell Walls Provide a Structural Framework and Serve as a Permeability Barrier | 449 | ||
The Plant Cell Wall Is a Network of Cellulose Microfibrils, Polysaccharides, and Glycoproteins | 449 | ||
Cell Walls Are Synthesized in Several Discrete Stages | 450 | ||
Plasmodesmata Permit Direct Cell-Cell Communication Through the Cell Wall | 451 | ||
Summary of Key Points | 452 | ||
Problem Set | 453 | ||
Human Connections: The Costly Effects of Weak Adhesion | 435 | ||
Key Technique: Building an ECM from Scratch | 446 | ||
Chapter 16: The Structural Basis of Cellular Information: DNA, Chromosomes, and the Nucleus | 455 | ||
16.1: Chemical Nature of the Genetic Material | 456 | ||
The Discovery of DNA Led to Conflicting Proposals Concerning the Chemical Nature of Genes | 456 | ||
Avery Showed That DNA Is the Genetic Material of Bacteria | 457 | ||
Hershey and Chase Showed That DNA Is the Genetic Material of Viruses | 457 | ||
RNA Is the Genetic Material in Some Viruses | 459 | ||
16.2: DNA Structure | 461 | ||
Chargaff’s Rules Reveal That A = T and G = C | 461 | ||
Watson and Crick Discovered That DNA Is a Double Helix | 462 | ||
DNA Can Be Interconverted Between Relaxed and Supercoiled Forms | 464 | ||
The Two Strands of a DNA Double Helix Can Be Denatured and Renatured | 466 | ||
16.3: DNA Packaging | 467 | ||
Bacteria Package DNA in Bacterial Chromosomes and Plasmids | 467 | ||
Eukaryotes Package DNA in Chromatin and Chromosomes | 470 | ||
Nucleosomes Are the Basic Unit of Chromatin Structure | 470 | ||
A Histone Octamer Forms the Nucleosome Core | 471 | ||
Nucleosomes Are Packed Together to Form Chromatin Fibers and Chromosomes | 471 | ||
Changes in Histones and Chromatin Remodeling Proteins Can Alter Chromatin Packing | 473 | ||
Chromosomal DNA Contains Euchromatin and Heterochromatin | 474 | ||
Some Heterochromatin Plays a Structural Role in Chromosomes | 474 | ||
Chromosomes Can Be Identified by Unique Banding Patterns | 474 | ||
Eukaryotic Chromosomes Contain Large Amounts of Repeated DNA Sequences | 475 | ||
Eukaryotes Package Some of Their DNA in Mitochondria and Chloroplasts | 477 | ||
16.4: The Nucleus | 478 | ||
A Double-Membrane Nuclear Envelope Surrounds the Nucleus | 478 | ||
Molecules Enter and Exit the Nucleus Through Nuclear Pores | 480 | ||
The Nucleus Is Mechanically Integrated with the Rest of the Cell | 483 | ||
Chromatin Is Located Within the Nucleus in a Nonrandom Fashion | 484 | ||
The Nucleolus Is Involved in Ribosome Formation | 484 | ||
Summary of Key Points | 486 | ||
Problem Set | 486 | ||
Key Technique: FISHing for Specific Sequences | 468 | ||
Human Connections: Lamins and Premature Aging | 485 | ||
Chapter 17: DNA Replication, Repair, and Recombination | 488 | ||
17.1: DNA Replication | 489 | ||
DNA Synthesis Occurs During S Phase | 489 | ||
DNA Replication Is Semiconservative | 489 | ||
DNA Replication Is Usually Bidirectional | 491 | ||
Replication Initiates at Specialized DNA Elements | 494 | ||
DNA Polymerases Catalyze the Elongation of DNA Chains | 495 | ||
DNA Is Synthesized as Discontinuous Segments That Are Joined Together by DNA Ligase | 498 | ||
In Bacteria, Proofreading Is Performed bythe 3‘ -> 5‘ Exonuclease Activity of DNA Polymerase | 499 | ||
RNA Primers Initiate DNA Replication | 500 | ||
The DNA Double Helix Must Be Locally Unwound During Replication | 502 | ||
DNA Unwinding and DNA Synthesis Are Coordinated on Both Strands Via the Replisome | 503 | ||
Eukaryotes Disassemble and Reassemble Nucleosomes as Replication Proceeds | 503 | ||
Telomeres Solve the DNA End-Replication Problem | 505 | ||
17.2: DNA Damage and Repair | 508 | ||
Mutations Can Occur Spontaneously During Replication | 508 | ||
Mutagens Can Induce Mutations | 510 | ||
DNA Repair Systems Correct Many Kinds of DNA Damage | 512 | ||
17.3: Homologous Recombination and Mobile Genetic Elements | 517 | ||
Homologous Recombination Is Initiated by Double-Stranded Breaks in DNA | 517 | ||
Transposons Are Mobile Genetic Elements | 519 | ||
Transposons Differ Based on Their Autonomy and Mechanism of Movement | 519 | ||
Bacterial Transposons Can Be Composite or Non-composite | 520 | ||
Eukaryotes Also Have Transposons | 521 | ||
Summary of Key Points | 521 | ||
Problem Set | 521 | ||
Key Technique: The Polymerase Chain Reaction (PCR) | 500 | ||
Human Connections: Children of the Moon | 514 | ||
Chapter 18: Gene Expression: I. The Genetic Code and Transcription | 523 | ||
18.1: The Genetic Code and the Directional Flow of Genetic Information | 524 | ||
Transcription and Translation Involve Many of the Same Components in Prokaryotes and Eukaryotes | 524 | ||
Where Transcription and Translation Occur Differs in Prokaryotes and Eukaryotes | 524 | ||
In Some Cases RNA Is Reversed Transcribed into DNA | 526 | ||
The Genetic Code | 527 | ||
The Genetic Code Is a Triplet Code | 529 | ||
The Genetic Code Is Degenerate and Nonoverlapping | 530 | ||
Messenger RNA Guides the Synthesis of Polypeptide Chains | 531 | ||
The Codon Dictionary Was Established Using Synthetic RNA Polymers and Triplets | 532 | ||
Of the 64 Possible Codons in Messenger RNA, 61 Encode Amino Acids | 532 | ||
The Genetic Code Is (Nearly) Universal | 533 | ||
18.2: Mechanisms of Transcription | 534 | ||
Transcription Involves Four Stages: RNA Polymerase Binding, Initiation, Elongation, and Termination | 534 | ||
Bacterial Transcription Involves σ Factor Binding, Initiation, Elongation, andTermination | 534 | ||
Transcription in Eukaryotic Cells Has Additional Complexity Compared with Prokaryotes | 540 | ||
RNA Polymerases I, II, and III Carry Out Transcription in the Eukaryotic Nucleus | 540 | ||
Three Classes of Promoters Are Found in Eukaryotic Nuclear Genes, One for Each Type of RNA Polymerase | 541 | ||
General Transcription Factors Are Involved in the Transcription of All Nuclear Genes | 543 | ||
Elongation, Termination, and RNA Cleavage Are Involved in Completing Eukaryotic RNA Synthesis | 544 | ||
18.3: RNA Processing and Turnover | 545 | ||
The Nucleolus Is Involved in Ribosome Formation | 545 | ||
Ribosomal RNA Processing Involves Cleavage of Multiple rRNAs from a Common Precursor | 546 | ||
Transfer RNA Processing Involves Removal, Addition, and Chemical Modification of Nucleotides | 548 | ||
Messenger RNA Processing in Eukaryotes Involves Capping, Addition of Poly(A), and Removal of Introns | 549 | ||
Spliceosomes Remove Introns from Pre-mRNA | 551 | ||
Some Introns Are Self-Splicing | 552 | ||
The Existence of Introns Permits Alternative Splicing and Exon Shuffling | 553 | ||
Cells Localize Nuclear RNAs in Several Types of Processing Centers | 554 | ||
Nucleic Acid Editing Allows Sequences to Be Altered | 554 | ||
The C-Terminal Domain of RNA Polymerase II Coordinates RNA Processing | 555 | ||
Most mRNA Molecules Have a Relatively Short Life Span | 555 | ||
The Abundance of mRNA Allows Amplification of Genetic Information | 555 | ||
Summary of Key Points | 556 | ||
Problem Set | 556 | ||
Key Technique: Hunting for DNA-Protein Interactions | 536 | ||
Chapter 19: Gene Expression: II. Protein Synthesis and Sorting | 559 | ||
19.1: Translation: The Cast of Characters | 560 | ||
Ribosomes Carry Out Polypeptide Synthesis | 560 | ||
Transfer RNA Molecules Bring Amino Acids to the Ribosome | 561 | ||
Aminoacyl-tRNA Synthetases Link Amino Acids to the Correct Transfer RNAs | 564 | ||
Messenger RNA Brings Polypeptide Coding Information to the Ribosome | 565 | ||
Protein Factors Are Required for Translational Initiation, Elongation, and Termination | 566 | ||
19.2: The Mechanism of Translation | 566 | ||
Translational Initiation Requires Initiation Factors, Ribosomal Subunits, mRNA, and Initiator tRNA | 566 | ||
Chain Elongation Involves Cycles of Aminoacyl tRNA Binding, Peptide Bond Formation, and Translocation | 570 | ||
Most mRNAs Are Read by Many Ribosomes Simultaneously | 572 | ||
Termination of Polypeptide Synthesis Is Triggered by Release Factors That Recognize Stop Codons | 572 | ||
Polypeptide Folding Is Facilitated by Molecular Chaperones | 572 | ||
Protein Synthesis Typically Utilizes a Substantial Fraction of a Cell’s Energy Budget | 574 | ||
A Summary of Translation | 575 | ||
19.3: Mutations and Translation | 575 | ||
Suppressor tRNAs Overcome the Effects of Some Mutations | 576 | ||
Nonsense-Mediated Decay and Nonstop Decay Promote the Destruction of Defective mRNAs | 577 | ||
19.4: Posttranslational Processing | 577 | ||
19.5: Protein Targeting and Sorting | 580 | ||
Cotranslational Import Allows Some Polypeptides to Enter the ER as They Are Being Synthesized | 580 | ||
The Signal Recognition Particle (SRP) Attachesthe Ribosome-mRNA-Polypeptide Complex to the ER Membrane | 584 | ||
Protein Folding and Quality Control Take Place Within the ER | 585 | ||
Proteins Released into the ER Lumen Are Routed to the Golgi Apparatus, Secretory Vesicles, Lysosomes, or Back to the ER | 585 | ||
Stop-Transfer Sequences Mediate the Insertion of Integral Membrane Proteins | 586 | ||
Posttranslational Import Is an Alternative Mechanism for Import into the ER Lumen | 586 | ||
Posttranslational Import Across Two Membranes Allows Some Polypeptides to Enter Mitochondria and Chloroplasts | 588 | ||
Summary of Key Points | 590 | ||
Problem Set | 591 | ||
Key Technique: Protein Localization Using Fluorescent Fusion Proteins | 578 | ||
Human Connections: To Catch a Killer: The Problem of Antibiotic Resistance in Bacteria | 583 | ||
Chapter 20: The Regulation of Gene Expression | 593 | ||
20.1: Bacterial Gene Regulation | 594 | ||
Catabolic and Anabolic Pathways Are Regulated Through Induction and Repression, Respectively | 594 | ||
The Genes Involved in Lactose Catabolism Are Organized into an Inducible Operon | 595 | ||
The lac Operon Is Negatively Regulated by the lac Repressor | 595 | ||
Studies of Mutant Bacteria Revealed How the lac Operon Is Organized | 597 | ||
Catabolite Activator Protein (CAP) Positively Regulates the lac Operon | 599 | ||
The lac Operon Is an Example of the Dual Control of Gene Expression | 599 | ||
The Structure of the lac Repressor/Operator Complex Confirms the Operon Model | 600 | ||
The Genes Involved in Tryptophan Synthesis Are Organized into a Repressible Operon | 600 | ||
Sigma Factors Determine Which Sets of Genes Can Be Expressed | 600 | ||
Attenuation Allows Transcription to Be Regulated After the Initiation Step | 601 | ||
Riboswitches Allow Transcription and Translation to Be Controlled by Small-Molecule Interactions with RNA | 603 | ||
The CRISPR/Cas System Protects Bacteria Against Viral Infection | 604 | ||
20.2: Eukaryotic Gene Regulation: Genomic Control | 605 | ||
Multicellular Eukaryotes Are Composed of Numerous Specialized Cell Types | 605 | ||
Eukaryotic Gene Expression Is Regulated at Five Main Levels | 605 | ||
The Cells of a Multicellular Organism Usually Contain the Same Set of Genes | 606 | ||
Gene Amplification and Deletion Can Alter the Genome | 608 | ||
DNA Rearrangements Can Alter the Genome | 609 | ||
Chromatin Decondensation Is Involved in Genomic Control | 610 | ||
DNA Methylation Is Associated with Inactive Regions of the Genome | 611 | ||
20.3: Eukaryotic Gene Regulation: Transcriptional Control | 615 | ||
Different Sets of Genes Are Transcribed in Different Cell Types | 615 | ||
Proximal Control Elements Lie Close to the Promoter | 616 | ||
Enhancers and Silencers Are DNA Elements Located at Variable Distances from the Promoter | 617 | ||
Coactivators Mediate the Interaction Between Regulatory Transcription Factors and the RNA Polymerase Complex | 619 | ||
Multiple DNA Control Elements and Transcription Factors Act in Combination | 620 | ||
DNA-Binding and Activation Domains of Regulatory Transcription Factors Are Functionally Separable | 620 | ||
Several Common Types of Transcription Factors Bind to DNA and Activate Transcription | 621 | ||
DNA Response Elements Coordinate the Expression of Nonadjacent Genes | 623 | ||
Steroid Hormone Receptors Act as Transcription Factors That Bind to Hormone Response Elements | 623 | ||
CREBs and STATs Are Examples of Transcription Factors Activated by Phosphorylation | 624 | ||
The Heat-Shock Response Element Coordinates Stress Responses | 625 | ||
Homeotic Genes Encode Transcription Factors That Regulate Embryonic Development | 625 | ||
20.4: Eukaryotic Gene Regulation: Posttranscriptional Control | 627 | ||
Control of RNA Processing and Nuclear Export Follows Transcription | 627 | ||
Translation Rates Can Be Controlled by Initiation Factors and Translational Repressors | 627 | ||
Translation Can Also Be Controlled by Regulation of mRNA Degradation | 629 | ||
RNA Interference Utilizes Small RNAs to Silence Gene Expression | 630 | ||
MicroRNAs Produced by Normal Cellular Genes Silence the Translation of mRNAs | 631 | ||
Piwi-interacting RNAs Are Small Regulatory RNAs That Protect the Germline of Eukaryotes | 634 | ||
Long Noncoding RNAs Play a Variety of Roles in Eukaryotic Gene Regulation | 634 | ||
Posttranslational Control Involves Modifications of Protein Structure, Function, and Degradation | 634 | ||
Ubiquitin Targets Proteins for Degradation by Proteasomes | 635 | ||
A Summary of Eukaryotic Gene Regulation | 636 | ||
Summary of Key Points | 637 | ||
Problem Set | 638 | ||
Human Connections: The Epigenome: Methylation and Disease | 613 | ||
Key Technique: Gene Knockdown via RNAi | 632 | ||
Chapter 21: Molecular Biology Techniques for Cell Biology | 640 | ||
21.1: Analyzing and Manipulating DNA | 640 | ||
Gel Electrophoresis Allows DNA to Be Separated by Size | 641 | ||
Restriction Endonucleases Cleave DNA Molecules at Specific Sites | 642 | ||
Restriction Mapping Can Characterize DNA | 643 | ||
Restriction Endonucleases Can Identify Methylated DNA | 644 | ||
Southern Blotting Identifies Specific DNAs from a Mixture | 644 | ||
Restriction Enzymes Allow Production of Recombinant DNA | 645 | ||
PCR Is Widely Used to Clone Genes | 646 | ||
Genomic and cDNA Libraries Are Both Useful for DNA Cloning | 646 | ||
Rapid Procedures Exist for DNA Sequencing | 651 | ||
21.2: Analyzing Genomes | 654 | ||
Whole Genomes Can Be Sequenced | 654 | ||
Comparative Genomics Allows Comparison of Genomes and Genes Within Them | 656 | ||
The Field of Bioinformatics Helps to Decipher Genomes | 657 | ||
Tiny Differences in Genome Sequence Distinguish People from One Another | 660 | ||
21.3: Analyzing RNA and Proteins | 663 | ||
Several Techniques Allow Detection of mRNAs in Time and Space | 663 | ||
The Transcription of Thousands of Genes Can Be Assessed Simultaneously | 664 | ||
Proteins Can Be Studied Using Electrophoresis | 665 | ||
Antibodies Can Be Used to Study Specific Proteins | 665 | ||
Proteins Can Be Isolated by Size, Charge, or Affinity | 667 | ||
Proteins Can Be Identified from Complex Mixtures Using Mass Spectrometry | 668 | ||
Protein Function Can Be Studied Using Molecular Biology Techniques | 669 | ||
Protein-Protein Interactions Can Be Studied in a Variety of Ways | 670 | ||
21.4: Analyzing and Manipulating Gene Function | 672 | ||
Transgenic Organisms Carry Foreign Genes That Are Passed On to Subsequent Generations | 672 | ||
Transcriptional Reporters Are Useful for Studying Regulation of Gene Expression | 674 | ||
The Role of Specific Genes Can Be Assessed By Identifying Mutations and by Knockdown | 674 | ||
Genetic Engineering Can Produce Valuable Proteins That Are Otherwise Difficult to Obtain | 677 | ||
Food Crops Can Be Genetically Modified | 678 | ||
Gene Therapies Are Being Developed for the Treatment of Human Diseases | 678 | ||
Summary of Key Points | 679 | ||
Problem Set | 680 | ||
Key Technique: DNA Cloning | 648 | ||
Human Connections: More Than Your Fingertips: Identifying Genetic “Fingerprints” | 662 | ||
Chapter 22: Signal Transduction Mechanisms: I. Electrical and Synaptic Signaling in Neurons | 682 | ||
22.1: Neurons and Membrane Potential | 683 | ||
Neurons Are Specially Adapted for the Transmission of Electrical Signals | 683 | ||
Neurons Undergo Changes in Membrane Potential | 684 | ||
Resting Membrane Potential Depends on Ion Concentrations and Selective Membrane Permeability | 685 | ||
The Nernst Equation Describes the Relationship Between Membrane Potential and Ion Concentration | 686 | ||
Steady-State Ion Concentrations Affect Resting Membrane Potential | 686 | ||
The Goldman Equation Describes the Combined Effects of Ions on Membrane Potential | 687 | ||
22.2: Electrical Excitability and the Action Potential | 688 | ||
Patch Clamping and Molecular Biological Techniques Allow Study of Single Ion Channels | 688 | ||
Specific Domains of Voltage-Gated Channels Act as Sensors and Inactivators | 688 | ||
Action Potentials Propagate Electrical Signals Along an Axon | 692 | ||
Action Potentials Involve Rapid Changes in the Membrane Potential of the Axon | 692 | ||
Action Potentials Result from the Rapid Movement of Ions Through Axonal Membrane Channels | 692 | ||
Action Potentials Are Propagated Along the Axon Without Losing Strength | 694 | ||
The Myelin Sheath Acts Like an Electrical Insulator Surrounding the Axon | 695 | ||
22.3: Synaptic Transmission and Signal Integration | 697 | ||
Neurotransmitters Relay Signals Across Nerve Synapses | 697 | ||
Elevated Calcium Levels Stimulate Secretion of Neurotransmitters from Presynaptic Neurons | 700 | ||
Secretion of Neurotransmitters Involves the Docking and Fusion of Vesicles with the Plasma Membrane | 701 | ||
Neurotransmitters Are Detected by Specific Receptors on Postsynaptic Neurons | 703 | ||
Neurotransmitters Must Be Inactivated Shortly After Their Release | 704 | ||
Postsynaptic Potentials Integrate Signals from Multiple Neurons | 704 | ||
Summary of Key Points | 705 | ||
Problem Set | 706 | ||
Key Technique: Patch Clamping | 690 | ||
Human Connections: In the Search for the Fountain of Youth, Are People Paying for Poison? | 702 | ||
Chapter 23: Signal Transduction Mechanisms: II. Messengers and Receptors | 708 | ||
23.1: Chemical Signals and Cellular Receptors | 709 | ||
Chemical Signaling Involves Several Key Components | 709 | ||
Receptor Binding Involves Quantitative Interactions Between Ligands and Their Receptors | 710 | ||
Cells Can Amplify Signals Once They Are Received | 711 | ||
Cell-Cell Signals Act Through a Limited Number of Receptors and Signal Transduction Pathways | 711 | ||
23.2: G Protein–Coupled Receptors | 713 | ||
G Protein–Coupled Receptors Act Via Hydrolysis of GTP | 713 | ||
Cyclic AMP Is a Second Messenger Whose Production Is Regulated by Some G Proteins | 715 | ||
Disruption of G Protein Signaling Causes Human Disease | 716 | ||
Many G Proteins Act Through Inositol Trisphosphate and Diacylglycerol | 717 | ||
The Release of Calcium Ions Is a Key Event in Many Signaling Processes | 718 | ||
23.3: Protein Kinase-Associated Receptors | 722 | ||
Growth Factors Often Bind Protein Kinase-Associated Receptors | 723 | ||
Receptor Tyrosine Kinases Aggregate and Undergo Autophosphorylation | 723 | ||
Receptor Tyrosine Kinases Initiate a Signal Transduction Cascade Involving Ras and MAP Kinase | 724 | ||
The Key Steps in RTK Signaling Can Be Dissected Using Mutants | 725 | ||
Receptor Tyrosine Kinases Activate a Variety of Other Signaling Pathways | 727 | ||
Other Growth Factors Transduce Their Signals via Receptor Serine-Threonine Kinases | 727 | ||
23.4: Putting It All Together: Signal Integration | 728 | ||
Scaffolding Complexes Can Facilitate Cell Signaling | 729 | ||
Different Signaling Pathways Are Integrated Through Crosstalk | 730 | ||
23.5: Hormones and Other Long-Range Signals | 731 | ||
Hormones Can Be Classified by Their Chemical Properties | 731 | ||
The Endocrine System Controls Multiple Signaling Pathways to Regulate Glucose Levels | 731 | ||
Steroid Hormones Bind Hormones in the Cytosol and Carry Them into the Nucleus | 733 | ||
Gases Can Act as Cell Signals | 734 | ||
Summary of Key Points | 736 | ||
Problem Set | 737 | ||
Key Technique: Calcium Indicators and Ionophore | 721 | ||
Human Connections: The Gas That Prevents a Heart Attack | 735 | ||
Chapter 24: The Cell Cycle and Mitosis | 738 | ||
24.1: Overview of the Cell Cycle | 739 | ||
24.2: Nuclear and Cell Division | 743 | ||
Mitosis Is Subdivided into Prophase, Prometaphase, Metaphase, Anaphase, and Telophase | 743 | ||
The Mitotic Spindle Is Responsible for Chromosome Movements During Mitosis | 746 | ||
Cytokinesis Divides the Cytoplasm | 749 | ||
Bacteria and Eukaryotic Organelles Divide in a Different Manner from Eukaryotic Cells | 752 | ||
24.3: Regulation of the Cell Cycle | 753 | ||
Cell Cycle Length Varies Among Different Cell Types | 753 | ||
Cell Cycle Progression Is Controlled at Several Key Transition Points | 754 | ||
Cell Fusion Experiments and Cell Cycle Mutants Identified Molecules That Control the Cell Cycle | 755 | ||
Progression Through the Cell Cycle Is Controlled by Cyclin-Dependent Kinases (Cdks) | 755 | ||
Cdk-Cyclin Complexes Are Regulated | 756 | ||
The Anaphase-Promoting Complex Allows Exit from Mitosis | 758 | ||
Checkpoint Pathways Monitor Key Steps in the Cell Cycle | 758 | ||
Putting It All Together: The Cell Cycle Regulation Machine | 761 | ||
24.4: Growth Factors and Cell Proliferation | 762 | ||
Stimulatory Growth Factors Activate the Ras Pathway | 762 | ||
Stimulatory Growth Factors Can Also Activate the PI 3-Kinase–Akt Pathway | 763 | ||
Inhibitory Growth Factors Act Through Cdk Inhibitors | 764 | ||
24.5: Apoptosis | 764 | ||
Apoptosis Is Triggered by Death Signals or Withdrawal of Survival Factors | 765 | ||
Summary of Key Points | 767 | ||
Problem Set | 768 | ||
Key Technique: Measuring Cells Millions at a Time | 740 | ||
Human Connections: What Do Ethnobotany and Cancer Have in Common? | 750 | ||
Chapter 25: Sexual Reproduction, Meiosis, and Genetic Recombination | 770 | ||
25.1: Sexual Reproduction | 771 | ||
Sexual Reproduction Produces Genetic Variety | 771 | ||
Gametes Are Haploid Cells Specialized for Sexual Reproduction | 771 | ||
25.2: Meiosis | 771 | ||
The Life Cycles of Sexual Organisms Have Diploid and Haploid Phases | 772 | ||
Meiosis Converts One Diploid Cell into Four Haploid Cells | 772 | ||
Meiosis I Produces Two Haploid Cells That Have Chromosomes Composed of Sister Chromatids | 773 | ||
Meiosis II Resembles a Mitotic Division | 778 | ||
Defects in Meiosis Lead to Nondisjunction | 779 | ||
Sperm and Egg Cells Are Generated by Meiosis Accompanied by Cell Differentiation | 781 | ||
Meiotic Maturation of Oocytes is Tightly Regulated | 782 | ||
25.3: Genetic Variability: Segregation and Assortment of Alleles | 784 | ||
Meiosis Generates Genetic Diversity | 784 | ||
Information Specifying Recessive Traits Can Be Present Without Being Displayed | 784 | ||
Alleles of Each Gene Segregate from Each Other During Gamete Formation | 785 | ||
Alleles of Each Gene Segregate Independently of the Alleles of Other Genes | 786 | ||
Chromosome Behavior Explains the Laws of Segregation and Independent Assortment | 787 | ||
The DNA Molecules of Homologous Chromosomes Have Similar Base Sequences | 787 | ||
25.4: Genetic Variability: Recombination and Crossing Over | 789 | ||
Chromosomes Contain Groups of Linked Genes That Are Usually Inherited Together | 789 | ||
Homologous Chromosomes Exchange Segments During Crossing Over | 789 | ||
Gene Locations Can Be Mapped by Measuring Recombination Frequencies | 791 | ||
25.5: Genetic Recombination in Bacteria and Viruses | 792 | ||
Co-infection of Bacterial Cells with Related Bacteriophages Can Lead to Genetic Recombination | 792 | ||
Recombination in Bacteria Can Occur Via Transformation or Transduction | 793 | ||
Conjugation Is a Modified Sexual Activity That Facilitates Genetic Recombination in Bacteria | 793 | ||
25.6: Mechanisms of Homologous Recombination | 796 | ||
DNA Breakage and Exchange Underlie Homologous Recombination Between Chromosomes | 796 | ||
The Synaptonemal Complex Facilitates Homologous Recombination During Meiosis | 796 | ||
Homologous Recombination Between Chromosomes Relies on High-Fidelity DNA Repair | 797 | ||
Summary of Key Points | 799 | ||
Problem Set | 799 | ||
Human Connections: When Meiosis Goes Awry | 780 | ||
Key Technique: Using Mendel’s Rules to Predict Human Disease | 790 | ||
Chapter 26: Cancer Cells | 802 | ||
26.1: How Cancers Arise | 802 | ||
Tumors Arise When the Balance Between Cell Division and Cell Differentiation or Death Is Disrupted | 803 | ||
Cancer Cell Proliferation Is Anchorage Independent and Insensitive to Population Density | 804 | ||
Cancer Cells Are Immortalized by Mechanisms That Maintain Telomere Length | 804 | ||
Defects in Signaling Pathways, Cell Cycle Controls, and Apoptosis Contribute to Cancer | 804 | ||
Cancer Arises Through a Multistep Process Involving Initiation, Promotion, and Tumor Progression | 805 | ||
26.2: How Cancers Spread | 806 | ||
Angiogenesis Is Required for Tumors to Grow Beyond a Few Millimeters in Diameter | 806 | ||
Blood Vessel Growth Is Controlled by a Balance Between Angiogenesis Activators and Inhibitors | 807 | ||
Cancer Cells Spread by Invasion and Metastasis | 808 | ||
Changes in Cell Adhesion, Motility, and Protease Production Promote Metastasis | 808 | ||
Relatively Few Cancer Cells Survive the Voyage Through the Bloodstream | 809 | ||
Blood Flow and Organ-Specific Factors Determine Sites of Metastasis | 809 | ||
The Immune System Influences the Growth and Spread of Cancer Cells | 810 | ||
The Tumor Microenvironment Influences Tumor Growth, Invasion, and Metastasis | 810 | ||
26.3: What Causes Cancer? | 811 | ||
Epidemiological Data Have Allowed Many Causes of Cancer to Be Identified | 811 | ||
Many Chemicals Can Cause Cancer, Often After Metabolic Activation in the Liver | 812 | ||
DNA Mutations Triggered by Chemical Carcinogens Lead to Cancer | 812 | ||
Ionizing and Ultraviolet Radiation Also Cause DNA Mutations That Lead to Cancer | 813 | ||
Viruses and Other Infectious Agents Trigger the Development of Some Cancers | 814 | ||
26.4: Oncogenes and Tumor Suppressor Genes | 815 | ||
Oncogenes Are Genes Whose Products Can Trigger the Development of Cancer | 815 | ||
Proto-oncogenes Are Converted into Oncogenes by Several Distinct Mechanisms | 815 | ||
Most Oncogenes Encode Components of Growth-Signaling Pathways | 817 | ||
Tumor Suppressor Genes Are Genes Whose Loss or Inactivation Can Lead to Cancer | 820 | ||
The RB Tumor Suppressor Gene Was Discovered by Studying Families with Hereditary Retinoblastoma | 821 | ||
The p53 Tumor Suppressor Gene Is the Most Frequently Mutated Gene in Human Cancers | 822 | ||
The APC Tumor Suppressor Gene Encodes a Protein That Inhibits the Wnt Signaling Pathway | 823 | ||
Inactivation of Some Tumor Suppressor Genes Leads to Genetic Instability | 824 | ||
Cancers Develop by the Stepwise Accumulation of Mutations Involving Oncogenes and Tumor Suppressor Genes | 825 | ||
Epigenetic Changes in Gene Expression Influence the Properties of Cancer Cells | 828 | ||
Summing Up: Carcinogenesis and the Hallmarks of Cancer | 828 | ||
26.5: Diagnosis, Screening, and Treatment | 829 | ||
Cancer Is Diagnosed by Microscopic and Molecular Examination of Tissue Specimens | 830 | ||
Screening Techniques for Early Detection Can Prevent Cancer Deaths | 830 | ||
Surgery, Radiation, and Chemotherapy Are Standard Treatments for Cancer | 831 | ||
Using the Immune System to Target Cancer Cells | 831 | ||
Molecular Targeting Can Attack Cancer Cells More Specifically Than Chemotherapy | 834 | ||
Cancer Treatments Can Be Tailored to Individual Patients | 834 | ||
Summary of Key Points | 834 | ||
Problem Set | 835 | ||
Key Technique: Targeting Molecules in the Fight Against Cancer | 832 | ||
Human Connections: Molecular Sleuthing in Cancer Diagnosis | 826 | ||
Appendix: Visualizing Cells and Molecules | 837 | ||
Optical Principles of Microscopy | 837 | ||
The Illuminating Wavelength Sets a Limit on How Small an Object Can Be Seen | 837 | ||
Resolution Refers to the Ability to Distinguish Adjacent Objects as Separate from One Another | 838 | ||
The Practical Limit of Resolution Is Roughly 200 nm for Standard Light Microscopes and 2 nm for Electron Microscopes | 840 | ||
The Light Microscope | 840 | ||
Compound Microscopes Use Several Lenses in Combination | 840 | ||
Phase-Contrast Microscopy Detects Differences in Refractive Index and Thickness | 841 | ||
Differential Interference Contrast (DIC) Microscopy Utilizes a Split Light Beam to Detect Phase Differences | 842 | ||
Digital Microscopy Can Enhance Captured Images | 843 | ||
Fluorescence Microscopy Can Detect the Presence of Specific Molecules or Ions Within Cells | 844 | ||
Confocal Microscopy Minimizes Blurring by Excluding Out-of-Focus Light from an Image | 846 | ||
Other Techniques Minimize Blurring by Exciting a Thin Strip of Fluorescent Molecules | 848 | ||
Digital Deconvolution Microscopy Can Be Used to Generate Sharp Three-Dimensional Images After Acquisition | 849 | ||
Optical Methods Can Be Used to Measure and Manipulate Macromolecules | 850 | ||
Superresolution Microscopy Has Broken the Diffraction Limit | 851 | ||
Specimen Preparation Often Involves Fixation, Sectioning, and Staining | 852 | ||
The Electron Microscope | 852 | ||
Transmission Electron Microscopy Forms an Image from Electrons That Pass Through the Specimen | 852 | ||
Scanning Electron Microscopy Reveals the Surface Architecture of Cells and Organelles | 854 | ||
Sample Preparation Techniques for Electron Microscopy | 854 | ||
Ultrathin Sectioning and Staining Are Common Techniques in Transmission Electron Microscopy | 854 | ||
Radioisotopes and Antibodies Can Localize Molecules in Electron Micrographs | 856 | ||
Negative Staining Can Highlight Small Objects in Relief Against a Stained Background | 856 | ||
Shadowing Techniques Use Metal Vapor Sprayed Across a Specimen’s Surface | 856 | ||
Freeze Fracturing and Freeze Etching Are Useful for Examining Membranes | 857 | ||
Stereo Electron Microscopy and 3-D Electron Tomography Allow Specimens to Be Viewed in Three Dimensions | 858 | ||
Specimen Preparation for Scanning Electron Microscopy Involves Fixation but Not Sectioning | 859 | ||
Other Imaging Methods | 860 | ||
Scanning Probe Microscopy Reveals the Surface Features of Individual Molecules | 860 | ||
CryoEM Bridges the Gap Between X-Ray Crystallography and Electron Microscopy | 861 | ||
Answer Key to Concept Check and Key Technique Box Questions | 862 | ||
Glossary | 869 | ||
Photo, Illustration, and Text Credits | 895 | ||
Index | 901 | ||
Back Cover | Back Cover |