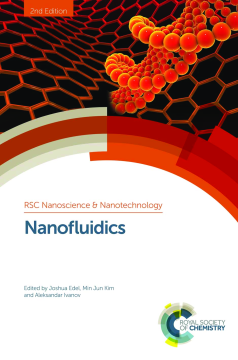
Additional Information
Book Details
Abstract
There has been significant growth in the field of nanofluidics, where nanoscale analytical instruments employ micromachined features and are able to manipulate fluid samples with high precision and efficiency and have many advantages over their conventional (larger) analogues.
The new edition of Nanofluidics has been fully revised and updated with the latest advancements and applications. With a focus on bioanalysis, specific applications are given with case studies. The end of each chapter now also features a methodology section to explain experimental protocols and “tips and tricks”.
The editors draw on an international authorship and provide a handbook for the community. Written at an accessible level the book is suitable for both experts and non-experts alike.
Recommended for the reader wanting to get a rapid look into a number of active research areas in nanofluidics.
Aaron Timperman
Table of Contents
Section Title | Page | Action | Price |
---|---|---|---|
Cover | Cover | ||
Preface | vii | ||
About the Editors | x | ||
Contents | xiii | ||
Chapter 1 Transport of Ions, DNA Polymers, and Microtubules in the Nanofluidic Regime | 1 | ||
1.1 Introduction | 1 | ||
1.2 Ionic Transport | 2 | ||
1.2.1 Electrically Driven Ion Transport | 3 | ||
1.2.2 Streaming Currents | 6 | ||
1.2.3 Streaming Currents as a Probe of Charge Inversion | 8 | ||
1.2.4 Electrokinetic Energy Conversion in Nanofluidic Channels | 10 | ||
1.3 Polymer Transport | 12 | ||
1.3.1 Pressure-driven Polymer Transport | 13 | ||
1.3.2 Electrokinetic DNA Concentration in Nanofluidic Channels | 17 | ||
1.3.3 DNA Conformations and Dynamics in Slit-like Nanochannels | 19 | ||
1.4 Microtubule Transport in Nanofluidic Channels Driven by Electric Fields and by Kinesin Biomolecular Motors | 20 | ||
1.4.1 Electrical Manipulation of Kinesin-driven Microtubule Transport | 21 | ||
1.4.2 Mechanical Properties of Microtubules Measured from Electric Field-induced Bending | 25 | ||
1.4.3 Electrophoresis of Individual Microtubules in Microfluidic Channels | 28 | ||
Acknowledgments | 31 | ||
References | 32 | ||
Chapter 2 Electrokinetic Transport and Fluidic Manipulation in Three Dimensional Integrated Nanofluidic Networks | 37 | ||
2.1 Introduction | 37 | ||
2.2 Experimental Characterization of Nanofluidic Flow | 40 | ||
2.2.1 Surface Charge | 40 | ||
2.2.2 Debye Length | 42 | ||
2.3 Integrated Nanofluidic Systems | 44 | ||
2.3.1 Molecular Sampling (Digital Fluidic Manipulation) | 44 | ||
2.3.2 Pre-concentration | 47 | ||
2.3.3 Chemical Reactivity | 50 | ||
2.4 Theory and Simulations | 53 | ||
2.4.1 Theory | 53 | ||
2.4.2 Ion Accumulation and Depletion | 55 | ||
2.4.3 Ionic Currents | 58 | ||
2.4.4 Induced Flow | 59 | ||
2.4.5 Nanofluidic Diode | 66 | ||
2.4.6 Reaction Kinetics | 68 | ||
2.5 Conclusions | 71 | ||
Acknowledgments | 71 | ||
References | 72 | ||
Chapter 3 Nanopillars, Nanowires and Nanoballs for DNA and Protein Analysis | 76 | ||
3.1 Introduction | 76 | ||
3.2 Fabrication of Nanopillars, Nanowires, and Nanoballs | 77 | ||
3.2.1 Fabrication of Nanopillars | 77 | ||
3.2.2 Fabrication of Nanowires | 78 | ||
3.2.3 Self-assembled Nanospheres | 78 | ||
3.2.4 Synthesis of PEGylated-latex | 81 | ||
3.3 Nanopillars for DNA and Protein Analysis | 82 | ||
3.3.1 DNA Analysis by Tilted Patterned Nanopillar Chips | 82 | ||
3.3.2 Single DNA Molecule Imaging in Tilted Pattern Nanopillar Chips | 84 | ||
3.3.3 DNA Analysis by Square Patterned Nanopillar Chips and Nanowall Chips | 85 | ||
3.3.4 Single DNA Molecule Imaging in Square Patterned Nanopillar Chips | 86 | ||
3.3.5 Protein Analysis by Tilted Patterned Nanopillar Chips | 86 | ||
3.4 Nanowalls for DNA Analysis | 86 | ||
3.4.1 DNA Analysis by Nanowall Chips | 86 | ||
3.4.2 Single DNA Molecule Imaging in Nanowall Chips | 87 | ||
3.4.3 Mechanism of Separation in Nanopillar Chips and Nanowall Chips | 88 | ||
3.5 Nanowires for DNA and Protein Analysis | 89 | ||
3.5.1 DNA Analysis by Nanowire Chips | 89 | ||
3.5.2 Single DNA Molecule Imaging in Nanowire Chips | 91 | ||
3.5.3 Separation Mechanism in Nanowire Chips | 91 | ||
3.5.4 Protein Analysis by Nanowire Chips | 92 | ||
3.6 Nanoballs for DNA Analysis | 92 | ||
3.6.1 DNA Analysis by a Self-assembled Nanosphere Solution in a Chip | 92 | ||
3.6.2 DNA Analysis by PEGylated-latex Mixed Polymer Solution in a Chip | 93 | ||
3.6.3 Single DNA Molecule Imaging in a Nanoball Solution | 94 | ||
3.7 Conclusion | 96 | ||
Acknowledgments | 96 | ||
References | 97 | ||
Chapter 4 Nanofluidic Devices for Electroanalytical Applications | 99 | ||
4.1 Introduction | 99 | ||
4.2 Nanofluidic Devices for Single Molecule Electrochemical Detection | 102 | ||
4.3 Nanofluidic Devices for Selective Electrochemical Detection | 108 | ||
4.4 Nanofluidic Devices for Probing Electron Transfer Kinetics | 110 | ||
4.5 Conclusions | 111 | ||
References | 111 | ||
Chapter 5 Nanofluidic Strategies for Cancer Research | 114 | ||
5.1 Introduction | 114 | ||
5.2 Fabrication of Nanofluidic Platforms | 116 | ||
5.2.1 Concepts | 116 | ||
5.2.2 Top-down Nanofluidic Platform Fabrication | 118 | ||
5.2.3 Nanofluidic Platform Fabrication | 118 | ||
5.2.4 Heat-induced Stretching Method | 130 | ||
5.3 Analysis of Single Molecules Using Nanofluidic Tubes | 132 | ||
5.3.1 Experimental Setup | 132 | ||
5.3.2 Detection and Measurement of Single Molecules in Nanofluidic Channels | 135 | ||
5.3.3 Electrokinetic Molecule Transport in Nanofluidic Tubing | 135 | ||
5.4 Cancer Research Application | 139 | ||
5.4.1 Determination of the Detection of the MAX Concentration Using a Nanochannel Device | 139 | ||
5.4.2 Epithelial Growth Factor Receptor Ubiquitination Detection by Microchannels with Two Fluorescent Color Detection System | 139 | ||
5.4.3 Electrokinetic C-3. EGFR Phosphorylation Detection by Microchannels with Three Fluorescent Color Detection System | 142 | ||
5.5 Conclusions | 144 | ||
Acknowledgments | 145 | ||
References | 145 | ||
Chapter 6 Nanofluidics for Biomolecular Detection | 150 | ||
6.1 Introduction to Nanopore-based Genome Sequencing | 150 | ||
6.1.1 The Basic Idea: From Coulter Counter to Sequencer | 150 | ||
6.1.2 Sequencing via Tunnelling Conductance | 152 | ||
6.1.3 Challenges: Regulating Molecule Motion in Nanofluidics | 154 | ||
6.2 Electrical Gating of Nanopore System | 155 | ||
6.2.1 Electroosmotic Flow and DNA Motion | 157 | ||
6.2.2 Poisson-Navier-Nernst Description of Nanofluidic System | 161 | ||
6.2.3 Gate Manipulating: DNA Translocating Stage | 164 | ||
6.2.4 Gate Manipulating: DNA Capture Stage | 166 | ||
6.2.5 Experiments: Gating Nanopore | 169 | ||
6.3 Salt-gradient Driving DNA Motion | 171 | ||
6.3.1 DNA Capture Under Salt Gradient | 172 | ||
6.3.2 DNA Translocation Tuned by Salt Gradient | 174 | ||
6.4 Temperature-gradient for DNA Propelling | 178 | ||
6.4.1 Temperature Distribution in Nanofluidics | 180 | ||
6.4.2 Temperature-gradient Driven DNA Capture | 183 | ||
6.4.3 Temperature-gradient Driven DNA Translocation | 184 | ||
References | 187 | ||
Chapter 7 Silicon Nitride Thin Films for Nanofluidic Device Fabrication | 190 | ||
7.1 Introduction | 190 | ||
7.1.1 Formation of LPCVD Silicon Nitride Films | 191 | ||
7.1.2 Formation of Free-standing LPCVD Silicon Nitride Films | 192 | ||
7.1.3 Overview of Selected Free-standing Silicon Nitride Membrane Structural Motifs and Applications | 193 | ||
7.2 Nanofluidic Applications of Thin Silicon Nitride Membranes | 194 | ||
7.2.1 10 Picoleagues Under the Sea: Nanofluidics for Transmission Electron Microscopy (TEM) of Liquid Samples | 195 | ||
7.2.2 Portal to the Molecular World: Nanopore Single-molecule Sensing | 200 | ||
7.3 Silicon-rich Silicon Nitride Surface Chemistry | 203 | ||
7.3.1 Real-world Silicon Nitride Surface Chemistry | 204 | ||
7.3.2 Hydrosilylation of Silicon-rich Silicon Nitride | 208 | ||
7.4 Fabrication of Channels in Silicon Nitride Nanofluidic Devices | 210 | ||
7.4.1 Windowed Nanochannels | 210 | ||
7.4.2 Nanopore Formation and Fabrication | 213 | ||
7.5 Peering into the Void: Characterising Nanopores Using Conductance | 218 | ||
7.6 Nanofluidic Vistas | 227 | ||
Acknowledgments | 228 | ||
References | 228 | ||
Chapter 8 Single Molecule Protein Unfolding Using a Nanopore | 237 | ||
8.1 Introduction | 237 | ||
8.1.1 Nanopores as a Unique Molecular Probe | 238 | ||
8.2 Nanopore Geometry and Fabrication | 240 | ||
8.3 Protein Adsorption Kinetics | 244 | ||
8.3.1 The PDZ2 Protein Domain | 244 | ||
8.3.2 PDZ2-Nanopore Interactions | 245 | ||
8.3.3 Voltage Pulses for Controlling Nanopore Clogging | 247 | ||
8.4 Chemical and Electric Field Unfolding: Competing Effects | 249 | ||
8.5 Simulating Protein Folding in a Nanopore | 257 | ||
8.6 Detecting Single Point Mutations and Stability Variations | 259 | ||
8.6.1 Translocation Event Statistics | 259 | ||
8.6.2 Excluded Volumes and Stability Measurements | 262 | ||
8.7 Outlook | 265 | ||
References | 266 | ||
Chapter 9 Low Noise Nanopore Platforms Optimised for the Synchronised Optical and Electrical Detection of Biomolecules | 270 | ||
9.1 Introduction | 270 | ||
9.2 Hybrid Nanopore-Zero-mode Waveguide Platforms: A Brief History | 272 | ||
9.3 Designing a Hybrid Nanopore-Zero-mode Waveguide | 274 | ||
9.3.1 Choice of Pore Diameter | 274 | ||
9.3.2 Choice of Membrane Materials | 276 | ||
9.4 A Novel Low-noise Platform | 279 | ||
9.4.1 Fabrication Protocol | 280 | ||
9.4.2 Laboratory Set-up | 282 | ||
9.4.3 Sources of Ionic Current Noise | 284 | ||
9.4.4 Device Performance | 286 | ||
9.5 Synchronizing Optical and Electrical Detection Measurements | 292 | ||
9.5.1 Independent Electrical and Optical Detection of dsDNA | 292 | ||
9.5.2 Synchronized Optical and Electrical Detection of dsDNA | 293 | ||
9.5.3 Future Work: Device Optimisation and Applications | 296 | ||
9.6 Conclusion | 296 | ||
Acknowledgments | 297 | ||
References | 297 | ||
Subject Index | 301 |