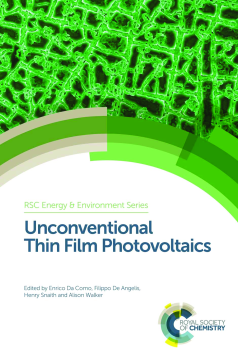
BOOK
Unconventional Thin Film Photovoltaics
Enrico Da Como | Filippo De Angelis | Henry Snaith | Alison Walker
(2016)
Additional Information
Book Details
Abstract
Covering both organic materials, where recent advances in the understanding of device physics is driving progress, and the newly emerging field of mixed halide perovskites, which are challenging the efficiencies of conventional thin film PV cells, this book provides a balanced overview of the experimental and theoretical aspects of these two classes of solar cell. The book explores both the experimental and theoretical aspects of these solar cell classes. Emphasis is placed on understanding the fundamental physics of the devices. The book also discusses modelling over many length scales, from nano to macro. The first book to cover perovskites, this is an important reference for industrialists and researchers working in energy technologies and materials.
Enrico Da Como is a Reader in the Department of Physics, University of Bath, UK. His research deals with the interaction of light with condensed matter.
Filippo De Angelis is Senior Researcher and Deputy Director at the CNR Institute of Molecular Sciences and Technologies, Italy. His research interests are mainly focussed on the computational modeling of hybrid/organic photovoltaics.
Henry Snaith is a Professor in the Department of Physics, University of Oxford, UK. His research focusses on developing the physics and technology behind low cost photovoltaic concepts.
Alison Walker is a Professor in the Department of Physics, University of Bath, UK. Her research covers the materials and physics of organic, inorganic and hybrid semiconductor devices.
Table of Contents
Section Title | Page | Action | Price |
---|---|---|---|
Cover | Cover | ||
Contents | ix | ||
Preface | v | ||
Chapter 1 High Efficiency Mesoscopic Organometal Halide Perovskite Solar Cells | 1 | ||
1.1 Introduction | 1 | ||
1.1.1 Emergence and Progress of Perovskite Solar Cells | 1 | ||
1.1.2 Role and Importance of the Organic Cation in Halide Perovskites: Phase Transitions, Ferroelectricity and Ion Migration | 6 | ||
1.2 Mesoscopic Perovskite Solar Cells | 10 | ||
1.2.1 Perovskite Dots and Extremely Thin Absorber Layers | 10 | ||
1.2.2 Perovskite Hybrids with Mesoporous and Nanostructured TiO2 | 15 | ||
1.3 Summary | 29 | ||
Acknowledgments | 29 | ||
References | 29 | ||
Chapter 2 Towards Optimum Solution-processed Planar Heterojunction Perovskite Solar Cells | 32 | ||
2.1 Introduction | 32 | ||
2.2 Towards Optimum Solution-processed Cells | 33 | ||
2.2.1 Basic Cell Structure | 33 | ||
2.2.2 Towards 100% Surface Coverage | 34 | ||
2.2.3 Contact Materials | 43 | ||
2.3 Outlook and Conclusions | 48 | ||
References | 51 | ||
Chapter 3 Characterization of Capacitance, Transport and Recombination Parameters in Hybrid Perovskite and Organic Solar Cells | 57 | ||
3.1 Introduction | 57 | ||
3.2 Dielectric Relaxation, Impedance and Capacitance Spectroscopy | 59 | ||
3.2.1 General Definitions and Concepts | 59 | ||
3.2.2 Dielectric Relaxation and Frequency Dispersion | 60 | ||
3.2.3 The Chemical Capacitance | 68 | ||
3.2.4 Contact Capacitances | 69 | ||
3.2.5 Recombination Parameters | 70 | ||
3.3 Capacitance in Organic Solar Cells | 72 | ||
3.3.1 Chemical Capacitance | 72 | ||
3.3.2 Mott-Schottky Analysis | 74 | ||
3.4 Capacitances in Hybrid Perovskite Solar Cells | 76 | ||
3.4.1 The Density of States | 76 | ||
3.4.2 Bulk Dielectric Constant | 77 | ||
3.4.3 Electronic Contact Capacitance: A Mott-Schottky Analysis | 79 | ||
3.4.4 Electrode Capacitance | 80 | ||
3.4.5 Dependence of Capacitance on Illumination | 81 | ||
3.5 Carrier Transport | 86 | ||
3.6 Recombination in Organic Solar Cells | 90 | ||
3.6.1 Recombination Mechanisms | 90 | ||
3.6.2 Determination of Recombination Kinetics | 91 | ||
3.7 Recombination in Perovskite Solar Cells | 93 | ||
3.7.1 Radiative Recombination Coefficient of MAPbI3 | 93 | ||
3.7.2 Radiative and Non-radiative Recombination in Perovskite Solar Cells | 95 | ||
3.8 Conclusions | 100 | ||
Acknowledgments | 101 | ||
References | 101 | ||
Chapter 4 Photophysics of Hybrid Perovskites | 107 | ||
4.1 Introduction | 107 | ||
4.2 Linear Absorption | 108 | ||
4.2.1 3D Semiconductor Absorption | 108 | ||
4.2.2 Bandgap | 110 | ||
4.2.3 Excitonic Absorption | 111 | ||
4.2.4 The Saha-Langmuir Equation | 120 | ||
4.3 Photoluminescence | 122 | ||
4.3.1 Spontaneous Emission | 122 | ||
4.3.2 Shockley-Read-Hall Recombination | 123 | ||
4.3.3 Auger Recombination | 124 | ||
4.3.4 Model for PL Dynamics in CH3NH3PbI3 | 125 | ||
4.4 Micro-structure and Optical Properties | 129 | ||
4.4.1 Bandgap and Photoluminescence | 130 | ||
4.4.2 Electron-Hole Screening: Pump-Probe Spectroscopy as a Probe | 132 | ||
4.5 Transport Properties | 135 | ||
4.5.1 Carrier Diffusion from Photoluminescence Decays | 136 | ||
4.5.2 Optical Pump–Terahertz Probe (OPTP) Experiments | 137 | ||
4.6 Summary and Outlook | 137 | ||
Acknowledgments | 138 | ||
References | 138 | ||
Chapter 5 The Role of Nanostructured Metal Oxides in Hybrid Solar Cells | 141 | ||
5.1 Introduction | 141 | ||
5.2 Fundamentals of Hybrid Solar Cells | 143 | ||
5.3 Transparent Electrodes and Blocking Layers | 149 | ||
5.4 Nanostructured Active Layers | 155 | ||
5.4.1 Physical Structuring | 155 | ||
5.4.2 Nanostructures for Light Manipulation | 162 | ||
5.4.3 Nanostructures for Energy Landscape Engineering | 165 | ||
5.5 Conclusions and Outlook | 170 | ||
Acknowledgments | 171 | ||
References | 171 | ||
Chapter 6 Structural, Electronic, and Optical Properties of Lead Halide Perovskites | 177 | ||
6.1 Introduction | 177 | ||
6.2 Crystal Structure | 178 | ||
6.3 Electronic Structure | 181 | ||
6.4 Optical Absorption | 184 | ||
6.5 Point Defect Properties | 186 | ||
6.6 Grain Boundaries and the Passivating Effect of Cl | 190 | ||
6.7 Conclusions and Outlook | 197 | ||
References | 197 | ||
Chapter 7 Electronic Properties of Metal Halide Perovskites | 202 | ||
7.1 Introduction to All-Inorganic (AIP) and Hybrid Organic (HOP) Metal Halide Perovskites | 202 | ||
7.2 A Pedestrian Guide to Symmetry Properties of the Reference Cubic Structure | 205 | ||
7.2.1 General Overview and Lattice Symmetries | 205 | ||
7.2.2 Localized and Collective Vibrations and Strain | 208 | ||
7.2.3 Localized Rotations and Collective Rotational Pseudospins | 210 | ||
7.2.4 Basic Optoelectronic Properties of the Metal Halide Lattice | 212 | ||
7.2.5 Importance of Spin–Orbit (SOC) Coupling | 214 | ||
7.3 Phase Transitions | 215 | ||
7.3.1 A Landau Theory for HOP with Linear and Linear-quadratic Order Parameter Couplings | 215 | ||
7.3.2 Electronic Properties: Brillouin Zone (BZ) Folding | 216 | ||
7.3.3 Electronic Properties: Lattice Strain and Octahedron Tilting | 217 | ||
7.3.4 Electronic Properties: Interplay of SOC and Loss of Inversion Symmetry | 218 | ||
7.3.5 Electronic Properties: Quantum Confinement | 219 | ||
7.4 Electronic Excitations | 222 | ||
7.4.1 Dielectric Properties of HOP and AIP | 222 | ||
7.4.2 From Wannier to Bound Excitons in HOP | 223 | ||
7.4.3 Free Carrier Coupling to Phonons and Molecular Rotations | 225 | ||
7.4.4 From Free Carriers to Heavy Polarons in HOP | 226 | ||
7.4.5 Many-body and Auger Effects in AIP and HOP | 226 | ||
7.5 Conclusions and Outlook | 228 | ||
Acknowledgments | 229 | ||
References | 229 | ||
Chapter 8 First Principles Modeling of Perovskite Solar Cells: Interplay of Structural, Electronic and Dynamical Effects | 234 | ||
8.1 Introduction | 234 | ||
8.2 Benchmarking the Computation Toolbox: Sn vs. Pb Perovskites | 235 | ||
8.3 Mixed Metal, Mixed Halide and Different Cations | 242 | ||
8.3.1 Mixed Sn/Pb Perovskites | 242 | ||
8.3.2 The Role of the A Cation | 249 | ||
8.3.3 Methylammonium Dynamics in the Perovskite Lattice | 260 | ||
8.3.4 The Problem of the Methylammonium Orientation | 260 | ||
8.3.5 The Effect of Methylammonium Orientation in Thin Films | 270 | ||
8.3.6 Conversion among Different Methylammonium Orientations | 272 | ||
8.3.7 Dynamics in Polar and Apolar Structures | 275 | ||
8.4 The Effect of Chlorine Doping: the Nature of the MAPbI3-xClx Perovskite | 278 | ||
8.4.1 Quantifying the Chlorine Doping Percentage | 278 | ||
8.4.2 Unravelling the Location of Chlorine in Perovskite Thin Films | 281 | ||
8.4.3 The Electronic Role of Interfacial Chlorine | 286 | ||
8.5 Conclusions and Outlook | 289 | ||
Acknowledgments | 290 | ||
References | 290 | ||
Chapter 9 Drift Diffusion Modelling of Charge Transport in Photovoltaic Devices | 297 | ||
9.1 Introduction | 297 | ||
9.2 Equilibrium Electron and Hole Distribution in a Semiconductor | 300 | ||
9.2.1 The Boltzmann Approximation | 301 | ||
9.2.2 The Intrinsic Carrier Density | 301 | ||
9.2.3 Doping | 301 | ||
9.3 Probabilistic and Drift Diffusion Models of Charge Transport | 302 | ||
9.3.1 Rate Equations for Particle Hopping on a Lattice | 303 | ||
9.3.2 A Probabilistic Approach to Particle Hopping | 304 | ||
9.3.3 Derivation of a Diffusion Equation for Particle Hopping | 305 | ||
9.3.4 Description of Particle Hopping on a Lattice in an Applied Potential | 306 | ||
9.3.5 Other Processes Giving Rise to Drift Diffusion Models | 307 | ||
9.4 Drift Diffusion Models of Charge Transport in Semiconductors | 307 | ||
9.4.1 Currents, Fluxes and Carrier Concentration | 309 | ||
9.4.2 Carrier Generation and Recombination | 311 | ||
9.4.3 The Full Equations | 313 | ||
9.4.4 A Simple One-dimensional Inorganic Solar Cell: The n-p Homojunction | 314 | ||
9.5 Shockley Equivalent Circuit Models of Photovoltaic Devices | 322 | ||
9.5.1 The Current-Voltage Curve of a Diode | 322 | ||
9.6 Inclusion of Defect-mediated Ion Motion | 325 | ||
9.7 Conclusions and Outlook | 329 | ||
Acknowledgments | 330 | ||
References | 330 | ||
Chapter 10 Small Molecule Organic Solar Cells | 332 | ||
10.1 Introduction | 332 | ||
10.2 Device Structures | 334 | ||
10.2.1 The p-i-n Structure | 334 | ||
10.2.2 The Built-in Voltage | 337 | ||
10.2.3 Doped Transport Layers | 345 | ||
10.3 High-efficiency Devices | 348 | ||
10.3.1 No Need for Ultra-high Mobilities? | 348 | ||
10.3.2 Charge Generation at the DCVnT:C60 Interface | 352 | ||
10.3.3 Correlating Charge Carrier Generation Yield with Charge Carrier Mobility | 353 | ||
10.3.4 Multi-junction Cells | 357 | ||
10.4 Conclusions | 360 | ||
References | 361 | ||
Chapter 11 Modeling Organic Solar Cells: What are the Challenges Ahead? | 367 | ||
11.1 Introduction | 367 | ||
11.2 Light Absorption and Exciton Dissociation: Review of Recent Modeling Work | 374 | ||
11.3 The Challenges Ahead: Towards the Prediction of CT Energies and Decay Dynamics | 383 | ||
11.4 Concluding Remarks | 387 | ||
Acknowledgments | 387 | ||
References | 388 | ||
Chapter 12 Molecular Dynamics Simulations and their Application to Thin-film Devices | 391 | ||
12.1 Introduction | 391 | ||
12.2 Force Fields | 394 | ||
12.3 Observables and Characterisation | 400 | ||
12.4 Thin Films and Interfaces | 410 | ||
Acknowledgments | 414 | ||
References | 414 | ||
Chapter 13 3D Simulations of Organic Solar Cells | 420 | ||
13.1 Introduction | 420 | ||
13.2 Fundamental Processes in Organic Solar Cells | 421 | ||
13.2.1 Generation of Free Charges | 422 | ||
13.2.2 Charge Transport | 422 | ||
13.2.3 Charge Recombination | 424 | ||
13.3 Operation of Drift-diffusion Simulations | 425 | ||
13.4 Operation of KMC Simulations | 428 | ||
13.5 Implementation | 430 | ||
13.6 Impact of Morphology on OPV Performance | 431 | ||
13.7 KMC for Other Types of Devices | 445 | ||
13.8 Comparing Drift-diffusion and KMC Simulations | 445 | ||
13.9 Outlook | 446 | ||
Acknowledgments | 447 | ||
References | 447 | ||
Chapter 14 Continuum-limit Modelling of Structure Evolution in Active Blends for Organic Solar Cells | 453 | ||
14.1 Introduction | 453 | ||
14.2 Experimental Observation of Liquid-phase Demixing in Polymer:PCBM Blends | 454 | ||
14.3 Continuum Modelling of Morphology Formation by Liquid-phase Demixing | 458 | ||
14.3.1 Free Energy | 460 | ||
14.3.2 Transport Dynamics | 461 | ||
14.3.3 Substrate Interaction | 463 | ||
14.3.4 Solvent Evaporation | 465 | ||
14.3.5 The Effect of Solvent Evaporation on Structure Size Evolution | 466 | ||
14.3.6 Polymer:PCBM Morphology Formation and Relation to OPV Device Performance | 470 | ||
14.4 Conclusions and Outlook | 474 | ||
Acknowledgments | 474 | ||
References | 474 | ||
Subject Index | 478 |