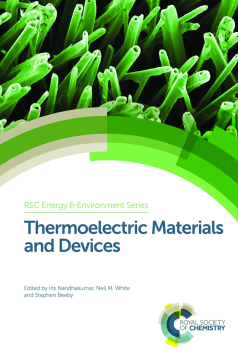
Additional Information
Book Details
Abstract
Thermal energy harvesting is predicted to become a global, billion-pound market by 2020. This book provides a current perspective of recent developments and trends within thermoelectric materials and devices for power energy harvesting applications. The book highlights the potential of thermoelectrics in the context of a low carbon energy economy, and features in-depth coverage of a range of different fabrication methods for thermoelectric materials including electrodeposition. Topics covered include layered and pseudo-layered materials, thermoelectric oxides, nano- and micro-fabrication techniques, high-throughput thermoelectric measurement techniques and power mining. This book is ideal for researchers and industrialists in materials science.
Iris Nandhakumar is Merck Lecturer in Materials Science within Chemistry at the University of Southampton. Her research focusses on the preparation and characterization of novel materials with controlled nanoarchitectures.
Neil M. White is Professor of Intelligent Sensor Systems in the Department of Electronics and Computer Science at the University of Southampton. His research interests include thick-film sensors, intelligent instrumentation, microelectromechanical systems, self-powered microsensors and sensor networks.
Stephen Beeby is Professor of Electronic Systems and Devices at Southampton University. He has been awarded a prestigious EPSRC Leadership Fellowship on the subject of Energy Harvesting Materials for Smart Fabrics and Interactive Textiles.
Table of Contents
Section Title | Page | Action | Price |
---|---|---|---|
Cover | Cover | ||
Contents | vii | ||
Preface | v | ||
Chapter 1 Zintl Phases: Recent Developments in Thermoelectrics and Future Outlook | 1 | ||
1.1 Introduction | 1 | ||
1.1.1 Definition of Zintl Phases | 1 | ||
1.1.2 Charge Counting/Formal Valence Rules | 2 | ||
1.1.3 Thermoelectric Zintl Compounds | 4 | ||
1.2 Thermal Properties | 7 | ||
1.2.1 Theory behind Low KL in Complex Materials | 8 | ||
1.2.2 Case Studies | 11 | ||
1.3 Electronic Transport | 13 | ||
1.3.1 Controlling and Optimizing Carrier Concentration | 14 | ||
1.3.2 Limits to Controlling Carrier Concentration | 15 | ||
1.3.3 Band Structure Requirements | 16 | ||
1.3.4 Carrier Relaxation Time | 18 | ||
1.4 Future Opportunities for Zintl Thermoelectric Materials | 19 | ||
Acknowledgments | 19 | ||
References | 19 | ||
Chapter 2 Chalcogenide Thermoelectric Materials | 27 | ||
2.1 Introduction | 27 | ||
2.2 Synthesis | 30 | ||
2.3 Low-dimensionality in Chalcogenides | 32 | ||
2.3.1 Layered Dichalcogenides | 32 | ||
2.3.2 Intercalated Phases | 35 | ||
2.3.3 Structurally-related Phases | 37 | ||
2.4 Shandite-related Phases | 37 | ||
2.5 Rocksalt-derived Chalcogenides | 40 | ||
2.6 Tin Selenide and Related Materials | 45 | ||
2.7 Oxychalcogenides | 47 | ||
2.8 Copper-containing Chalcogenides with Low Thermal Conductivities | 49 | ||
2.9 Concluding Remarks | 51 | ||
References | 52 | ||
Chapter 3 Thermoelectric Oxides | 60 | ||
3.1 Introduction | 60 | ||
3.2 Manufacture | 63 | ||
3.3 Composition and Atomic Structure | 67 | ||
3.3.1 Strontium Titanate Based Materials | 67 | ||
3.3.2 Cobaltites | 70 | ||
3.3.3 Calcium Manganate Based Materials | 73 | ||
3.3.4 Zinc Oxide | 74 | ||
3.4 Microstructure | 74 | ||
3.5 Module Manufacture | 76 | ||
3.6 Conclusions | 77 | ||
References | 78 | ||
Chapter 4 Nano- and Micro-fabrication Techniques for Improving Thermoelectric Materials and Generators | 83 | ||
4.1 Introduction | 83 | ||
4.2 Low-dimensional Electrical Conductivity | 85 | ||
4.3 The Seebeck Coefficient and Low-dimensional Modifications | 92 | ||
4.4 Thermal Conductivity | 93 | ||
4.5 Potential Improvements to Thermoelectrics from Nano- and Micro-structures | 97 | ||
4.6 Micro-fabrication of Thermoelectric Generators | 102 | ||
4.7 Conclusions | 107 | ||
References | 107 | ||
Chapter 5 Review of the Methods for Thermal Conductivity Measurements Most Appropriate for Thermoelectric Materials | 109 | ||
5.1 Introduction | 109 | ||
5.1.1 Thermoelectric Challenges | 109 | ||
5.1.2 Thermal Conductivity Measurements | 110 | ||
5.2 Steady-state Methods | 112 | ||
5.2.1 Guarded Hot Plate | 112 | ||
5.2.2 High-temperature Measurements | 116 | ||
5.2.3 Radial Heat Flow Method | 117 | ||
5.2.4 High-temperature Measurements | 118 | ||
5.2.5 Longitudinal Heat Flow | 119 | ||
5.2.6 High-temperature Measurements | 123 | ||
5.2.7 Heat-flow Meter | 123 | ||
5.3 Transient Methods | 125 | ||
5.3.1 Metrological Approach | 126 | ||
5.4 Conclusions | 128 | ||
Acknowledgments | 128 | ||
References | 128 | ||
Chapter 6 High-throughput Thermoelectric Measurement Techniques | 133 | ||
6.1 Introduction | 133 | ||
6.2 Multifunctional Probes | 134 | ||
6.2.1 Measuring Principles | 134 | ||
6.2.2 Design and Fabrication of Multifunctional Probes | 135 | ||
6.2.3 Measurement Systems and Procedures | 137 | ||
6.2.4 Precision, Accuracy and Rapidness | 143 | ||
6.3 Impedance Spectroscopy | 146 | ||
6.3.1 Fundamentals of Impedance Spectroscopy | 146 | ||
6.3.2 Theoretical Framework | 147 | ||
6.3.3 Experimental Set-up | 150 | ||
6.3.4 Measurement Analysis and Applications | 151 | ||
6.4 Conclusions | 154 | ||
Acknowledgments | 155 | ||
References | 155 | ||
Chapter 7 System Design Considerations for Thermoelectric Energy Recovery | 156 | ||
7.1 Introduction | 156 | ||
7.1.1 The Potential for Waste Heat Recovery | 156 | ||
7.2 Modelling TEG Performance | 163 | ||
7.3 The Role of the Heat Exchanger in TEG Design | 165 | ||
7.4 Modelling a Thermoelectric Generator | 166 | ||
7.4.1 Defining the Structure of TEG | 167 | ||
7.4.2 Calculating Temperature Distributions | 169 | ||
7.4.3 Thermal Resistance Network in a CV | 170 | ||
7.5 Electrical-resistance Network of a TEG | 175 | ||
7.6 Model Structure | 176 | ||
7.7 Validation Strategy for Models | 178 | ||
7.8 Using the Model to Optimize TEM Geometry | 180 | ||
7.8.1 Geometric Factors | 180 | ||
7.8.2 Influence of Geometric Parameters on Maximum Power | 181 | ||
7.8.3 Influence of Operating Environments on Optimal Geometric Parameters | 183 | ||
7.8.4 Three-dimensional Figures to Identify the Optimal Geometry Parameters | 183 | ||
7.9 Selecting and Evaluating Heat Exchange Designs for TEG Applications | 183 | ||
7.9.1 Identifying the Design Parameters of a Plate Fin Heat Exchanger | 183 | ||
7.9.2 Comparing Heat Exchange Architectures | 189 | ||
7.10 An Example of a Family of Heat Exchangers | 193 | ||
7.11 Observations on TEG Design | 198 | ||
7.12 Concluding Remarks | 199 | ||
Appendix A: Exhaust Gas Properties Employed in the Simulation Model | 200 | ||
Appendix B: Properties of the Simulated Thermoelectric Module | 201 | ||
Acknowledgments | 201 | ||
References | 202 | ||
Chapter 8 Electrodeposition of Thermoelectric Materials | 204 | ||
8.1 Introduction | 204 | ||
8.1.1 Electrodeposition of Nanostructured Materials | 204 | ||
8.1.2 Recent Advances in the Electrodeposition of Thermoelectric Materials | 211 | ||
8.2 Experimental | 215 | ||
8.3 Results and Discussion | 217 | ||
8.3.1 Sodium Lignosulfonate as an Additive in the Electrodeposition of Bismuth Telluride | 217 | ||
8.3.2 Electrodeposition of n-type Copper-doped Bismuth Tellurium Selenide | 222 | ||
8.4 Conclusions | 226 | ||
Acknowledgments | 226 | ||
References | 226 | ||
Chapter 9 Automotive Power Harvesting/Thermoelectric Applications | 230 | ||
9.1 Why are Thermoelectric Devices Suitable for Automotive Applications? | 230 | ||
9.2 Automotive TEG Systems | 232 | ||
9.3 Challenges/Trade-offs in Automotive TEGs | 235 | ||
9.3.1 Thermoelectric Modules Packaging | 235 | ||
9.3.2 TEG Hot and Cold Heat Exchangers | 236 | ||
9.3.3 Exhaust Gas Flow Control (Valves and Sensors) | 240 | ||
9.3.4 TEG Overall Mechanical Packaging | 241 | ||
9.3.5 TEG Electrical Output Control Measurement and Control | 244 | ||
9.4 Failure of Thermoelectric Modules in Automotive Applications | 244 | ||
9.4.1 Ceramic Failures | 246 | ||
9.4.2 Pellet Failures | 247 | ||
9.4.3 Interconnect and Joint Failures | 247 | ||
9.4.4 Failure Modelling | 247 | ||
9.4.5 Failure Summary | 250 | ||
References | 251 | ||
Subject Index | 252 |