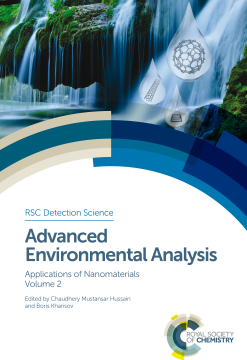
Additional Information
Book Details
Abstract
Environmental analysis techniques have advanced due to the use of nanotechnologies in improving the detection sensitivity and miniaturization of the devices in analytical procedures. These allow for developments such as increases in analyte concentration, the removal of interfering species and improvements in the detection limits. Bridging a gap in the literature, this book uniquely brings together state-of-the-art research in the applications of novel nanomaterials to each of the classical components of environmental analysis, namely sample preparation and extraction, separation and identification by spectroscopic techniques. Special attention is paid to those approaches that are considered greener and reduce the cost of the analysis process both in terms of chemicals and time consumption.
Advanced undergraduates, graduates and researchers at the forefront of environmental science and engineering will find this book a good source of information. It will also help regulators, decision makers, surveillance agencies and the organizations assessing the impact of pollutants on the environment.
Table of Contents
Section Title | Page | Action | Price |
---|---|---|---|
Cover | Cover | ||
Advanced Environmental Analysis Applications of Nanomaterials, Volume 2 | i | ||
Preface | v | ||
Contents | vii | ||
Section V - New Trends in Environmental Analysis (Magnetic NMs, Nano-Sensors, Nano-Bio Sensors etc.,) | 1 | ||
Chapter 19 - Magnetic Nanomaterials for Environmental Analysis | 3 | ||
19.1 Introduction | 3 | ||
19.2 Properties and Format of Magnetic Nanomaterials | 4 | ||
19.3 Synthesis and Processing of Magnetic NMs | 6 | ||
19.4 Environmental Applications of Magnetic Nanomaterials | 7 | ||
19.5 Conclusions and Perspectives | 10 | ||
Websites of Interest | 12 | ||
References | 12 | ||
Chapter 20 - New Nanoscaled Paramagnetic Complexes (NPCs) Based on Porphyrins/Phthalocyanines for Environmental Chemistry | 14 | ||
20.1 Introduction | 14 | ||
20.2 Synthesis, Characterization and Calculation Techniques for NPCs | 19 | ||
20.2.1 Synthesis, Spectra and Stability of the NPCs | 19 | ||
20.2.2 Microcalorimetric Characterization for NPCs | 21 | ||
20.2.3 Calculation of the Magnetothermal Properties Parameters for NPCs | 21 | ||
20.3 Important Features of New NPCs | 23 | ||
20.3.1 Chemical Structure and Stability in Solutions and Solid | 23 | ||
20.3.2 Magnetothermal Properties of New NPCs | 27 | ||
20.3.2.1 (5,10,15,20-Tetraphenylporphinato)Gadolinium(iii) Acetylacetonate | 27 | ||
20.3.2.2 (Phthalocyaninato)Gadolinium(iii) and (Phthalocyaninato)Thulium(iii) Acetates | 30 | ||
20.3.2.3 (Phthalocyaninato)Gadolinium(iii) Double-Decker Complex | 33 | ||
20.3.2.4 (2,3,7,8,12,13,17,18-Octa-meta-trifluoromethylphenyltetraazaporphinato)manganese(iii) Acetate | 36 | ||
20.4 Success in Using Strategy of SCU–FCU Coupling for NPCs | 40 | ||
20.5 Future Aspects and Conclusion | 42 | ||
Acknowledgements | 43 | ||
References | 43 | ||
Chapter 21 - Nanostructured Metal Oxides for Sensing Toxic Air Pollutants | 48 | ||
21.1 Introduction | 48 | ||
21.2 Semiconductor Gas Sensors | 50 | ||
21.3 Film Deposition Methods | 51 | ||
21.3.1 Chemical Vapour Deposition | 52 | ||
21.3.2 Thermal and Electron Beam Evaporation | 53 | ||
21.3.3 Sputter Deposition | 53 | ||
21.3.4 Pulsed Laser Deposition | 54 | ||
21.3.5 Spray Pyrolysis | 54 | ||
21.3.6 Sol–Gel Process | 54 | ||
21.3.7 Screen Printing | 55 | ||
21.3.8 Alternative Film Deposition Methods | 55 | ||
21.4 Gas Sensing Materials | 55 | ||
21.4.1 Binary Metal Oxides | 57 | ||
21.4.2 Ternary Metal Oxides | 57 | ||
21.4.3 Mixed Metal Oxides | 62 | ||
21.5 Gas Sensing Mechanism | 64 | ||
21.6 Sensitivity and Selectivity | 67 | ||
21.6.1 Film Microstructure | 68 | ||
21.6.2 Film Thickness | 70 | ||
21.6.3 Metal Additives | 71 | ||
21.7 Field Testing | 72 | ||
References | 75 | ||
Chapter 22 - Endotoxin Monitoring Using Nanomaterials | 91 | ||
22.1 Introduction | 91 | ||
22.2 Current Diagnosis Techniques for Endotoxin Monitoring | 92 | ||
22.2.1 Conventional Methods | 93 | ||
22.2.2 Biosensor-Based Endotoxin Monitoring Methods | 94 | ||
22.2.3 LAL-Based Endotoxin Detection Method | 96 | ||
22.3 Nanomaterials for Endotoxin Detection | 96 | ||
22.3.1 Inorganic Nanomaterials | 96 | ||
22.3.2 Organic Nanomaterials | 99 | ||
22.4 Conclusions | 102 | ||
References | 102 | ||
Chapter 23 - Nanozyme-Based Environmental Monitoring | 108 | ||
23.1 Introduction | 108 | ||
23.2 Different Types of Enzyme-Like Characteristics Exhibited By Nanomaterials | 110 | ||
23.2.1 Peroxidase Activity | 110 | ||
23.2.2 Oxidase Activity | 110 | ||
23.2.3 Catalase Activity | 111 | ||
23.2.4 Superoxide Dismutase (SOD) Activity | 111 | ||
23.3 Nanomaterials as Artificial Enzymes (Nanozymes) | 112 | ||
23.3.1 Iron Oxide Nanoparticles | 112 | ||
23.3.1.1 Peroxidase Mimetic Activity of IONPs | 112 | ||
23.3.1.2 Catalase-Like Activity of Iron Oxide Nanoparticles | 113 | ||
23.3.2 Cerium Oxide Nanoparticles | 114 | ||
23.3.2.1 Nanoceria as SOD Mimetic | 114 | ||
23.3.2.2 Nanoceria as Catalase Mimetic | 114 | ||
23.3.2.3 Nanoceria as Oxidase Mimetic | 115 | ||
23.3.2.4 Nanoceria as Peroxidase Mimetic | 116 | ||
23.3.3 Cobalt Oxide Nanoparticles | 116 | ||
23.3.3.1 Cobalt Oxide as Peroxidase Mimetic | 116 | ||
23.3.3.2 Cobalt Oxide as Catalase Mimetic | 117 | ||
23.3.3.3 Cobalt Oxide as Oxidase Mimetic | 117 | ||
23.3.3.4 Cobalt Oxide as SOD Mimetic | 117 | ||
23.3.4 Other Oxide-Based Nanoparticles | 117 | ||
23.3.4.1 Copper Oxide as Peroxidase Mimetic | 117 | ||
23.3.4.2 Vanadium Oxide as Peroxidase Mimetic | 117 | ||
23.3.5 Gold Nanoparticles | 118 | ||
23.3.5.1 Gold Nanoparticles as Peroxidase Mimetic | 118 | ||
23.3.6 Platinum Nanoparticles | 119 | ||
23.3.6.1 Platinum Nanoparticles as Peroxidase Mimetic | 119 | ||
23.3.6.2 Platinum Nanoparticles as Catalase Mimetic | 119 | ||
23.3.6.3 Platinum Nanoparticles as SOD Mimics | 119 | ||
23.3.7 Carbon-Based Nanomaterials | 119 | ||
23.3.7.1 Carbon-Based Nanomaterials as Peroxidase Mimetic | 120 | ||
23.4 Applications Exploiting Enzyme-Like Activity of Nanoparticles for Environmental Monitoring | 120 | ||
23.4.1 Hydrogen Peroxide and Associated Molecule Detection | 120 | ||
23.4.2 Glutathione Detection | 121 | ||
23.4.3 Melamine Detection | 122 | ||
23.4.4 Kanamycin Detection | 122 | ||
23.4.5 Acetamiprid Detection | 124 | ||
23.4.6 Amino Acid Detection | 124 | ||
23.4.7 Sulfite Detection | 124 | ||
23.4.8 Metal Ion Detection | 125 | ||
23.4.9 Degradation of Organic Pollutants | 126 | ||
23.5 Conclusion and Future Perspective | 127 | ||
Acknowledgements | 127 | ||
References | 128 | ||
Chapter 24 - Graphene-Based Gas Sensors | 133 | ||
24.1 Introduction | 133 | ||
24.2 Synthesis and Properties of Graphene | 134 | ||
24.3 Chemiresistive Gas Sensors | 137 | ||
24.4 Sensors Based on Field Effect Transistors | 141 | ||
24.5 Surface Acoustic Wave Sensors | 144 | ||
24.6 Quartz Crystal Microbalance Sensors | 145 | ||
24.7 Other Types of Sensing Devices | 148 | ||
References | 150 | ||
Chapter 25 - Photocatalytic Degradation of Natural and Synthetic Estrogens with Semiconducting Nanoparticles | 153 | ||
25.1 Introduction | 153 | ||
25.2 Methodology | 155 | ||
25.3 Estrogen Photolysis | 156 | ||
25.4 Titanium Dioxide Photocatalysis | 156 | ||
25.4.1 Degradation of Estrogens Using Immobilized TiO2 Photocatalyst | 161 | ||
25.4.2 Photocatalytic Degradation of Estrogens Using Powdered TiO2 Nanoparticles | 162 | ||
25.5 Photocatalytic Efficiency Measurement | 164 | ||
25.6 Mechanism of Estrogen Degradation by Titanium Dioxide | 165 | ||
25.7 Photocatalytic Degradation of Estrogen in Wastewater and Freshwater | 169 | ||
25.8 Enhanced Photocatalytic Processes | 171 | ||
25.9 Conclusion and Perspectives | 173 | ||
References | 173 | ||
Chapter 26 - Smart Composite Materials for Environmental Decontamination | 178 | ||
26.1 Introduction | 178 | ||
26.2 Smart Composites | 179 | ||
26.3 Types of Smart Composites | 179 | ||
26.3.1 Polymer Composites | 180 | ||
26.3.1.1 Polymer-Semiconductor Composites | 181 | ||
26.3.1.2 Polymer–Metal Composites | 183 | ||
26.3.1.3 Polymer–Metal Oxide Composites | 184 | ||
26.3.1.4 Polymer–Carbon Nanotube Composites | 185 | ||
26.3.1.5 Polymer–Graphene Composites | 187 | ||
26.3.2 Metal–Polymer Composites | 188 | ||
26.3.3 CNT or Graphene-Semiconductor Composites | 189 | ||
26.3.4 Other Carbon Allotropes | 190 | ||
26.4 Properties and Behaviours of Smart Composites | 190 | ||
26.5 Applications | 191 | ||
26.5.1 Environmental Decontamination | 191 | ||
26.5.1.1 Adsorption | 191 | ||
26.5.1.2 Photocatalytic Water Treatment | 192 | ||
26.5.1.3 Air Purification | 193 | ||
26.5.1.4 Self-Cleaning Surfaces and Devices | 194 | ||
26.5.1.5 Photocatalytic Disinfection | 194 | ||
26.5.2 Desalination | 194 | ||
26.5.3 Energy Storage and Conversion | 195 | ||
26.5.4 Dye-Sensitized Solar Cells | 195 | ||
26.5.5 Water Splitting | 196 | ||
26.5.6 Sensors | 197 | ||
26.6 Conclusion and Perspectives | 199 | ||
Abbreviations | 199 | ||
References | 200 | ||
Chapter 27 - Nanostructured Oxide Materials for Photodegradation of Dyes | 207 | ||
27.1 Introduction | 207 | ||
27.2 Mechanisms | 209 | ||
27.3 TiO2 in Photocatalysis | 211 | ||
27.3.1 Brookite Phase in Photocatalysis | 213 | ||
27.3.2 Biphase Anatase–Brookite and Rutile–Brookite in Photocatalysis | 215 | ||
27.4 Nanomaterials in Photocatalysis | 216 | ||
27.4.1 Zinc Oxide | 218 | ||
27.4.2 Titanium Dioxide | 219 | ||
27.4.3 WO3 in Photocatalysis | 219 | ||
27.4.4 Copper Oxide in Photocatalysis | 223 | ||
27.5 Major Problems in Photocatalysis Processes | 223 | ||
27.5.1 Effect of pH | 224 | ||
27.5.2 Effect of Light Intensity | 225 | ||
27.5.3 Effect of Initial Concentration | 225 | ||
27.6 Conclusion and Future Challenges | 225 | ||
References | 226 | ||
Section VI Nanomaterials for Water Treatment and Purification | 235 | ||
Chapter 28 - Desalination of Irrigation Water, Livestock Water, and Reject Brine Using n-ZVM (Fe0, Al0, Cu0) | 237 | ||
28.1 Introduction | 237 | ||
28.1.1 Desalination Using n-ZVM | 238 | ||
28.1.1.1 n-ZVM Stabilization | 240 | ||
28.1.1.2 ZVM Particle Sizes Associated with Desalination | 240 | ||
28.1.1.3 Observations Associated with ZVM Desalination | 241 | ||
28.2 Desalination Process | 242 | ||
28.2.1 Terminal Surfaces Responsible for Na and Cl Removal | 243 | ||
28.2.2 Role of Capacitance | 243 | ||
28.2.3 Pourbaix Stability Fields | 244 | ||
28.2.3.1 Green Rust | 244 | ||
28.2.3.2 β-FeOOH | 245 | ||
28.2.4 Assessment of Desalination | 245 | ||
28.2.4.1 Electrical Conductivity | 245 | ||
28.2.4.2 UV-Visible Absorbance | 246 | ||
28.2.4.3 Impact of Water Consumption | 246 | ||
28.3 Desalination of a Batch of Water | 247 | ||
28.3.1 Desalination Rate Constant | 247 | ||
28.3.1.1 Assessment of Rate Constant, kobserved, Variation with Temperature | 247 | ||
28.3.1.2 Assessment of the Rate Constant, kactual | 247 | ||
28.3.1.3 Increasing Particle Surface Area and the Exponential Constant w | 248 | ||
28.3.1.4 Variation in the Rate Constant, kactual, with Temperature | 248 | ||
28.3.1.5 Impact of Freezing Events | 248 | ||
28.3.2 Desalination Producing Potable Water at Ambient Temperatures | 249 | ||
28.3.2.1 Desalination Profile Subdivision | 249 | ||
Negative Activation Energies.Negative activation energies are a characteristic of the outcome of sequential reactions where the ... | 249 | ||
28.3.2.2 Definition of Potable Water Salinity | 250 | ||
28.3.3 Partial Desalination Using N2/N2 + H2 Modified Particles and Pellets | 250 | ||
28.3.3.1 Auto-Reduction | 250 | ||
28.3.4 Partial Desalination in a Reducing Environment | 252 | ||
28.3.4.1 Acceleration of Partial Desalination by Partial Freezing | 253 | ||
28.3.4.2 Assessment of the Reaction Quotient | 253 | ||
28.3.5 Partial Desalination in an Oxidising Environment | 254 | ||
28.3.5.1 Basic Redox Desalination Principles | 255 | ||
28.3.5.2 Assessing the Electrochemical Pseudo Specific Capacitance | 256 | ||
28.3.5.3 Partial Desalination Using COx/N2/H2 Modified Particles and Pellets | 257 | ||
28.3.5.4 Commercial Unit Reactor Structure | 257 | ||
28.3.5.5 Combined Redox and Eutectic Desalination | 258 | ||
Example of Combined Eutectic Desalination with Redox Desalination.An example relationship between water temperature, residual wa... | 259 | ||
28.3.5.6 Rapid Desalination at Elevated Temperatures (25–75 °C) | 260 | ||
28.4 Potential Applications of n-ZVM Desalination | 261 | ||
28.4.1 Irrigation | 261 | ||
28.4.2 Livestock Feed Water | 263 | ||
28.4.3 Impoundments | 264 | ||
28.4.4 Reject Brine from Reverse Osmosis Plants | 265 | ||
28.4.5 Emergency Relief Water | 267 | ||
28.5 Conclusions | 267 | ||
References | 268 | ||
Section VII - Various Important Aspects About Nanomaterials (Toxicity, Economic and Legal) | 273 | ||
Chapter 29 - Nanomaterial Toxicity | 275 | ||
29.1 Definition and Classification of Nanomaterials | 275 | ||
29.2 Nanoparticle Physicochemical Properties-Mediated Toxicity | 281 | ||
29.2.1 Size-Mediated Toxicity | 283 | ||
29.2.2 Composition-Mediated Toxicity | 284 | ||
29.2.3 Crystalline Structure-Mediated Toxicity | 284 | ||
29.2.4 Shape-Mediated Toxicity | 285 | ||
29.2.5 Water Solubility–Dispersability- or Hydrophobicity–Hydrophilicity-Mediated Toxicity | 287 | ||
29.2.6 Surface Functionalization-Dependent Toxicity | 288 | ||
29.2.7 Surface Charge-Mediated Toxicity | 289 | ||
29.2.8 Magnetic Properties-Mediated Toxicity | 289 | ||
29.3 Interaction of Nanoparticles with Cells | 290 | ||
29.4 Materials and Their Toxicity | 294 | ||
29.4.1 Au Nanoparticle Toxicity | 294 | ||
29.4.2 Silver Nanoparticle Toxicity | 295 | ||
29.4.3 Copper Oxide Nanoparticle Toxicity | 296 | ||
29.4.4 Titanium Dioxide Nanoparticle Toxicity | 296 | ||
29.4.5 Cerium Oxide Nanoparticle Toxicity | 297 | ||
29.4.6 Zinc Oxide Nanoparticle Toxicity | 298 | ||
29.4.7 Toxicity of Cobalt and Cobalt Compound Nanoparticles | 298 | ||
29.4.8 Nickel Nanoparticle Toxicity | 298 | ||
29.4.9 Quantum Dot Toxicity | 299 | ||
29.4.10 Carbon Nanoparticle and Carbon Nanotube Toxicity | 299 | ||
29.4.11 Urban Air Pollution Nanoparticle Toxicity | 299 | ||
29.4.12 Comparative Toxicity of Nanoparticles | 302 | ||
29.5 Nanoparticle Biodistribution and Toxicity | 303 | ||
29.5.1 Inhalation | 304 | ||
29.5.2 Oral (Ingestion) Exposure | 308 | ||
29.5.3 Nanoparticles and Cardiovascular Diseases | 310 | ||
29.5.4 Nanoparticles, Placental Barrier and Fetus | 311 | ||
29.5.5 Interaction of Organs With Nanoparticles | 312 | ||
29.5.6 Central Nervous System and Nanoparticles | 312 | ||
29.5.7 The Immune System and Nanoparticles | 312 | ||
29.5.8 Nanoparticles and Diabetes | 313 | ||
29.6 Conclusions | 313 | ||
Abbreviation List | 314 | ||
References | 314 | ||
Chapter 30 - Legal Aspects of Environmental Safety Regulation in the Sphere of Nanotechnology in the Russian Federation | 325 | ||
30.1 Introduction | 325 | ||
30.2 International Overview | 327 | ||
30.3 System of Regulation of the Environmental Safety in the Russian Federation: General Overview | 328 | ||
30.3.1 Primary Regulation of Environmental Safety in the Russian Federation | 328 | ||
30.3.2 Secondary Regulation of Environmental Safety in the Russian Federation: General Overview | 331 | ||
30.4 Governance in the Sphere of Nano Safety in the Russian Federation | 333 | ||
30.5 Regulation of the Nano Safety in the Russian Federation | 336 | ||
30.5.1 Primary Regulation of Nano Safety in the Russian Federation | 338 | ||
30.5.2 Secondary/Subordinate Regulation of Nano Safety in the Russian Federation | 341 | ||
30.6 Conclusion | 343 | ||
References | 345 | ||
Chapter 31 - Nanotechnology and International Environmental Law: A Preliminary Assessment | 348 | ||
31.1 Introduction | 348 | ||
31.2 Nanotechnology, Environment and Legal Concerns: A Snapshot | 350 | ||
31.3 International Environmental Law: An Overview | 351 | ||
31.3.1 Preventive Principle | 353 | ||
31.3.2 Precautionary Principle | 354 | ||
31.4 Nanotechnology and International Environmental Law | 355 | ||
31.5 Nanotechnologies and International Organizations | 362 | ||
31.6 Discussion | 365 | ||
31.7 Conclusion | 369 | ||
References | 370 | ||
Section VIII - Monitoring and Analysis of Nanomaterials | 381 | ||
Chapter 32 - Fire and Explosion Risk Analysis for Nanomaterials | 383 | ||
32.1 Introduction | 383 | ||
32.2 Explosion Characteristics of Nanopowders | 384 | ||
32.2.1 The Minimum Ignition Energy for Micro- and Nano-Particles | 385 | ||
32.2.2 Minimum Explosive Concentration, Maximum Explosion Pressure, and Maximum Rate of Pressure Rise for Micro- and Nano-Aluminu... | 385 | ||
32.2.3 Maximum Explosion Pressure and Maximum Rate of Pressure Rise for Particles on Nano-Aluminum | 386 | ||
32.2.4 Minimum Ignition Temperature (MIT) of Titanium | 388 | ||
32.2.5 Data for Nano Zn, Nano Cu, Carbon Nanotubes, and Poly(methyl methacrylate) (PMMA) | 388 | ||
32.3 Risk of Nanometals Conveyed by Air | 389 | ||
32.3.1 Nano Ti, Fe, and Al Conveyed by Air in a 20 Liter Explosion Ball Experiment | 389 | ||
32.3.2 Risk of Old Oxygen Facility and Cylinder | 390 | ||
32.3.3 Nano Ti and Fe Conveyed by Air in a Nano-Grinder | 390 | ||
32.3.4 Study of the Relationship Between Air Velocity and Charge of Nanoparticles | 392 | ||
32.3.5 Hypothesis of Nano-Metal Fire Caused by High-Speed Air Transport | 392 | ||
32.4 Process Safety Control | 393 | ||
References | 394 | ||
Section IX - Future of Environmental Analysis | 397 | ||
Chapter 33 - Consequences of the Use of Nanomaterials for Environmental Analysis: Fate, Transport and Bioavailability in the Environment | 399 | ||
33.1 Introduction | 399 | ||
33.2 Nanomaterials in Environmental Analysis and Monitoring | 400 | ||
33.3 Environmental Consequences | 402 | ||
33.3.1 Dynamic Speciation of NMs | 403 | ||
33.3.2 Analytical Challenges | 406 | ||
33.3.3 Bioavailability | 407 | ||
33.4 Future Work and Pressing Problems | 409 | ||
References | 410 | ||
Chapter 34 - Conclusions | 413 | ||
References | 415 | ||
Subject Index | 416 |