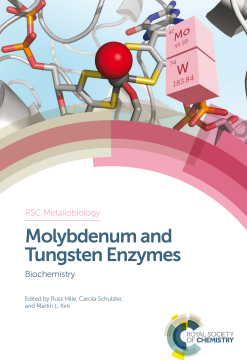
Additional Information
Book Details
Abstract
There has been enormous progress in our understanding of molybdenum and tungsten enzymes and relevant inorganic complexes of molybdenum and tungsten over the past twenty years. This set of three books provides a timely and comprehensive overview of the field and documents the latest research.
The first volume in the set focusses on the enzymes themselves, and discusses active sites and substrate channels of relevant proteins. The book begins with an introductory overview of the whole field. Chapters are contributed by world leaders and topics covered include pyranopterin cofactor biosynthesis and incorporation of the mature cofactor into apoprotein. Subsequent volumes cover the bioinorganic chemistry relevant to these enzymes and the full range of spectroscopic and theoretical methods that are used to investigate their physical and electronic structure and function.
This text will be a valuable reference to workers both inside and outside the field, including graduate students and young investigators interested in developing new research programs in this area.
This book is edited by Professor Russ Hille (University of California, Riverside), Professor Carola Schulzke (University of Greifswald) and Professor Martin Kirk (University of New Mexico) who have over 275 publications ad over 65 years of experience between them in the field. Their complementary expertise in molybdenum containing enzymes, bioinorganic chemistry and the physical inorganic chemistry of molybdenum complexes and enzymes, respectively, allow the authoritative and comprehensive coverage demonstrated in this book.
Table of Contents
Section Title | Page | Action | Price |
---|---|---|---|
Cover | Cover | ||
Molybdenum and Tungsten Enzymes Biochemistry | i | ||
Preface | v | ||
Dedication | vii | ||
Contents | ix | ||
Chapter 1 - Molybdenum and Tungsten-Containing Enzymes: An Overview | 1 | ||
1.1 Introduction | 1 | ||
1.2 Living with Molybdenum and Tungsten | 2 | ||
1.2.1 The Nitrogen-to-Molybdenum Bio-to-Inorganic Bridge Hypothesis | 6 | ||
1.3 Chemistry Relevant to Molybdenum and Tungsten Biochemistry | 9 | ||
1.4 Molybdenum- and Tungsten-Containing Enzymes | 15 | ||
1.4.1 The Xanthine Oxidase Family | 17 | ||
1.4.2 The Sulfite Oxidase Family | 25 | ||
1.4.3 The Dimethylsulfoxide Reductase Family | 32 | ||
1.4.4 The Tungstoenzymes Family | 43 | ||
1.4.5 The Nitrogenases | 49 | ||
1.4.6 A Novel Heterometallic Cluster Containing Molybdenum Found in Biology | 52 | ||
1.5 Outlook | 52 | ||
Abbreviations | 53 | ||
Acknowledgements | 54 | ||
References | 54 | ||
Chapter 2 - Abundance, Ubiquity and Evolution of Molybdoenzymes | 81 | ||
2.1 Introduction | 81 | ||
2.2 Molybdate Uptake and Molybdenum Cofactor Biosynthesis | 83 | ||
2.3 Classification of Molybdoenzymes | 85 | ||
2.4 Occurrence and Evolution of Molybdenum Utilization and Molybdoenzymes | 88 | ||
2.4.1 Occurrence of Molybdenum Transport and Moco Biosynthesis Pathway Genes | 88 | ||
2.4.2 Distribution and Phylogeny of Molybdoenzymes | 90 | ||
2.4.3 Factors that May Affect Evolution of Mo Utilization and Molybdoenzymes | 92 | ||
2.5 Concluding Remarks | 93 | ||
Acknowledgements | 94 | ||
References | 94 | ||
Chapter 3 - Molybdenum Cofactor Biosynthesis | 100 | ||
3.1 Introduction | 100 | ||
3.2 Formation of cPMP from 5′GTP | 104 | ||
3.3 Formation of MPT by Sulfur Insertion into cPMP | 105 | ||
3.4 Insertion of Molybdate into MPT | 108 | ||
3.5 Further Modification of Moco | 109 | ||
3.5.1 Formation of bis-MGD | 109 | ||
3.5.2 The Formation of the MCD Cofactor | 109 | ||
3.5.3 Moco Sulfuration in Eukaryotes | 110 | ||
3.6 Trafficking of Moco in the Cell | 110 | ||
3.7 Conclusions | 111 | ||
Acknowledgements | 111 | ||
References | 111 | ||
Chapter 4 - Bacterial Molybdoenzymes: Chaperones, Assembly and Insertion | 117 | ||
4.1 Introduction | 117 | ||
4.2 The Essential Role of Dedicated Chaperones for Molybdoenzyme Assembly | 118 | ||
4.2.1 Chaperones are Required for the Biogenesis of Cognate Molybdoenzymes | 118 | ||
4.2.2 Structural Constraints of Molybdoenzymes | 120 | ||
4.2.3 The Identification of Chaperones Dedicated to Specific Molybdoenzymes | 120 | ||
4.3 The TorD Family of Moco-Binding Chaperones | 122 | ||
4.3.1 Subfamily Organization of the TorD-Like Chaperones | 122 | ||
4.3.2 Structural Features of TorD-Like Chaperones | 124 | ||
4.3.3 Mechanism of Molybdoenzyme Maturation Dependent on TorD-Like Chaperones | 125 | ||
4.3.3.1 Maturation of TorA, a Soluble Monomeric Molybdoenzyme | 125 | ||
4.3.3.2 Maturation of Complex Membrane-Associated Molybdoenzymes | 127 | ||
4.3.3.3 bis-MGD Enzymes Can Acquire Other Accessory Proteins | 128 | ||
4.3.3.4 Systems Without a Dedicated Chaperone | 129 | ||
4.4 The Maturation of Formate Dehydrogenases | 129 | ||
4.5 The Step of Moco Sulfuration for Enzymes of the Xanthine Oxidase Family | 133 | ||
4.6 Conclusions | 136 | ||
Funding | 136 | ||
Acknowledgements | 136 | ||
References | 137 | ||
Chapter 5 - The Prokaryotic Mo/W-bisPGD Enzymes Family | 143 | ||
5.1 Introduction | 143 | ||
5.2 Reactivity and Substrate Specificity | 146 | ||
5.2.1 Role of the Mo/W Ligands | 149 | ||
5.2.2 Role of Amino Acids in the Immediate Environment of the Mo/W Atom | 151 | ||
5.2.3 Role of the Pterins | 152 | ||
5.2.4 What Can We Learn from Phylogenetic Analysis of the Mo-bisPGD Superfamily | 153 | ||
5.3 Molecular Variation of the Mo/W-bisPGD Enzymes | 155 | ||
5.3.1 The Catalytic Subunit | 155 | ||
5.3.2 The Electron Transfer Subunit | 158 | ||
5.3.2.1 Archetypal Subunit | 158 | ||
5.3.2.2 “Exotic” Subunits | 159 | ||
5.3.3 The Electron Entry/Exit Subunit | 160 | ||
5.3.3.1 b-Type Cytochromes | 160 | ||
5.3.3.2 The NrfD-Type Subunits | 161 | ||
5.3.3.3 c-Type Cytochromes | 161 | ||
5.3.3.4 The HydA Subunit in the Hydrogen-Dependent Carbon Dioxide Reductase System | 162 | ||
5.3.3.5 The NapGH Component | 162 | ||
5.4 Metabolic Chains Involving Mo/W-bisPGD Enzymes | 163 | ||
5.4.1 Enzymes Involved in the Nitrogen Cycle | 164 | ||
5.4.1.1 Nas and Nitrogen Assimilation | 165 | ||
5.4.1.2 Nar and Nitrate Respiration | 166 | ||
nNar and cNar.The first step of the bacterial denitrification pathway, catalyzed by the cytoplasmically oriented membrane-bound ... | 166 | ||
pNar.Several archaeal denitrifiers (e.g. several Haloarchaea sp.244–247) possess a Nar complex that shows two main differences a... | 169 | ||
5.4.1.3 Nap and Respiration or Dissipation of Excess Reductant | 170 | ||
5.4.1.4 Nxr and Nitrification or Anammox | 171 | ||
5.4.2 Enzymes Involved in the Sulfur Cycle | 172 | ||
5.4.3 Enzymes Involved in the Carbon Cycle | 174 | ||
5.5 Concluding Remarks | 176 | ||
Acknowledgements | 177 | ||
References | 177 | ||
Chapter 6 - Enzymes of the Xanthine Oxidase Family | 192 | ||
6.1 Introduction | 192 | ||
6.2 An Overview of Enzymes from the Xanthine Oxidase Family | 195 | ||
6.3 Xanthine Oxidoreductases from Eukaryotes and Bacteria | 200 | ||
6.3.1 The Crystal Structure of Bovine XOR | 202 | ||
6.3.2 The Xanthine Oxidase/Dehydrogenase Enigma | 202 | ||
6.3.3 The Physiological Role of Xanthine Oxidoreductase in Mammals | 205 | ||
6.3.4 The Bacterial XDH from Rhodobacter capsulatus | 206 | ||
6.3.5 The Mechanism of Substrate Conversion at the XOR Active Site | 208 | ||
6.3.6 Medical Relevance of XOR | 211 | ||
6.4 Aldehyde Oxidases | 213 | ||
6.4.1 The Mammalian Aldehyde Oxidases | 214 | ||
6.4.1.1 Gene Structure and Expression | 214 | ||
6.4.1.2 Substrates of Mammalian Aldehyde Oxidases | 216 | ||
6.4.1.3 Single Nucleotide Polymorphisms | 217 | ||
6.4.1.4 The Crystal Structure of mAOX3 | 217 | ||
6.4.1.5 Catalytic Mechanism | 219 | ||
6.4.1.6 The FAD Site | 221 | ||
6.4.2 Bacterial Aldehyde Oxidoreductases | 222 | ||
6.4.2.1 The Aldehyde Oxidoreductase MOP from Desulfovibrio gigas | 222 | ||
6.4.2.2 Quinoline 2-Oxidoreductase from Pseudomonas putida 86 | 224 | ||
6.4.3 Unusual Bacterial Enzymes of the XO Family with Unique Features | 226 | ||
6.4.3.1 Reversing the Reaction Mechanism in 4-Hydroxybenzoyl-CoA-Reductase | 226 | ||
6.4.3.2 A Selenide Ligand at the Active Site of Nicotinate Dehydrogenase | 227 | ||
6.4.3.3 The Only Binuclear Molybdenum Enzyme: Copper and Molybdenum at the Active Site of CODH | 229 | ||
6.5 Conclusions | 231 | ||
Acknowledgements | 231 | ||
References | 232 | ||
Chapter 7 - The Sulfite Oxidase Family of Molybdenum Enzymes | 240 | ||
7.1 Introduction | 240 | ||
7.2 Phylogenetic Structure of the SO Enzyme Family | 241 | ||
7.3 SO Family Enzymes from Different Types of Organisms | 246 | ||
7.3.1 SO Family Enzymes from Bacteria | 246 | ||
7.3.1.1 The cd_02107 Group: YedY and Related Enzymes | 246 | ||
7.3.1.2 The cd_02108 Group of Enzymes | 247 | ||
7.3.1.3 SO Family Proteins from Archaea and Proteobacteria (cd_02109) | 248 | ||
7.3.1.4 The Bacterial Enzymes of the “Moco-dimer” Group – Bacterial Sulfite and Sulfur Dehydrogenases | 249 | ||
7.3.1.5 The Bacterial SoxCD Proteins (cd_02113) | 250 | ||
7.3.1.6 The Bacterial “SorA”-type Sulfite Dehydrogenases (cd_02114) | 251 | ||
7.3.1.7 Other Bacterial Sulfite Oxidizing Enzymes (cd_02110) | 252 | ||
7.3.2 SO Family Enzymes from Vertebrates | 255 | ||
7.3.2.1 Maturation of Mammalian SO | 256 | ||
7.3.2.2 Catalytic Cycle in Vertebrate SO | 256 | ||
7.3.2.3 SO Deficiency | 258 | ||
7.3.3 SO Family Enzymes from Plants | 261 | ||
7.3.3.1 Nitrate Reductase | 261 | ||
7.3.3.2 SO in Plants | 262 | ||
7.4 General Aspects of Catalysis in SO Family Enzymes | 263 | ||
7.4.1 Sulfite Oxidizing Enzymes | 263 | ||
7.4.2 Nitrite Reduction by SO and Other Mo Enzymes | 266 | ||
7.5 Concluding Remarks | 267 | ||
Acknowledgements | 268 | ||
References | 268 | ||
Chapter 8 - Nitrogenase Mechanism: Electron and Proton Accumulation and N2 Reduction | 274 | ||
8.1 Introduction | 274 | ||
8.2 Electron Transfer and ATP Hydrolysis in Nitrogenase | 276 | ||
8.2.1 Docking of Fe Protein to the MoFe Protein | 276 | ||
8.2.2 Electron Transfer Events | 277 | ||
8.2.3 ATP and Nitrogenase | 279 | ||
8.2.4 Negative Cooperativity in the Electron Transfer/ATP Hydrolysis Cycle | 281 | ||
8.3 On the Mechanism of N2 Reduction | 282 | ||
8.3.1 E4: The “Janus Intermediate” | 284 | ||
8.3.2 “Dueling” N2 Reduction Pathways | 285 | ||
8.3.3 Nitrite and Hydroxylamine as Nitrogenase Substrates: Mechanistic Implications for the Pathway of N2 Reduction | 287 | ||
8.3.4 Mechanistic Convergence and its Implications | 287 | ||
8.3.5 Evolution of One H2 per N2 Reduced in Nitrogen Fixation is Obligatory | 289 | ||
8.3.5.1 Hydride Protonation (hp) Mechanism | 290 | ||
8.3.5.2 Reductive Elimination (re) Mechanism | 290 | ||
8.3.6 First Experimental Test of the re Mechanism | 291 | ||
8.3.7 Second Experimental Test of re: Identification of the Key E4(2N2H) Catalytic Intermediate | 291 | ||
8.4 Summary and Prospects | 293 | ||
Acknowledgements | 294 | ||
References | 294 | ||
Chapter 9 - Biosynthesis of the M-Cluster of Mo-Nitrogenase | 297 | ||
9.1 Introduction | 297 | ||
9.2 M-Cluster Assembly | 299 | ||
9.2.1 Overview | 299 | ||
9.2.2 Formation of a Precursor to the M-Cluster | 299 | ||
9.2.3 Maturation of the Precursor into an M-Cluster | 306 | ||
Acknowledgements | 310 | ||
References | 310 | ||
Chapter 10 - Tungsten-Containing Enzymes | 313 | ||
10.1 Introduction | 313 | ||
10.2 General Properties of Tungstoenzymes | 314 | ||
10.2.1 Tungsten-Based Enzymology: State of the Art | 314 | ||
10.2.2 Tungsto-Pterin Prosthetic-Group: Nomenclature Issues | 315 | ||
10.2.3 Tungstoenzymes Come in Two Families | 317 | ||
10.2.4 Reactions Catalyzed by Tungstoenzymes | 319 | ||
10.3 Specific Properties of AOR-Family Tungstoenzymes | 321 | ||
10.3.1 Aldehyde Oxidoreductases | 321 | ||
10.3.1.1 Substrate Specificity | 322 | ||
10.3.1.2 Reaction Mechanism | 323 | ||
10.3.2 Benzoyl-CoA Reductase | 326 | ||
10.4 Specific Properties of DMSOR-Family Tungstoenzyme | 328 | ||
10.4.1 Formate Dehydrogenase | 328 | ||
10.4.2 Formylmethanofuran Dehydrogenase | 329 | ||
10.4.3 DMSO Reductase | 331 | ||
10.4.4 Nitrate Reductase | 332 | ||
10.4.5 Acetylene Hydratase | 332 | ||
10.5 Tungsten Metallomics | 333 | ||
10.6 Why Tungsten | 335 | ||
References | 337 | ||
Subject Index | 343 |