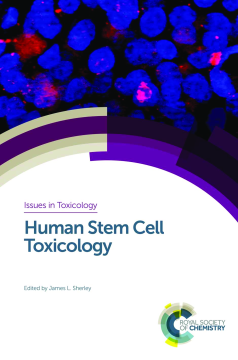
Additional Information
Book Details
Abstract
Toxicity against tissue stem cells (TSCs) is a major problem in drug development and environmental health science. Despite their essential function in all human cellular tissues, the nature of tissue stem cells is not fully understood. The small fraction of stem cells in tissues and the lack of specific biomarkers for their quantification present a formidable challenge to developing tools for their study and assays that can identify stem cell-toxic agents.
Human Stem Cell Toxicology reveals TSC toxicity as a biomedical reality that is now well under siege by newly emerging ideas and technologies, despite these challenges. Chapters consider stem cell toxicity by environmental agents, pharmaceutical drug candidates, and marketed therapeutic medicines with adverse side effects. New insights to cellular, molecular, biochemical, and chemical mechanisms of human tissue stem cell toxicity are brought together. Experimental and theoretical treatments are of specific topics, including approaches to monitoring TSC function, newly discovered TSC types and TSC toxicity resistance mechanisms, are covered by expert authors.
This book informs and champions the continued development of innovative technologies to predict the TSC toxicity of compounds before their use, whether in patients or the environment, by addressing emerging new cell-based approaches and concepts for technical innovation. This publication will be a useful reference for postgraduate students and researchers working in toxicology, pharmaceutical science, tissue cell biology and stem cell biology.
James L. Sherley, M.D., Ph.D. is the founder and director of Asymmetrex, LLC. Launched in 2009 during his tenure as a Senior Scientist at the Boston Biomedical Research Institute (BBRI) from 2007 to 2013, originally as the Adult Stem Cell Technology Center, Asymmetrex has the mission of advancing tissue stem cell-based technologies to commercial development for biomedical and research applications. Dr Sherley joined the faculty of BBRI as a senior member of its research programs in Regenerative Biology and Cancer Biology to lead a new focus in developing adult stem cell-based technologies for advancing cellular medicine. Dr Sherley is a 1980 graduate of Harvard College, with a B.A. degree in biology; and he completed joint M.D. and Ph.D. degrees at the Johns Hopkins University School of Medicine in 1988. After post-doctoral studies in cancer cell molecular biology at Princeton University, he joined the Fox Chase Cancer Center in Philadelphia as a principal investigator in 1991. In 1998, he joined the faculty of the future Department of Biological Engineering at the Massachusetts Institute of Technology, where he undertook research and teaching in the areas of cancer cell molecular biology, tissue stem cell bioengineering, and environmental health science until moving to BBRI in 2007. Dr Sherley’s awards include 1993 Pew Biomedical Research Scholar, 2003 Ellison Medical Foundation Senior Scholar in Aging Research, and 2006 NIH Director’s Pioneer Award. He is also "PGP-10" in the Personal Genome Project at Harvard Medical School.
Table of Contents
Section Title | Page | Action | Price |
---|---|---|---|
Cover | Cover | ||
Contents | vii | ||
Chapter 1 Addressing Challenges to Progress in Human Stem Cell Toxicology Concepts and Practice | 1 | ||
1.1 Filling in the Stem Cell Gap in Human Toxicology | 1 | ||
1.2 Historical Impact of the Hierarchical, Anatomical, Sub-disciplinary Structure of Toxicological Sciences | 2 | ||
1.3 Human Stem Cell Toxicology as a Stem Cell Exact Science | 3 | ||
1.4 Health and Medical Applications for Human Stem Cell Toxicological Sciences | 5 | ||
1.5 Introducing the Future Diverse Impacts of Human Stem Cell Toxicology | 6 | ||
Acknowledgments | 7 | ||
References | 7 | ||
Chapter 2 Alternative Methods in Haematopoietic Stem Cell Toxicology | 9 | ||
2.1 Introduction | 9 | ||
2.2 Haematopoietic Stem Cell Toxicity or Hematotoxicity | 11 | ||
2.2.1 Sources of Haematopoietic Stem Cell Toxicity | 12 | ||
2.2.2 Importance of Studying Haematopoietic Stem Cell Toxicity | 13 | ||
2.2.3 Haematopoietic Stem Cell Toxicity in Drug Development | 14 | ||
2.3 Colonogenic Assays as Predictors of Haematopoietic Stem Cell Toxicity | 14 | ||
2.3.1 CFU-GM Colonogenic Assay | 15 | ||
2.3.2 CFU-Mk Colonogenic Assay | 16 | ||
2.3.3 BFU-E Colonogenic Assay | 18 | ||
2.3.4 Lymphoid Lineage Based Colonogenic Assays | 18 | ||
2.4 Conclusions | 21 | ||
Acknowledgments | 22 | ||
References | 22 | ||
Chapter 3 High-throughput Screening of Toxic Chemicals on Neural Stem Cells | 31 | ||
3.1 Neural Stem Cells | 31 | ||
3.2 Toxic Chemicals in the Environment | 32 | ||
3.3 Mechanisms of Neural Stem Cell Toxicity | 33 | ||
3.3.1 Ion Channel Blocking | 34 | ||
3.3.2 Drug Metabolism Effects | 37 | ||
3.3.3 Oxidative Stress | 40 | ||
3.3.4 DNA/RNA Denaturation | 42 | ||
3.3.5 Membrane Compromise | 42 | ||
3.3.6 Other Mechanisms of Neurotoxicity | 43 | ||
3.4 NSC Differentiation | 43 | ||
3.5 Conventional In vitro Assays for Toxicity Screening against Neural Stem Cells | 45 | ||
3.5.1 Well Plate Assays | 45 | ||
3.5.2 Cellular Microarray Assays | 47 | ||
3.5.3 Microfluidic Assays | 49 | ||
3.5.4 Other Assays | 51 | ||
3.6 Challenges of Conventional In vitro Approaches in Neurotoxicity Screening | 51 | ||
3.7 Conclusions and Future Directions | 52 | ||
Acknowledgments | 53 | ||
References | 53 | ||
Chapter 4 The Role of Catecholamines in Stem Cell Mobilisation | 64 | ||
4.1 Introduction | 64 | ||
4.2 Catecholamines | 64 | ||
4.3 Catecholamines and Stem Cell Mobilisation | 67 | ||
4.3.1 Endothelial Progenitor Cells | 70 | ||
4.3.2 Mesenchymal Stem Cells | 71 | ||
4.3.3 Catecholamines and Stem Cell Biology | 72 | ||
4.4 Consequences of Catecholamine-modulating Agents for Stem Cell Toxicity | 73 | ||
4.4.1 Other Considerations | 78 | ||
4.5 Concluding Comments | 80 | ||
References | 81 | ||
Chapter 5 Toxicological Risk Assessment – Proposed Assay Platform Using Stem and Progenitor Cell Differentiation in Response to Environmental Toxicants | 94 | ||
5.1 Introduction | 94 | ||
5.1.1 Toxicity | 95 | ||
5.1.2 Environmental Toxicology | 95 | ||
5.1.3 Predictive Toxicology | 95 | ||
5.1.4 Automated High Content Imaging and High Throughput, or High Content, Screening | 96 | ||
5.1.5 Risk Assessment | 97 | ||
5.1.6 Components of Risk Assessment | 97 | ||
5.2 Environmental Toxicological Risk Assessment Employing an Assay Platform That Uses Stem and Progenitor Cell Differentiation | 98 | ||
5.2.1 Endothelial Colony Forming Cells (ECFCs) | 99 | ||
5.2.2 ECFCs are Sensitive to Low-dose Ionizing Radiation (LDIR) | 100 | ||
5.2.3 Individual ECFC Cultures Exhibit Donor-related LDIR Responses | 100 | ||
5.2.4 The Profiling of Intracellular Signal Transduction Pathways Provides an Insight into the Mechanism of LDIR Toxicity | 101 | ||
5.3 Current State of ECF Platform Development | 102 | ||
5.3.1 Impedance-based Analysis of ECFC Viability after Exposure to Environmental Toxicants | 102 | ||
5.3.2 ECFCs Exhibit Lot-to-lot Variability in Toxicant Response | 105 | ||
5.3.3 Development of a Novel ROS Assay Using ECFCs | 105 | ||
5.3.4 Density-dependent ROS Levels in Cultured ECFCs | 106 | ||
5.3.5 Signal Transduction Assays in Toxicant-treated ECFCs | 108 | ||
5.4 Bioanalytical Method Validation | 110 | ||
5.4.1 Development of a Quantitative High Content Imaging (QHCA) Platform Using ECFCs | 112 | ||
5.4.2 Optimize Culture Conditions for High-throughput Screening | 112 | ||
5.4.3 Initiate Translation of Assay to 384-Well Plates | 113 | ||
5.4.4 Incorporation of Automation to Increase Throughput | 114 | ||
5.4.5 Validation of the ECFC QHCA | 114 | ||
5.4.6 Determining the Z' factor of the Cell Death Assays Using Positive and Negative Controls | 115 | ||
5.4.7 Assessing Sources of Assay Variability Including Manual Pipetting, Plating and Edge Effects | 116 | ||
5.4.8 Determining Day-to-day Variability of EC50 for Each Assay | 116 | ||
5.4.9 Determining Significant Biological Replicate Power | 117 | ||
5.4.10 Perform the High-throughput Assay Using Compounds from the ToxCastTM Phase I Library | 117 | ||
5.4.11 Incorporation of the Toxicant-induced ECFC Differentiation Assays into the QHCA Screen | 118 | ||
5.4.12 Establish a Repository of ECFCs from Various Donors | 120 | ||
5.5 Final Thoughts | 121 | ||
References | 121 | ||
Chapter 6 Current Developments in the Use of Human Stem Cell Derived Cardiomyocytes to Examine Drug-induced Cardiotoxicity | 124 | ||
6.1 Introduction | 124 | ||
6.2 Constraints Due to Species Differences | 125 | ||
6.3 Stem Cells and iPSC-CMs | 127 | ||
6.4 Limitations with Stem Cells | 128 | ||
6.5 Stem Cells in Cardiovascular Safety Pharmacology | 129 | ||
6.6 Disease Models Based on iPSC-CMs | 135 | ||
6.7 Generation of iPSC-CMs – Considerations on Differentiation, Maturity, Heterogeneity and Purification Protocols | 137 | ||
6.7.1 Differentiation | 137 | ||
6.7.2 Maturity | 138 | ||
6.7.3 Heterogeneity | 138 | ||
6.7.4 Purification | 138 | ||
6.8 Use of iPSC-CMs in Phenotypic Assays | 139 | ||
6.9 Assay Technologies Incorporating iPSC-CMs and hESC-CMs | 140 | ||
6.9.1 Manual Patch Clamp | 140 | ||
6.9.2 Automated Patch Clamp | 142 | ||
6.9.3 MEA (Microelectrode Array) | 142 | ||
6.10 CiPA: Comprehensive In vitro Proarrhythmia Assay | 144 | ||
6.11 Conclusion | 146 | ||
References | 147 | ||
Chapter 7 Pesticides and Hematopoietic Stem Cells | 160 | ||
7.1 Pesticide Toxicity-induced Disorders of Hematopoietic System | 160 | ||
7.1.1 Hematopoietic System and Hematotoxic Pesticides | 160 | ||
7.1.2 Pesticide-induced Aplastic Anemia: A Rare but Severe Hematopathology due to Stem Cell Failure | 162 | ||
7.1.3 Assessment of Hematotoxicity | 166 | ||
7.2 Pesticide Toxicity on Hematopoietic Stem Cells and their Microenvironment | 167 | ||
7.2.1 Oxidative Stress Induction | 167 | ||
7.2.2 Apoptosis Induction | 167 | ||
7.2.3 Alteration of Developmental Signaling Pathways | 168 | ||
7.3 Experimental Medicine Against Pesticide Toxicity-induced Hematopoietic Failure | 170 | ||
7.4 Future Direction | 171 | ||
References | 171 | ||
Chapter 8 Epigenetic Impact of Stem Cell Toxicants | 178 | ||
8.1 Introduction | 178 | ||
8.2 Epigenetic Regulation of Stem Cells | 181 | ||
8.3 Stem Cell Toxicants as Modulators of Epigenetic Programming | 182 | ||
8.3.1 Heavy Metals | 183 | ||
8.3.2 Pharmaceuticals | 185 | ||
8.4 Conclusion | 187 | ||
Acknowledgments | 187 | ||
References | 187 | ||
Chapter 9 Metakaryotic Cancer Stem Cells are Constitutively Resistant to X-Rays and Chemotherapeutic Agents, but Sensitive to Many Common Drugs | 196 | ||
9.1 Introduction | 197 | ||
9.1.1 Introduction to Metakaryotic Biology | 197 | ||
9.2 Materials and Methods | 200 | ||
9.2.1 Methods for Studies of Metakaryotic Cancer Stem Cells In vivo and In vitro | 200 | ||
9.3 Results | 204 | ||
9.3.1 Observations in Tumors after Radiation Therapy and Chemotherapy | 204 | ||
9.3.2 Observations in Cell Cultures | 206 | ||
9.4 Discussion | 233 | ||
9.4.1 Stem Cells in Human Tumors and Tumor-derived Cell Lines are Amitotic, Metakaryotic Cells | 233 | ||
9.4.2 Assays that Recognize and Measure the Toxicity of Radiation and Chemicals to Metakaryotic Stem Cells | 235 | ||
9.4.3 Growth and Development of Turnover Units in HT-29 Cultures | 235 | ||
9.4.4 Metakaryotic Stem Cells are Resistant to Doses of X-Rays and Drug Classes Commonly in Use for Cancer Chemotherapy | 237 | ||
9.4.5 Metakaryotic Stem Cells are Sensitive to Many Drugs in Common Use: Verapamil, Metformin, NSAIDS and Antibiotics | 237 | ||
9.4.6 Hypotheses about Metakaryocidal Mechanisms, e.g. Inhibition of Mitochondrial Function | 239 | ||
9.4.7 Other Potential Targets for Metakaryocides: Genome Replication and Segregation | 241 | ||
9.4.8 Translation into Clinical Practice | 242 | ||
9.4.9 Potential Use of Metakaryocides in Prevention of Cancers and Other Clonal Diseases | 244 | ||
9.4.10 Other Considerations | 244 | ||
Acknowledgments | 246 | ||
References | 246 | ||
Chapter 10 Distributed Stem Cell Kinetotoxicity: A New Concept to Account for the Human Carcinogenicity of Non-genotoxic Environmental Toxicants | 250 | ||
10.1 Introduction | 250 | ||
10.2 Results and Discussion | 253 | ||
10.2.1 Development of a High-throughput Cell Kinetics Assay for Kinetotoxicity | 253 | ||
10.2.2 Use of High-throughput Screening to Detect Benzene and Hydroquinone as Kinetotoxic Agents | 256 | ||
10.2.3 Confirmation Studies for Benzene and Hydroquinone Kinetotoxicity | 262 | ||
10.2.4 Validation of Benzene and Hydroquinone Kinetotoxicity with DSCs | 265 | ||
10.2.5 Use of Microarray Analyses to Discover a Potential Molecular Biomarker for Kinetotoxicity | 268 | ||
10.3 Conclusions and Closing Thoughts | 270 | ||
10.3.1 Kinetotoxicity, An Extended Concept in Human Stem Cell Toxicology for Carcinogens | 270 | ||
10.3.2 Development of a High-throughput Screen for Kinetotoxic Agents | 271 | ||
10.3.3 Mechanisms of Kinetotoxicity by Benzene and Hydroquinone | 272 | ||
10.3.4 The DSC Specification Problem in Human Stem Cell Toxicology | 273 | ||
10.3.5 Looking Forward | 274 | ||
10.4 Materials and Methods | 275 | ||
10.4.1 Cells | 275 | ||
10.4.2 Chemicals | 275 | ||
10.4.3 Development of the High-throughput Microplate Assay for Kinetotoxicity | 276 | ||
10.4.4 Assays for Self-renewal Kinetics Pattern Determination | 276 | ||
10.4.5 Microarray Analyses | 276 | ||
Acknowledgments | 276 | ||
References | 277 | ||
Chapter 11 Cancer Stem Cells as Therapeutic Targets | 280 | ||
11.1 Introduction | 280 | ||
11.2 CSC Markers and Therapeutic Targets | 282 | ||
11.3 Signal Transduction in CSCs and Targeted Agents | 283 | ||
11.4 Asymmetric Cell Divisions: The Dilemma of Studies on CSCs | 284 | ||
11.5 Asymmetric Cell Divisions: Visualization of CSCs and Toxicology | 286 | ||
11.6 Asymmetric Cell Divisions; Potential Therapeutics Targeting CSCs | 289 | ||
11.7 Closing Remarks | 291 | ||
Acknowledgments | 291 | ||
References | 291 | ||
Subject Index | 295 |