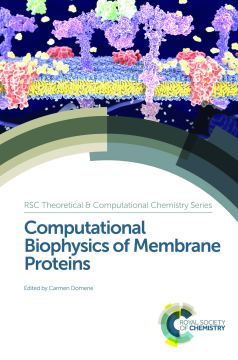
Additional Information
Book Details
Abstract
Exploring current themes in modern computational and membrane protein biophysics, this book presents a comprehensive account of the fundamental principles underlying different methods and techniques used to describe the intriguing mechanisms by which membrane proteins function. The book discusses the experimental approaches employed to study these proteins, with chapters reviewing recent crucial structural advances that have allowed computational biophysicists to discern how these molecular machines work. The book then explores what computational methods are available to researchers and what these have taught us about three key families of membrane proteins: ion channels, transporters and receptors. The book is ideal for researchers in computational chemistry and computational biophysics.
Carmen Domene is a Reader in Computational Chemistry at King’s College London, UK. Her research group applies principles from quantum mechanics and statistical thermodynamics to model biomolecular phenomena with computer simulations.
Table of Contents
Section Title | Page | Action | Price |
---|---|---|---|
Cover | Cover | ||
Contents | v | ||
Chapter 1 Introduction to the Structural Biology of Membrane Proteins | 1 | ||
1.1 Introduction | 1 | ||
1.2 Membrane Features | 2 | ||
1.3 Lipid Polymorphism | 5 | ||
1.4 Classes of Membrane Proteins | 7 | ||
1.4.1 α-Helical Bundles\r | 7 | ||
1.4.2 β-Barrels\r | 8 | ||
1.5 Functions of Membrane Proteins | 9 | ||
1.5.1 Channels | 10 | ||
1.5.2 Transporters | 11 | ||
1.5.3 Enzymes | 14 | ||
1.5.4 Receptors | 14 | ||
1.6 Membrane Protein Complexes | 15 | ||
1.7 Conclusions | 17 | ||
References | 17 | ||
Chapter 2 Molecular Dynamics Simulations: Principles and Applications for the Study of Membrane Proteins | 19 | ||
2.1 Introduction | 19 | ||
2.2 Classical Molecular Dynamics | 20 | ||
2.2.1 Additive Force Fields | 22 | ||
2.2.2 Polarisable Force Fields | 24 | ||
2.2.3 Practical and Technical Considerations | 25 | ||
2.2.4 Applications | 29 | ||
2.3 Coarse-grained Molecular Dynamics Simulations | 30 | ||
2.4 Ab initio Molecular Dynamics | 33 | ||
2.5 Enhanced Sampling Techniques and Free Energy Methods | 34 | ||
2.6 Conclusions | 40 | ||
References | 40 | ||
Chapter 3 Free Energy Calculations for Understanding Membrane Receptors | 59 | ||
3.1 Introduction | 59 | ||
3.2 The Basics of Free Energy Calculations | 60 | ||
3.2.1 The Parametric Formulation of Free Energy Calculations | 60 | ||
3.2.2 Ergodicity, Variance Reduction Strategies, and the Transition Coordinate | 63 | ||
3.3 Free Energy Perturbation Methods | 65 | ||
3.3.1 Theoretical Background | 65 | ||
3.3.2 Alchemical Transformations | 70 | ||
3.4 Probability Distribution Methods | 73 | ||
3.5 Thermodynamic Integration | 75 | ||
3.5.1 Theoretical Background | 75 | ||
3.5.2 Adaptive Biasing Force Method | 78 | ||
3.6 Replica Exchange for Enhanced Sampling in Configurational Space | 80 | ||
3.7 Applications of Free Energy Calculations: Case Studies | 81 | ||
3.7.1 Binding of Anesthetic Ligands to Receptors | 82 | ||
3.7.2 Free Energies of Ions across Channels | 84 | ||
3.7.3 Conformational Transitions in Receptors | 86 | ||
3.8 Non-equilibrium Properties from Free Energy Calculations | 88 | ||
3.8.1 Theoretical Background | 90 | ||
3.8.2 Example - the Leucine-Serine Channel | 93 | ||
3.9 Summary and Conclusions | 96 | ||
References | 98 | ||
Chapter 4 Non-atomistic Simulations of Ion Channels | 107 | ||
4.1 Introduction | 107 | ||
4.2 Methods Based on Continuum Distributions of Ions | 111 | ||
4.2.1 Poisson-Boltzmann | 112 | ||
4.2.2 Poisson-Nernst-Planck | 117 | ||
4.2.3 Improvements of Classical Continuum Theories of Electrolytes | 119 | ||
4.3 Particle-based Methods | 122 | ||
4.3.1 Brownian Dynamics | 124 | ||
4.3.2 Monte Carlo | 127 | ||
4.4 Methods to Include Atomic Detail in Non-atomistic Models | 128 | ||
4.4.1 Atomic Detail in Brownian Dynamics | 129 | ||
4.4.2 Atomic Detail in Continuum Models | 132 | ||
4.5 Concluding Remarks | 133 | ||
References | 133 | ||
Chapter 5 Experimental and Computational Approaches to Study Membranes and Lipid–Protein Interactions | 137 | ||
5.1 Introduction | 137 | ||
5.1.1 Membrane Components | 138 | ||
5.2 Role of Membrane Lipids in Membrane Protein Organization and Function | 139 | ||
5.3 Mechanisms for Lipid Regulation of Membrane Proteins | 140 | ||
5.3.1 Specific Membrane Effects | 140 | ||
5.3.2 Non-specific Membrane Effects | 142 | ||
5.4 Range of Time Scales Exhibited by Membranes | 142 | ||
5.5 Lipid-Protein Interactions: Insights from Experimental Approaches | 144 | ||
5.5.1 Determining Near-neighbor Relationships in Membranes: Interaction of Melittin with Membrane Cholesterol utilizing FRET | 144 | ||
5.5.2 Interaction of the Actin Cytoskeleton with GPCRs: Application of FRAP | 147 | ||
5.6 Computational Approaches to Study Membrane Organization and Lipid-Protein Interactions | 149 | ||
5.6.1 Simulating Single Component and Multi-component Bilayers | 151 | ||
5.6.2 Atomistic Simulations Elucidating Lipid-Protein Interactions | 151 | ||
5.6.3 Coarse-grain Methods to Analyze Membrane Protein Interactions | 153 | ||
5.6.4 Enhanced Sampling Methods | 154 | ||
5.7 Future Perspectives: The Road Ahead | 155 | ||
Acknowledgments | 155 | ||
References | 155 | ||
Chapter 6 Computer Simulation of Ion Channels | 161 | ||
6.1 Introduction to Ion Channels | 161 | ||
6.2 Questions that can be Addressed and Associated Timescales | 165 | ||
6.3 Ion Permeation | 169 | ||
6.4 Ion Selectivity | 174 | ||
6.4.1 Na+/Ca2+ Selection | 174 | ||
6.4.2 Na+/K+ Selection | 177 | ||
6.5 Channel Gating | 181 | ||
6.6 Interactions of Channels with Drugs and Toxins | 184 | ||
6.6.1 Toxin-Channel Interactions | 184 | ||
6.6.2 Channel Blockage by Small Molecules | 187 | ||
6.7 Conclusions | 189 | ||
Acknowledgments | 190 | ||
References | 190 | ||
Chapter 7 Computational Characterization of Molecular Mechanisms of Membrane Transporter Function | 197 | ||
7.1 Membrane Transport – A Fundamental Biological Process | 197 | ||
7.2 Substrate Binding and Unbinding | 200 | ||
7.2.1 Spontaneous Binding Simulations Revealing a Binding Mechanism and Site | 201 | ||
7.2.2 Proposing Substrate Binding Sites through Molecular Docking | 202 | ||
7.2.3 Unraveling Substrate Release Pathways | 204 | ||
7.3 Capturing Localized Transporter Motions with Equilibrium Molecular Dynamics | 205 | ||
7.3.1 Substrate-induced Structural Changes of an Antiporter | 206 | ||
7.3.2 Gating Elements in a Neurotransmitter Transporter | 206 | ||
7.4 Computational Description of Global Structural Transitions in Membrane Transporters | 209 | ||
7.4.1 Nonequilibrium Simulation of Structural Changes | 210 | ||
7.4.2 Application to an ABC Transporter | 212 | ||
7.5 Water within Transporters | 215 | ||
7.5.1 Water Leaks in Transporters | 216 | ||
7.5.2 Water in Proton Pathways | 216 | ||
7.6 The Lipid Frontier | 219 | ||
7.6.1 Why Now? Initial Barriers to Simulating Lipid-Protein Interactions | 219 | ||
7.6.2 Computational Probes of Lipid-Protein Interactions | 220 | ||
7.7 Concluding Remarks | 221 | ||
Acknowledgments | 222 | ||
References | 223 | ||
Chapter 8 Computational Studies of Receptors | 237 | ||
8.1 Introduction | 237 | ||
8.2 Network Models Can Provide Insight into Large-scale Conformational Changes | 239 | ||
8.3 Network Models to Examine Gating | 241 | ||
8.4 Network Models to Compare Dynamics | 242 | ||
8.5 Network Models to Suggest Novel Mechanisms for Modulation | 244 | ||
8.6 Molecular Dynamics to Aid Crystallographic Interpretation | 245 | ||
8.7 Molecular Dynamics to Move between States | 247 | ||
8.8 Molecular Dynamics to Refine Working Models | 249 | ||
8.9 Molecular Dynamics to Explain the Effects of Ions and Water | 251 | ||
8.10 Molecular Dynamics to Quantify Free Energy Requirements | 253 | ||
8.11 Conclusions | 255 | ||
References | 255 | ||
Subject Index | 259 |