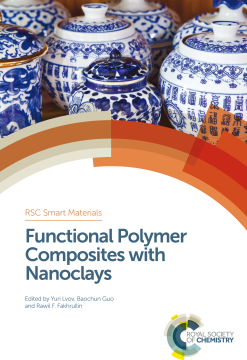
BOOK
Functional Polymer Composites with Nanoclays
Yuri Lvov | Baochun Guo | Rawil F Fakhrullin
(2016)
Additional Information
Book Details
Abstract
Polymer-clay nanocomposites have flame-retardant, antimicrobial, anticorrosion and self-healing properties, they are biocompatible and environmentally benign. Multiple types of clay minerals may be exfoliated or individually dispersed and then used as natural nanoparticle additives of different size and shape for composite formation. Loading polymers with clays increases their strength, however, it is only recently that such composites were prepared with controlled nanoscale organization allowing for the enhancement of their mechanical properties and functionality. Edited by pioneers in the field, this book will explain the great potential of these materials and will bring together the combined physico-chemical, materials science and biological expertise to introduce the reader to the vibrant field of nanoclay materials. This book will provide an essential text for materials and polymers scientists in industry and academia.
Table of Contents
Section Title | Page | Action | Price |
---|---|---|---|
Cover | Cover | ||
Functional Polymer Composites with Nanoclays | i | ||
Foreword | v | ||
Preface | vii | ||
Contents | ix | ||
Chapter 1 - Functional Nanocomposites Based on Fibrous Clays | 1 | ||
1.1 Introduction | 1 | ||
1.2 Modifications of Fibrous Clays for Use as Nanofillers | 4 | ||
1.3 Functional Polymer–Fibrous Clay Nanocomposites | 8 | ||
1.3.1 Functional Nanocomposites Based on Conventional Polymers | 9 | ||
1.3.2 Polymer–Clay Nanocomposites with Electrical Conductivity | 15 | ||
1.4 Functional Biopolymer–Fibrous Clay Nanocomposites | 20 | ||
1.4.1 Polylactic Acid and Other Biodegradable Polyesters | 20 | ||
1.4.2 Polysaccharides | 22 | ||
1.4.2.1 Bioplastics | 22 | ||
1.4.2.2 Environmental Remediation | 27 | ||
1.4.2.3 Biomedical Applications | 30 | ||
1.4.3 Proteins | 32 | ||
1.4.3.1 Bioplastics | 32 | ||
1.4.3.2 Biomedical Applications | 34 | ||
1.4.3.3 Biocatalytic Applications | 36 | ||
1.4.4 Lipids | 38 | ||
1.4.5 Nucleic Acids | 40 | ||
1.5 Conclusions | 40 | ||
Acknowledgements | 41 | ||
References | 41 | ||
Chapter 2 - Fibrillar Attapulgite–Rubber Nanocomposites | 54 | ||
2.1 Introduction | 54 | ||
2.2 Fibrillar Attapulgite | 56 | ||
2.2.1 Composition and Morphology of Fibrillar Attapulgite | 56 | ||
2.2.2 Physical Properties of Fibrillar Attapulgite | 58 | ||
2.2.2.1 Surface Properties of Fibrillar Attapulgite | 58 | ||
2.2.2.2 Adsorption Characteristics | 59 | ||
2.2.2.3 Ion Exchange Capacity | 60 | ||
2.2.3 Chemical Properties of Fibrillar Attapulgite | 60 | ||
2.3 Fibrillar Attapulgite–Rubber Composites | 60 | ||
2.3.1 Preparation Methods for Fibrillar Attapulgite–Rubber Composites | 61 | ||
2.3.2 Interface Design of Fibrillar Attapulgite–Rubber Composites | 64 | ||
2.3.2.1 Modification of Fibrillar Attapulgite by Coupling Agents | 64 | ||
2.3.2.1.1\rModification with Silane Coupling Agents.The silane coupling agents, including KH570, Si69, KH550, KH560, and A151 (see Table 2.... | 64 | ||
2.3.2.1.2\rModification with Organic Titanate Coupling Agents.The modification mechanism of titanate modification is similar to that of sil... | 68 | ||
2.3.2.1.3\rModification with Organic Aluminate Coupling Agents.Organic aluminate coupling agents have unique advantages in the surface trea... | 70 | ||
2.3.2.2 Modification of Fibrillar Attapulgite by Surfactant | 70 | ||
2.3.2.3 Acidification of Fibrillar Attapulgite | 71 | ||
2.3.2.4 Silver Nanoparticle-Immobilized Fibrillar Attapulgite | 72 | ||
2.3.3 Dispersion and Filler Network of Fibrillar Attapulgite in Elastomers | 74 | ||
2.3.3.1 Filler Network of Fibrillar Silicates in Elastomers | 74 | ||
2.3.3.1.1\rEffect of Rubber Viscosity.Acrylonitrile–butadiene rubber (NBR) was used as the matrix, the Mooney viscosity of which is very hi... | 74 | ||
2.3.3.1.2\rEffect of Rubber Polarity.As shown in Figure 2.22a, at the same NBR Mooney viscosity and NBR molecular weight, the higher the co... | 75 | ||
2.3.3.1.3\rEffect of Rubber Matrix.As shown in Figure 2.23, the AT–SiR compound shows the lowest dynamic modulus due to the highly flexible... | 75 | ||
2.3.3.1.4\rEffect of Silane Coupling Agent.As shown in Figure 2.24a, the Payne effects of modified AT–SBR compounds with different types of... | 79 | ||
2.3.3.2 Mechanism for Dispersion of Fibrillar Attapulgite in Elastomers | 79 | ||
2.3.4 Structure and Properties of Fibrillar Attapulgite–Rubber Composites | 80 | ||
2.3.4.1 Anisotropy of Fibrillar Attapulgite–Rubber Composites | 80 | ||
2.3.4.1.1\rInfluence of Preparation Process.Three mixing processes (A, B, and C) were used to prepare AT–rubber composites.56 In Process A,... | 81 | ||
2.3.4.1.2\rInfluence of AT Concentration.Table 2.7 further demonstrates that the AT–NBR composites prepared by Process C exhibit anisotropy... | 82 | ||
2.3.4.1.3\rInfluence of Rubber Matrix.As seen from Figure 2.28, the difference in tensile stress (σL − σT) at 100% strain between the paral... | 83 | ||
2.3.4.2 Mechanical Properties of Fibrillar Attapulgite–Rubber Composites with Different Modifiers | 85 | ||
2.3.4.3 Mechanical Properties of Fibrillar Attapulgite–Rubber Composites with Different Curing Systems | 87 | ||
2.3.4.4 Effect of Fibrillar Attapulgite on the Mechanical Properties of Different Rubber Matrices | 88 | ||
2.3.4.5 Comparison of Fibrillar Attapulgite with Other Fillers to Enhance the Mechanical Properties of Rubber Composites | 88 | ||
2.3.5 Synergistic Effect of Fibrillar Attapulgite and Other Microfibers on Mechanical Properties of Rubber | 90 | ||
2.4 Conclusion | 96 | ||
Acknowledgements | 96 | ||
References | 96 | ||
Chapter 3 - Rubber–Rectorite Composites with High Gas Barrier Properties | 100 | ||
3.1\rIntroduction | 100 | ||
3.1.1 Structure of Clays | 101 | ||
3.1.2 Types of Rubber–Clay Nanocomposites | 102 | ||
3.1.3 Preparation of Rubber–Clay Nanocomposites | 105 | ||
3.2 Gas Transport Mechanism | 106 | ||
3.2.1 Gas Transport in Rubber | 107 | ||
3.2.2 Gas Transport in Rubber–Clay Nanocomposites | 108 | ||
3.2.3 Characterization of Gas Barrier Properties | 111 | ||
3.3 Rubber–Rectorite Composites with High Gas Barrier Properties | 112 | ||
3.3.1 Rectorite Filled Non-Polar Elastomer Composites | 113 | ||
3.3.2 Rectorite Filled Polar Elastomer Composites | 118 | ||
3.4 Potential Approaches to Further Improve Gas Barrier Properties | 121 | ||
3.4.1 Improvement in Interfacial Interaction and Filler Dispersion | 121 | ||
3.4.2 Optimization of Filler Arrangement | 123 | ||
3.5 Other Properties of Rubber–Rectorite Nanocomposites | 124 | ||
3.5.1 Mechanical Properties | 124 | ||
3.5.2 Thermal Stability | 126 | ||
3.5.3 Aging Resistance and Oil Resistance | 127 | ||
3.6 Conclusions and Prospects | 127 | ||
Acknowledgements | 128 | ||
References | 128 | ||
Chapter 4 - Design and Physicochemical Characterization of Novel Organic–Inorganic Hybrids from Natural Aluminosilicate Nanotubes | 131 | ||
4.1 Imogolite and Halloysite | 131 | ||
4.2 Surface Modification of Imogolite and Application to Nanohybrids | 134 | ||
4.2.1 Modification of Imogolite Nanotubes with Alkyl Phosphate | 135 | ||
4.2.2 Polystyrene–Imogolite Nanohybrids | 137 | ||
4.2.3 Surface-Initiated Atom Transfer Radical Polymerization (SI-ATRP) from Imogolite Surface | 138 | ||
4.3 Surface Modification of Halloysite and Application to Nanohybrids | 142 | ||
4.3.1 Surface Modification of Halloysite | 142 | ||
4.3.2 Adsorption of Ferrocene into the Modified Halloysite and the Controlled Release | 147 | ||
4.3.3 Preparation of Inorganic Micelles by Bifunctionalization of Halloysite Nanotubes | 148 | ||
4.3.4 Polymer Growth from the Lumen of Halloysite | 150 | ||
4.3.5 Intelligent Polymer Nanocomposites with Flame Retardant Loaded Halloysite | 152 | ||
4.4 Conclusion | 154 | ||
Acknowledgements | 155 | ||
References | 155 | ||
Chapter 5 - Surface Modification of Halloysite | 157 | ||
5.1 Introduction | 157 | ||
5.2 Structural Characteristics and Surface Chemistry of HNTs | 158 | ||
5.2.1 Composition and Structure of HNTs | 158 | ||
5.2.2 General Considerations on the Modification Chemistry of HNTs | 159 | ||
5.3 Modification Chemistry and Techniques of HNTs | 162 | ||
5.3.1 Surface Silanization | 162 | ||
5.3.1.1 Reactivity of Silanes Towards HNTs | 162 | ||
5.3.1.2 Silanization of HNTs in Polymer-Based Composites | 163 | ||
5.3.1.3 Silanization of HNTs in HNT-Based Functional Composites | 164 | ||
5.3.2 Grafting Polymers onto HNTs | 166 | ||
5.3.2.1 Grafting To | 166 | ||
5.3.2.2 Grafting From | 167 | ||
5.3.3 Addition Reactions Between HNTs and (Iso)Cyanates | 169 | ||
5.3.4 Non-Covalent Interactions Between HNTs and Organic Molecules | 170 | ||
5.3.4.1 Hydrogen Bonding | 171 | ||
5.3.4.2 Electrostatic Interactions Between HNTs and Surfactants | 173 | ||
5.3.4.3 Charge Transferring | 174 | ||
5.3.5 Non-Covalent Interactions Between HNTs and Polymers | 174 | ||
5.3.5.1 Hydrogen Bonding with Polymers | 174 | ||
5.3.5.2 Electrostatic Interaction with Polymeric Electrolytes | 175 | ||
5.3.5.3 Plasma Polymerization | 177 | ||
5.3.6 Enlargement of the Lumen by Controlled Etching | 178 | ||
5.3.7 Selective Modification of the Lumen | 179 | ||
5.4 Concluding Remarks | 180 | ||
Acknowledgements | 181 | ||
References | 181 | ||
Chapter 6 - Halloysite Based Smart Hybrid Nanomaterials for the Solubilization of Hydrophobic Compounds in Aqueous Media | 187 | ||
6.1 Introduction | 187 | ||
6.2 Experimental | 188 | ||
6.2.1 HNT Functionalization with Surfactants | 189 | ||
6.2.2 Characterization | 190 | ||
6.2.2.1 Thermogravimetry | 191 | ||
6.2.2.2 Spectroscopy | 192 | ||
6.2.2.3 Microscopy | 193 | ||
6.2.2.4 Water Contact Angle | 193 | ||
6.2.2.5 DLS, ζ-Potential and Turbidimetry | 193 | ||
6.3 Results and Discussion | 194 | ||
6.3.1 Evidencing the Hybrid Formation | 194 | ||
6.3.2 Colloidal Behavior of the Hybrid in Solvent Media | 197 | ||
6.3.3 Solubilization Ability | 200 | ||
6.4 Conclusions | 204 | ||
Acknowledgements | 204 | ||
References | 204 | ||
Chapter 7 - Halloysite and Related Mesoporous Carriers for Advanced Catalysis and Drug Delivery | 207 | ||
7.1 Introduction | 207 | ||
7.2 Halloysite: Catalyst Carrier | 209 | ||
7.3 Halloysite: Carrier for Antibacterials | 213 | ||
7.4 Other Nanostructured Carriers for Drug Delivery | 215 | ||
7.5 Summary | 219 | ||
Acknowledgements | 221 | ||
References | 221 | ||
Chapter 8 - Application of Clay Materials as Nanocontainers for Self-Healing Coatings | 223 | ||
8.1 Introduction | 223 | ||
8.2 General Concept of Nanocontainer-Based Self-Healing Coatings | 225 | ||
8.3 Self-Healing Coatings Based on Ion-Exchange Nanoclays | 228 | ||
8.4 Self-Healing Coatings Based on Halloysites | 235 | ||
8.5 Conclusions and Outlook | 240 | ||
Acknowledgements | 241 | ||
References | 241 | ||
Chapter 9 - Flame Retardant Polymer–Halloysite Nanocomposites | 245 | ||
9.1 Introduction | 245 | ||
9.2 Use of HNTs as a Flame Retardant in Polymers | 246 | ||
9.3 Factors Affecting the Flame Retardancy of Polymer–HNTs Nanocomposites | 250 | ||
9.3.1 Dispersion of HNTs | 250 | ||
9.3.2 Modification of HNTs | 252 | ||
9.3.3 Hierarchical Structure in the Nanocomposites | 255 | ||
9.4 Synergistic Effects of HNTs and Other Flame Retardants | 256 | ||
9.4.1 Synergistic Effects with Intumescent Flame Retardants | 256 | ||
9.4.2 Synergistic Effects with Other Nanofillers | 261 | ||
9.5 Comparison Between HNTs and Other Clays | 262 | ||
9.5.1 Other Flame Retardant Clays in Polymers | 262 | ||
9.5.2 Comparing HNTs with Other Nanofillers in Flame Retardant Polymers | 264 | ||
9.6 Conclusion | 266 | ||
Acknowledgements | 267 | ||
References | 267 | ||
Chapter 10 - Polymer–Halloysite Composite Membranes for Ultrafiltration and Proton Exchange Applications | 271 | ||
10.1 Polymer–Halloysite Composite Membranes for Ultrafiltration Applications | 271 | ||
10.1.1 Introduction | 271 | ||
10.1.2 Special Structure and Properties of HNTs for Functionalization with Antibacterial Agents | 273 | ||
10.1.3 Application of HNT-Derived Nanomaterials for Antibacterial UF Membranes | 273 | ||
10.1.3.1 HNTs Functionalized with Inorganic NPs | 275 | ||
10.1.3.2 HNTs Functionalized with Organic Antibacterial Agents (N-halamine) | 279 | ||
10.1.3.3 HNTs Functionalized with Bio-Enzymatic Antibacterial Agents (Lysozyme) | 280 | ||
10.2 Polymer–Halloysite Composite Membranes for Proton Exchange Applications | 282 | ||
10.2.1 Introduction | 282 | ||
10.2.2 HNT-Incorporated Composite PEMs | 283 | ||
10.2.3 Modified-HNT-Incorporated Composite PEMs | 286 | ||
10.3 Conclusions | 296 | ||
Acknowledgements | 297 | ||
References | 297 | ||
Chapter 11 - Rubber Functionalized with Halloysite Loaded with Antioxidants and Antibacterials | 301 | ||
11.1 Introduction | 301 | ||
11.2 Rubber–Halloysite Nanocomposites | 302 | ||
11.2.1 Rubber–HNTs Nanocomposites for Engineering Applications | 302 | ||
11.2.2 Elastomer–HNTs Nanocomposites for Biomedical Applications | 306 | ||
11.3 Antioxidative Rubber Composites | 306 | ||
11.3.1 Aging of Elastomers and Their Antioxidative Progress | 306 | ||
11.3.2 Halloysite Loaded with Antioxidants | 308 | ||
11.3.3 Rubber Functionalized with Antioxidant-Loaded Halloysite | 308 | ||
11.3.3.1 Highly Aging-Resistant SBR Elastomers with Antioxidant-Loaded Halloysites | 308 | ||
11.3.3.2 Halloysite Immobilized with Antioxidant and Its Rubber Composites | 312 | ||
11.3.3.3 The Advantages of Rubber Functionalized with Antioxidant-Loaded HNTs | 315 | ||
11.4 Antibacterial Rubber Composite | 316 | ||
11.4.1 Applications of Antibacterials in Rubber Composites | 316 | ||
11.4.2 HNTs Loaded with Antibacterials | 320 | ||
11.4.3 Rubber Functionalized with Antibacterial-Loaded HNTs | 323 | ||
11.5 Conclusions | 323 | ||
Acknowledgements | 324 | ||
References | 324 | ||
Chapter 12 - Halloysite–Dopamine Hybrid Nanotubes to Immobilize Biomacromolecules | 329 | ||
12.1 Halloysite Nanotubes for Biomacromolecule Immobilization | 329 | ||
12.2 Dopamine Chemistry for Biomacromolecule Immobilization: Nature’s Choice | 332 | ||
12.3 Dopamine Modified Halloysite Nanotubes for Enzyme Immobilization | 335 | ||
12.3.1 Fabrication Procedure | 335 | ||
12.3.2 Morphologies of Dopamine Modified Halloysite Nanotubes | 335 | ||
12.3.3 Surface Chemical Structures of Dopamine Modified Halloysite Nanotubes | 336 | ||
12.3.4 Enzyme Immobilization on Dopamine Modified Halloysite Nanotubes | 338 | ||
12.4 Dopamine Modified Halloysite Nanotube Aggregates for Enzyme Immobilization | 342 | ||
12.4.1 Construction of Dopamine-Modified Halloysite Nanotube Aggregates | 342 | ||
12.4.2 Hierarchical Structures of Dopamine-Modified Halloysite Nanotube Aggregates | 343 | ||
12.4.3 Enzyme Immobilization on Dopamine Modified Halloysite Nanotube Aggregates | 347 | ||
12.5 Other Potential Applications of Halloysite–Dopamine Hybrid Nanotubes for Biomacromolecule Immobilization | 350 | ||
12.6 Conclusion | 351 | ||
References | 351 | ||
Chapter 13 - Halloysite Clay Nanotubes for Long Acting Controlled Release of Drugs and Proteins | 354 | ||
13.1 Introduction | 354 | ||
13.2 Loading and Release of Drugs with Untreated Halloysite Nanotubes | 355 | ||
13.3 Acid and Alkali Activation/Etching of Halloysite Nanotubes | 360 | ||
13.4 Modification of the Inner and Outer Surfaces of Halloysite | 362 | ||
13.5 End Stoppers and Encapsulation | 365 | ||
13.6 Antibacterial Halloysite Nanocomposites | 368 | ||
13.7 Protein and DNA Loading | 370 | ||
13.8 Conclusion | 376 | ||
References | 376 | ||
Chapter 14 - Biocompatible Electrospun Polymer–Halloysite Nanofibers for Sustained Release | 379 | ||
14.1 Introduction | 379 | ||
14.2 Electrospinning Technology | 380 | ||
14.2.1 Traditional Electrospun Nanofibers for Drug Delivery | 383 | ||
14.2.2 Coaxial and Emulsion Electrospinning Nanofibers for Drug Delivery | 383 | ||
14.2.3 Novel Electrospinning Nanofibers for Drug Delivery | 384 | ||
14.3 Electrospun Halloysite–Polymer Nanofibers | 386 | ||
14.4 Properties of Electrospun Drug-Loaded Halloysite–Polymer Nanofibers | 387 | ||
14.4.1 Mechanical Reinforcement | 387 | ||
14.4.2 Sustained Drug Release Effect | 387 | ||
14.4.3 Drug Activity Maintenance | 390 | ||
14.4.4 Biocompatibility and Biodegradability | 393 | ||
14.5 Conclusion and Outlook | 393 | ||
Abbreviations | 395 | ||
Acknowledgements | 395 | ||
References | 396 | ||
Chapter 15 - Toxicological Evaluation of Clay Nanomaterials and Polymer–Clay Nanocomposites | 399 | ||
15.1 Introduction | 399 | ||
15.2 Evaluation of Toxicity of Nanoclays | 402 | ||
15.2.1 Nanoclay Toxicity Towards Unicellular Prokaryotic and Eukaryotic Organisms | 402 | ||
15.2.2 Toxicological Evaluation of Nanoclays Using Mammalian Cell Cultures | 405 | ||
15.2.3 Nanoclay Toxicity Evaluation In vivo Using Multicellular Eukaryotic Organisms | 408 | ||
15.3 Toxicity of Nanoclay-Doped Polymer Nanocomposites | 410 | ||
15.3.1 In vitro Study of Polymer–Nanoclay Composites on Mammalian Cell Lines | 411 | ||
15.3.2 Evaluation of Implanted Clay-Doped Nanocomposite Toxicity In vivo | 413 | ||
15.4 Conclusion | 417 | ||
Acknowledgements | 417 | ||
References | 417 | ||
Subject Index | 420 |