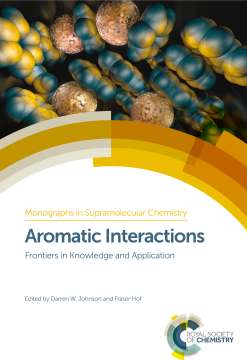
Additional Information
Book Details
Abstract
The field of aromatic interactions, the fundamental nature of substituent effects and the identification of contacts between anions and aromatic systems have generated stimulating arguments in recent years. New theoretical frameworks have been developed and tested and aromatic interactions have emerged as potential solutions for varied problems in biology and materials science.
This book provides a wide ranging survey of the latest findings and advances surrounding aromatic interactions, stretching from the fundamentals to modern applications in synthesis, biology and materials chemistry. It also discusses computational, experimental and analytical approaches to understanding these interactions, including pi-pi, anion-pi, and cation-pi interactions.
Aromatic Interactions: Frontiers in Knowledge and Application is a useful text for advanced students and researchers, and appeals to those working within the fields of supramolecular chemistry, computational chemistry and thermodynamics.
Darren W. Johnson received his BS in Chemistry at the University of Texas at Austin in 1996, where he performed undergraduate research under the direction of Prof. Jonathan Sessler. He earned his PhD in Chemistry in 2000 from UC-Berkeley working with Prof. Kenneth Raymond, and he then spent two years at the Scripps Research Institute as an NIH post-doctoral fellow with Prof. Julius Rebek, Jr. He joined the chemistry faculty at the University of Oregon in 2003, where he holds the rank of Professor. Research in his group uses supramolecular chemistry as a tool to explore a variety of problems in coordination chemistry, molecule/ion recognition and inorganic cluster synthesis.
Fraser Hof received his B.Sc. at the University of Alberta in 1998, completing an Honour’s thesis with Prof. Neil Branda. He carried out his Ph.D. studies (2003) in self-assembly at the Scripps Research Institute with Julius Rebek, Jr., and was a post-doctoral fellow (2003-2005) in medicinal chemistry with François Diederich at ETH Zurich. He has been at the at the University of Victoria, where he is currently the Canada Research Chair in Supramolecular and Medicinal Chemistry, since 2005. His research program revolves around molecular recognition and epigenetic protein methylation pathways. It includes basic research projects in protein binding, molecular recognition in pure water, and solvation and salt effects in competitive media. Applications include efforts to make supramolecular affinity reagents and sensors as tools for epigenetics research, as well as medicinal chemistry approaches that target the ‘aromatic cage’ binding pockets of several epigenetic reader proteins.
Table of Contents
Section Title | Page | Action | Price |
---|---|---|---|
Cover | Cover | ||
Aromatic Interactions Frontiers in Knowledge and Application | i | ||
Preface | v | ||
Contents | xi | ||
Chapter 1 - Modern Computational Approaches to Understanding Interactions of Aromatics | 1 | ||
1.1 Introduction and Background | 1 | ||
1.1.1 Arene–Arene Interactions | 2 | ||
1.1.2 Cation–Arene Interactions | 3 | ||
1.1.3 Beyond the Aromatic Quadrupole Moment | 4 | ||
1.2 Computational Approaches to Understanding Arene–Arene Interactions | 5 | ||
1.2.1 The Nature of Arene–Arene Interactions | 5 | ||
1.2.2 Predicting the Strength of Arene–Arene Interactions | 9 | ||
1.3 Computational Approaches to Understanding Cation–Arene Interactions | 10 | ||
1.3.1 The Nature of Cation–Arene Interactions | 10 | ||
1.3.2 Predicting the Strength of Cation–Arene Interactions | 13 | ||
1.4 Summary | 14 | ||
References | 15 | ||
Chapter 2 - Role of Aromatic Interactions in Directing Organic Reactions | 18 | ||
2.1 Introduction and Background | 18 | ||
2.2 Aromatic Interactions of Relevance to Organic Reactions | 19 | ||
2.3 Aromatic Interactions in Non-Catalytic Reactions | 22 | ||
2.4 Aromatic Interactions in Transition-Metal Catalyzed Reactions | 24 | ||
2.5 Aromatic Interactions in Organocatalysis | 25 | ||
2.6 Aromatic Interactions in Cooperative Catalysis | 31 | ||
2.7 Reactions Directed by Anion–π Interactions | 31 | ||
2.8 Reactions Directed by π–π+ Interactions | 33 | ||
2.9 Concluding Remarks | 34 | ||
Acknowledgements | 34 | ||
References | 34 | ||
Chapter 3 - Anion–π Interactions: Theoretical Studies, Supramolecular Chemistry and Catalysis | 39 | ||
3.1 Introduction | 39 | ||
3.2 Physical Nature | 41 | ||
3.3 Anion–π Interactions in Solution | 46 | ||
3.3.1 Supramolecular Chemistry and Synthetic Receptors Involving Anion–π Interactions | 46 | ||
3.3.2 Anion–π Interactions in Reaction Catalysis | 65 | ||
3.3.3 Ionic Liquids | 74 | ||
3.4 On the Importance of Anion–π Interactions in the Solid State | 76 | ||
3.4.1 Charge Neutral π-Systems | 76 | ||
3.4.2 Aromatic Ligands Coordinated to Metal Ions | 83 | ||
3.4.3 Positively Charged or Protonated Aromatics | 86 | ||
3.5 Outlook | 88 | ||
Acknowledgements | 88 | ||
References | 89 | ||
Chapter 4 - A New Non-Covalent Bonding Mode in Supramolecular Chemistry: Main Group Element Lone-Pair–π(arene) Interactions | 98 | ||
4.1 Introduction | 98 | ||
4.2 Methodology | 100 | ||
4.3 Overview of M(lp)⋯π(arene) Interactions | 102 | ||
4.3.1 Indium(i) | 102 | ||
4.3.2 Thallium(i) | 102 | ||
4.3.3 Tin(ii) | 104 | ||
4.3.4 Lead(ii) | 105 | ||
4.3.5 Arsenic(iii) | 106 | ||
4.3.6 Antimony(iii) | 108 | ||
4.3.7 Bismuth(iii) | 108 | ||
4.3.8 Selenium(ii, iv) | 109 | ||
4.3.9 Tellurium(ii, iv) | 115 | ||
4.4 Biological Relevance | 116 | ||
4.5 Conclusions and Outlook | 118 | ||
Acknowledgements | 119 | ||
References | 119 | ||
Chapter 5 - Solution-Phase Measurements of Aromatic Interactions | 124 | ||
5.1 Introduction | 124 | ||
5.2 Types of Model Systems | 125 | ||
5.2.1 Bimolecular Model Systems | 125 | ||
5.2.2 Unimolecular Model System | 126 | ||
5.2.3 Thermodynamic Approach | 126 | ||
5.2.4 Kinetic Approach | 127 | ||
5.2.5 Analysis of Experiment Models | 127 | ||
5.2.6 Isolation of the Interaction Energy | 128 | ||
5.3 Case Studies of Solution-Phase Measurements of Aromatic Interactions | 129 | ||
5.3.1 Aromatic Stacking Interactions | 129 | ||
5.3.1.1 Hunter’s Supramolecular Zipper Complex | 130 | ||
5.3.1.2 Shimizu’s Atropisomeric N-Arylimide Aromatic Stacking Balance | 133 | ||
5.3.1.3 Swager and Houk’s Diels–Alder Reaction Aromatic Stacking Model System | 137 | ||
5.3.1.4 Cozzi and Siegel’s 1,9-Diarylnaphthalene π-Cofacial Model | 138 | ||
5.3.1.5 Other Model Systems for Studying Aromatic Stacking Interactions in Solution | 140 | ||
5.3.2 Aromatic Edge-to-Face Interactions | 140 | ||
5.3.2.1 Wilcox’s ETF Molecular Torsional Balance | 141 | ||
5.3.2.2 Hsung’s 4+3 Cycloaddition ETF Model System | 143 | ||
5.3.2.3 Other Model Systems for Studying Aromatic ETF Interactions in Solution | 145 | ||
5.3.3 Aliphatic CH–π Interactions | 145 | ||
5.3.3.1 Asensio’s Imine/Hemiaminal CH–π Model | 145 | ||
5.3.3.2 Zondlo’s Proline Tetrapeptide CH–π Model | 149 | ||
5.3.3.3 Other Model Systems for Studying Aliphatic CH–π Interactions in Solution | 150 | ||
5.3.4 Aromatic Cation–π Interactions | 150 | ||
5.3.4.1 Dougherty’s Cyclophane Cation–π Model | 151 | ||
5.3.4.2 Waters’s β-Hairpin Peptide Cation–π Model | 152 | ||
5.3.4.3 Jacobsen’s Enantioselective Polycyclization Cation–π Model | 153 | ||
5.3.4.4 Waters’s Benzyl Pyridinium Cation–π Model | 154 | ||
5.3.4.5 Other Model Systems for Studying Cation–π Interactions in Solution | 155 | ||
5.3.5 Anion–π Interaction | 155 | ||
5.3.5.1 Wang’s Calix[2]arene[2]triazine Anion–π Model | 156 | ||
5.3.5.2 Ballester’s Calix[4]pyrrole Anion–π Model | 157 | ||
5.3.5.3 Other Model Systems for Studying Anion–π Interactions in Solution | 159 | ||
5.3.6 Lone Pair–π Interaction | 159 | ||
5.3.6.1 Gung’s Oxygen lp–π Triptycene Balance | 159 | ||
5.3.6.2 Motherwell’s lp–π Seesaw Balance | 161 | ||
5.4 Conclusion | 162 | ||
Acknowledgements | 163 | ||
References | 163 | ||
Chapter 6 - Molecular Recognition of Aromatic Peptides and Proteins in Nature and by Design | 172 | ||
6.1 Overview | 172 | ||
6.2 Aromatic Amino Acids | 173 | ||
6.3 Aromatic Residues in Biological Complexes | 175 | ||
6.4 Designed Ligands | 180 | ||
6.5 Efficiency of Aromatic Residues | 182 | ||
6.6 Synthetic Receptors | 183 | ||
6.7 Synthetic Receptors for Amino Acids | 184 | ||
6.7.1 Aromatic Amino Acid Recognition by Viologen-Containing Receptors | 184 | ||
6.7.2 Aromatic Amino Acid Recognition by Cyclodextrins | 186 | ||
6.7.3 Aromatic Amino Acid Recognition by Cucurbiturils | 188 | ||
6.7.4 Recognition of Non-Aromatic Amino Acids by Synthetic Receptors | 189 | ||
6.8 Recognition of Aromatic Peptides by Synthetic Receptors | 192 | ||
6.8.1 Early Receptors for Peptides | 193 | ||
6.8.2 Peptide Recognition by Coordination Complexes | 195 | ||
6.8.3 Peptide Recognition by Cucurbit[n]urils | 197 | ||
6.9 Recognition of Aromatic Sites on Proteins by Synthetic Receptors | 203 | ||
6.10 Lessons Learned and Challenges | 206 | ||
Acknowledgements | 208 | ||
References | 208 | ||
Chapter 7 - Cation–π Interactions in Biomolecular Recognition | 214 | ||
7.1 The Nature of the Cation–π Interaction | 214 | ||
7.2 Model Systems for Cation–π Interactions in Aqueous Solution | 216 | ||
7.2.1 Applications of Synthetic Systems that Mediate Cation–π Interactions | 218 | ||
7.3 Cation–π Interactions in Proteins | 218 | ||
7.4 Cation–π Interactions in Nucleic Acids | 225 | ||
7.5 Cation–π Interactions in Lipids | 229 | ||
7.6 Conclusions | 232 | ||
References | 232 | ||
Chapter 8 - Aromatic Molecules on Metallic Surfaces: Structure and Reactivity | 238 | ||
8.1 Introduction | 238 | ||
8.2 Structural Investigations of Aromatic Molecules on Surfaces | 240 | ||
8.2.1 General Surface Characterization Techniques | 240 | ||
8.2.2 Visualization Techniques with Sub-Molecular Resolution: STHM and nc-AFM | 241 | ||
8.3 Reactivity of Aromatic Molecules on Metallic Surfaces | 247 | ||
8.3.1 Surface-Mediated Polymerization | 248 | ||
8.3.1.1 Polymerization via Terminal Acetylene Coupling | 248 | ||
8.3.1.2 Polymerization of Halogenated Precursors | 250 | ||
8.3.1.3 Polymerization Through Dynamic Covalent Chemistry | 252 | ||
8.3.2 Cyclodehydrogenation Reactions | 255 | ||
8.3.3 Metal-Coordination Reactions | 257 | ||
8.3.4 Graphene Nanoribbon Synthesis | 261 | ||
8.4 Conclusions | 269 | ||
References | 269 | ||
Subject Index | 277 |