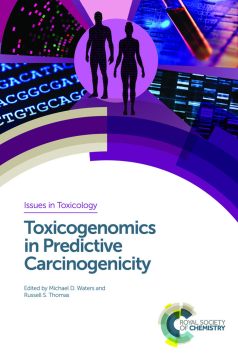
Additional Information
Book Details
Abstract
Research over the past decade has demonstrated that TGx methods of various types can be used to discriminate modes of mutagenesis as a function of dose. TGx can quickly inform safety evaluation regarding potential mechanisms of conventional outcomes and can provide essential dose-response information. This can then be used to ascertain the sequence of key events in a putative mode of action as may apply in quantitative cancer risk assessment. With the increasing complexity of research in mode of action investigations it is important to gain a better understand of approaches to data integration and health risk assessment. Furthermore, it is essential to consider how novel test systems and newer methods and approaches may be used in future to gain a better understanding of mechanisms.
Toxicogenomics in Predictive Carcinogenicity describes toxicogenomics methods in predictive carcinogenicity testing, mode of action and safety evaluation, and cancer risk assessment. It illustrates these methods using case studies that have yielded significant new information on compounds and classes of compounds that have proven difficult to evaluate using conventional methods alone. This book additionally covers current and potential toxicogenomic research using stem cells as well as new bioinformatics methods for drug discovery and environmental toxicology.
This publication is an indispensable tool for postgraduates, academics and industrialists working in biochemistry, genomics, carcinogenesis, pathology, pharmaceuticals, food technology, bioinformatics, risk assessment and environmental toxicology.
Dr Michael D. Waters is currently a Consultant with Integrated Laboratory Systems Inc. He is also an Adjunct Professor of Toxicology at the University of North Carolina at Chapel Hill and of Pharmacology and Toxicology at Duke University. He is Editor of Mutation Research - Reviews in Mutation Research. He has over 35 years of experience as a biochemist, genetic toxicologist, toxicogenomics and information scientist. Over the last 20 years Dr Waters has also worked extensively on database development; designing and conducting genotoxicity assays and chemoprevention studies in in vitro models; and studying genomics, clinical and toxicologic pathology.
Dr Russell S. Thomas is Director of the National Center for Computational Toxicology with the U.S. Environmental Protection Agency. Prior to this he was Director of the Institute for Chemical Safety Sciences at The Hamner Institutes for Health Sciences for over 10 years. Dr. Thomas also maintains an adjunct faculty appointment in the Division of Pharmacogenomics and Individualized Therapy at the University of North Carolina at Chapel Hill. Dr. Thomas has additionally been active in molecular biology and genomics research at the McArdle Cancer Research Laboratory at the University of Wisconsin.
Table of Contents
Section Title | Page | Action | Price |
---|---|---|---|
Cover | Cover | ||
Contents | ix | ||
Preface | vii | ||
Chapter 1 Introduction to Predictive Toxicogenomics for Carcinogenicity | 1 | ||
1.1 Background on -omics Technologies Applied in Toxicology | 1 | ||
1.1.1 Conventional Toxicity Testing | 5 | ||
1.1.2 Genomic and Postgenomic Technologies | 7 | ||
1.2 The Relative Roles of Toxicogenomics, Conventional Toxicity Testing, and High-throughput Screening | 12 | ||
1.3 Predictive Toxicology | 15 | ||
1.4 Systems Toxicology | 16 | ||
1.4.1 Dosimetry | 16 | ||
1.4.2 Adverse vs. Homeostatic Responses | 18 | ||
1.4.3 Phenotypic Anchoring | 18 | ||
1.4.4 Genetic Variation | 19 | ||
1.4.5 Validation | 19 | ||
1.4.6 Classes of Chemicals and Prototypic Compounds Studied to Date | 20 | ||
1.4.7 Target Organs Studied | 21 | ||
1.5 Predictive Carcinogenicity | 21 | ||
Acknowledgments | 26 | ||
References | 26 | ||
Chapter 2 Genomic Biomarkers in Cell-based Drug Screening | 39 | ||
2.1 Genotoxicity and the Traditional Testing Battery | 39 | ||
2.2 Mechanisms of Action for Genotoxicity and the Genotoxic Stress Responses | 40 | ||
2.2.1 Categorization by Mechanisms of Action | 40 | ||
2.2.2 Signaling and Transcriptional Responses upon Genotoxic Stress | 42 | ||
2.3 Expression Profiling and Toxicogenomics | 43 | ||
2.3.1 Genotoxicity Assays Based on Transcriptional Responses | 43 | ||
2.3.2 Toxicogenomics and Genomic Biomarkers | 45 | ||
2.3.3 Considerations in Biomarker Identification | 47 | ||
2.4 The Genotoxicity Biomarker TGx-28.65: Identification and Application | 48 | ||
2.4.1 Choice of the Cell Line and Toxicants | 48 | ||
2.4.2 Dose and Treatment Time Parameters | 49 | ||
2.4.3 Global Transcriptional Analysis | 51 | ||
2.4.4 Delineation of Gene Subclusters Using a Biclustering Approach | 53 | ||
2.4.5 Development of a Genomic Biomarker for Genotoxicity | 56 | ||
2.4.6 Validation and a Case Study on Utility of the TGx-28.65 Biomarker in Human Health Risk Assessment | 57 | ||
2.5 Summary and Perspectives | 58 | ||
References | 67 | ||
Chapter 3 Toxicogenomics In vitro: Gene Expression Signatures for Differentiating Genotoxic Mechanisms | 76 | ||
3.1 Introduction | 76 | ||
3.2 Predictive Toxicogenomics | 78 | ||
3.2.1 Overview of Predictive Toxicogenomics | 78 | ||
3.2.2 Rationale for the Need for Toxicogenomic Predictors of Genotoxicity | 79 | ||
3.3 Toxicogenomic Predictors of Genotoxicity | 80 | ||
3.3.1 Development and Validation of In vitro Toxicogenomic Predictors of Genotoxic MoAs | 80 | ||
3.3.2 Integration of Metabolic Activation | 93 | ||
3.3.3 Additional Considerations for Experimental Design of Predictive Toxicogenomics Studies | 95 | ||
3.4 Summary and Conclusions | 97 | ||
Acknowledgments | 99 | ||
References | 99 | ||
Chapter 4 In vivo Signatures of Genotoxic and Non-genotoxic Chemicals | 113 | ||
4.1 Introduction | 113 | ||
4.2 General Signature of Genotoxicity | 116 | ||
4.3 Liver | 129 | ||
4.3.1 Rat | 130 | ||
4.3.2 Mouse | 133 | ||
4.3.3 Human | 135 | ||
4.4 Kidney | 135 | ||
4.4.1 Rat | 135 | ||
4.4.2 Mouse | 137 | ||
4.4.3 Human | 137 | ||
4.5 Heart | 137 | ||
4.5.1 Rat | 138 | ||
4.5.2 Mouse | 138 | ||
4.5.3 Human | 139 | ||
4.6 Skeletal Muscle | 140 | ||
4.6.1 Rat | 140 | ||
4.6.2 Mouse | 140 | ||
4.6.3 Human | 140 | ||
4.7 Bone Marrow and Blood | 140 | ||
4.7.1 Rat | 141 | ||
4.7.2 Mouse | 141 | ||
4.7.3 Human | 141 | ||
4.8 Spleen | 143 | ||
4.8.1 Rat | 143 | ||
4.8.2 Mouse | 143 | ||
4.8.3 Human | 143 | ||
4.9 Other Tissues | 144 | ||
4.9.1 Rat | 144 | ||
4.9.2 Mouse | 144 | ||
4.9.3 Human | 144 | ||
4.10 Study Design | 145 | ||
4.10.1 Dose | 145 | ||
4.10.2 Duration | 146 | ||
4.10.3 Tissue Selection | 146 | ||
4.11 Conclusion | 147 | ||
Acknowledgments | 147 | ||
References | 147 | ||
Chapter 5 Transcriptomic Dose-Response Analysis for Mode of Action and Risk Assessment | 154 | ||
5.1 Introduction | 154 | ||
5.2 Traditional Statistical Methods for Analyzing Transcriptomic Dose-Response Data | 156 | ||
5.3 A Benchmark Dose Method for Analyzing Transcriptomic Data | 156 | ||
5.4 Application of Transcriptomic Dose-Response Analysis to MoA Assessment | 159 | ||
5.4.1 Case Study: Formaldehyde Exposure in Rat Nasal Epithelium | 160 | ||
5.4.2 Case Study: β-chloroprene in Mouse and Rat Lung\r | 162 | ||
5.4.3 Case Study: Naphthalene in the Rat Nasal and Olfactory Epithelium | 163 | ||
5.4.4 Case Study: Fenofibrate and Methapyrilene for Receptor-mediated Nongenotoxic Carcinogenesis | 165 | ||
5.5 Applications of Transcriptomic Dose-Response Analysis to Assess Cross-species Extrapolation | 166 | ||
5.6 On the use of Toxicity Pathways in Toxicity Testing, Transcriptional BMD Analysis, and Risk Assessment | 168 | ||
5.7 Comparison of Traditional Risk Assessment Approaches with Those Applying Transcriptomics | 173 | ||
5.8 Significance of Integrating Transcriptomic Data into Risk Assessment | 175 | ||
References | 176 | ||
Chapter 6 Using Transcriptomics to Evaluate Thresholds in Genotoxicity Dose-Response | 185 | ||
6.1 Introduction | 185 | ||
6.1.1 Challenges in Assessing the Safety of Potential Genotoxicants | 185 | ||
6.1.2 Understanding the Nature of the Threshold-shaped Dose-Response Curve | 187 | ||
6.2 Methods for Evaluating Low-dose Genotoxicity | 189 | ||
6.3 Micronucleus Dose Response | 190 | ||
6.4 Comparing Gene Signatures for Chemicals Causing Different Types of DNA Damage | 191 | ||
6.5 Integrating Dose-Response Trends for Transcriptomic and Micronucleus Endpoints | 196 | ||
6.6 Homeostasis, Transcriptional Regulation, and Post-translational Activity | 199 | ||
6.7 Suitability of Gene Expression Changes for Genotoxic Adversity | 201 | ||
References | 203 | ||
Chapter 7 Dissecting Modes of Action of Non-genotoxic Carcinogens | 209 | ||
7.1 Introduction | 209 | ||
7.1.1 Current Regulatory Requirements and Difficulties with Respect to Non-genotoxic Carcinogens | 209 | ||
7.1.2 What are Non-genotoxic Carcinogens? | 210 | ||
7.1.3 Possible Methods for the Identification of Non-genotoxic Carcinogens | 215 | ||
7.1.4 Comparison Approach | 215 | ||
7.2 Improving the Comparison Approach: A Case Study | 217 | ||
7.2.1 Cyclosporin A and Tacrolimus as Model Compounds | 217 | ||
7.2.2 Concentration Selection | 218 | ||
7.2.3 Microarray Analyses | 218 | ||
7.2.4 Comparison Approach on a Concentration Range | 219 | ||
7.2.5 Biological Response at the Pathway Level | 220 | ||
7.2.6 Biological Relevance of Comparison Approach | 225 | ||
7.3 Discussion and Future Prospects | 228 | ||
References | 230 | ||
Chapter 8 Human Embryonic Stem Cells as Biological Models to Examine the Impact of Xenobiotics on the Genome and Epigenome | 236 | ||
8.1 Introduction | 236 | ||
8.2 hES Cells as Biological Models in Toxicology | 238 | ||
8.3 Developing hES Cells into a Toxicology Testing Platform | 239 | ||
8.4 Stem Cells as a Biological Platform to Examine the Impact of Xenobiotics on the Genome and Epigenome | 239 | ||
8.5 The miRNA and lncRNA Epigenome | 241 | ||
8.6 Epigenomic Biomarkers and Toxicity Testing in the 21st Century | 243 | ||
Acknowledgments | 243 | ||
References | 244 | ||
Chapter 9 Novel Data Streams in the Assessment of Mutagenicity and Carcinogenicity: Implications for Cancer Hazard Assessment | 247 | ||
9.1 Integrating Across Multiple Data Streams to Reach Hazard Conclusions: Mechanistic Data can be Critical When Human Evidence is Less than Sufficient | 247 | ||
9.1.1 Introduction to the IARC Monographs Hazard Classification Process | 248 | ||
9.1.2 Recent Examples | 250 | ||
9.2 Predicting Chemical Carcinogenicity Using Mechanistic Data | 257 | ||
9.2.1 Toxicogenomics Studies to Predict Carcinogenicity | 258 | ||
9.2.2 In vitro Testing for Carcinogenicity-Moving Beyond Batteries | 258 | ||
9.2.3 Using Biomarkers of Cancer Risk in Molecular Epidemiology | 259 | ||
9.2.4 Filling Data Gaps: Predicting Chemical Carcinogenicity Using Modeling can Aid in Prioritization Exercises | 260 | ||
9.2.5 Addressing Human Variability | 261 | ||
9.3 Future Directions in Mechanistic Data Evaluations to Support IARC Monographs | 264 | ||
9.3.1 Systematic Approaches to Identifying and Presenting Mechanistic Evidence in Human Health Assessments | 264 | ||
9.3.2 High-throughput Data Analysis | 265 | ||
9.4 Conclusions and Future Directions | 267 | ||
Appendix 9.1: Classification Categories for the Overall Evaluation for the IARC Monographs (IARC 2006) | 268 | ||
References | 269 | ||
Chapter 10 Conazoles and Cancer: A Review | 280 | ||
10.1 Introduction and Background | 280 | ||
10.2 Inhibition of CYP51 by Conazoles | 281 | ||
10.2.1 Introduction to CYP51 | 281 | ||
10.2.2 Mechanism of the Inhibitory Action of CYP51 | 284 | ||
10.2.3 Inhibition of Fungal CYP51 | 285 | ||
10.2.4 Comparison of the Inhibition of Fungal and Human CYP51 | 287 | ||
10.2.5 Inhibition of Other Mammalian CYPs | 288 | ||
10.3 Induction of CYPs by Conazoles | 293 | ||
10.3.1 Introduction to Nuclear Receptors | 293 | ||
10.3.2 Induction of CYPs | 294 | ||
10.4 Hepatic Effects Induced by Conazoles | 294 | ||
10.4.1 Increased Liver Weight and Hypertrophy | 294 | ||
10.4.2 Cell Proliferation | 298 | ||
10.5 Effects on Serum Cholesterol and Triglyceride Levels | 300 | ||
10.6 Genotoxicity | 302 | ||
10.7 Tumorigenic Effects of Conazoles | 302 | ||
10.8 Toxicogenomic Studies in Mice | 306 | ||
10.8.1 Genomic Studies Using Liver Samples from Mice Treated with Conazoles | 306 | ||
10.8.2 Proteomic Studies Using Liver Samples from Mice Treated with Conazoles | 309 | ||
10.8.3 Metabolomic Studies Using Liver Samples from Mice Treated with Conazoles | 310 | ||
10.9 Toxicogenomic Studies in Rats | 312 | ||
10.9.1 Toxicological Studies in Rats | 312 | ||
10.9.2 Genomic Studies Using Liver Tissues from Rats Treated with Conazoles | 313 | ||
10.9.3 Genomic Studies Using Thyroid Tissues from Rats Treated with Conazoles | 313 | ||
10.10 Mode of Action of Propiconazole: Introduction | 314 | ||
10.10.1 Mode of Carcinogenic Action of Propiconazole, a Series of Key Events Leading to Cancer | 314 | ||
10.10.2 Key Event: Activate Nuclear Receptors | 316 | ||
10.10.3 Key Event: CYP Induction | 316 | ||
10.10.4 Key Event: Induction of ROS and Oxidative Stress | 317 | ||
10.10.5 Key Event: Increase in Endogenous DNA Adduct Levels and Mutations | 319 | ||
10.10.6 Key Event: Increased atRA Metabolism | 320 | ||
10.10.7 Key Event: Decreased Hepatic Levels of atRA | 320 | ||
10.10.8 Key Event: Inhibit CYP51 Activity | 320 | ||
10.10.9 Key Event: Dysregulation of Cholesterol Biosynthesis and Metabolism | 321 | ||
10.10.10 Key Event: Increase in Cell Proliferation by Decreased Levels of atRA and by a Mevalonic Acid/Cholesterol Biosynthesis Feedback Mechanism | 321 | ||
10.10.11 Key Event: Hepatocellular Tumors | 324 | ||
10.11 Mode of Carcinogenic Action of Propiconazole: Discussion | 325 | ||
10.12 Mode of Carcinogenic Action: Human Relevance | 327 | ||
10.12.1 Species Comparison Across Key Events | 327 | ||
10.12.2 Comparison of Toxicologic and Genomic Studies Comparing Mouse Liver Responses to Conazoles and Phenobarbital | 328 | ||
10.13 Conclusions | 334 | ||
Acknowledgments | 335 | ||
References | 335 | ||
Chapter 11 Application of Transcriptomics in Exposed Human Populations: Benzene as an Example | 352 | ||
11.1 Application of Toxicogenomics in Occupational Benzene Exposure | 352 | ||
11.1.1 Mechanisms and Biomarkers of Benzene Toxicity | 354 | ||
11.1.2 Discerning Low-dose Effects is a Challenge in Risk Assessment | 354 | ||
11.1.3 Toxicogenomic Studies | 355 | ||
11.2 Transcriptomic Studies of Occupational Benzene Exposure | 356 | ||
11.2.1 The Complex Human Transcriptome and Its Analysis | 356 | ||
11.2.2 Microarrays | 357 | ||
11.2.3 RNA-Seq | 361 | ||
11.2.4 NanoString | 365 | ||
11.2.5 L1000 and S1500 Platforms | 365 | ||
11.2.6 Transcriptomic Platform Choice and Study Design Considerations | 368 | ||
11.3 Future Directions and Translation | 368 | ||
11.4 Conclusion | 369 | ||
Acknowledgments | 370 | ||
References | 370 | ||
Chapter 12 Toxicogenomics Case Study: Furan | 390 | ||
12.1 Introduction | 390 | ||
12.1.1 Chemical Testing and Toxicogenomics | 391 | ||
12.1.2 Reducing Animal use in Toxicity Testing | 393 | ||
12.2 Toxicogenomics Case Study: Furan | 396 | ||
12.2.1 Liver Physiology and Hepatocarcinogenesis | 396 | ||
12.2.2 Test Article: Furan | 398 | ||
12.2.3 Quantitative Toxicogenomics | 402 | ||
12.2.4 Predictive Toxicogenomics | 403 | ||
12.2.5 Mechanistic Toxicogenomics | 405 | ||
12.2.6 FFPE Toxicogenomics | 408 | ||
12.3 Role for Toxicogenomics in Chemical Risk Assessment | 409 | ||
12.3.1 Guidelines for Using Toxicogenomics Data in Formal Risk Assessment | 410 | ||
12.3.2 FFPE Genomics in Risk Assessment | 411 | ||
12.3.3 Adverse Outcome Pathways | 412 | ||
12.3.4 Toxicogenomics in Tiered Testing Strategies | 413 | ||
12.4 Concluding Remarks | 414 | ||
References | 415 | ||
Chapter 13 The Parallelogram Approach to Assess Human Relevance of Toxicogenomics-derived Toxicity Pathways in Human Health Risk Assessment | 423 | ||
13.1 Human Health Risk Assessment | 423 | ||
13.2 Toxicogenomics in Risk Assessment | 425 | ||
13.2.1 Toxicogenomics in Hazard Identification | 426 | ||
13.2.2 Toxicogenomics in Dose-Response Modeling | 426 | ||
13.3 Assessing Biological Significance and Human Relevance of Toxicogenomics Data | 427 | ||
13.3.1 The Parallelogram Approach | 427 | ||
13.3.2 The Concordance Model | 431 | ||
13.4 Discussion | 435 | ||
13.4.1 Pathway Analysis | 436 | ||
13.4.2 Toxicity Pathway-derived BMDs | 436 | ||
13.4.3 Apical Endpoints | 437 | ||
13.4.4 Adversity | 437 | ||
13.5 Conclusion | 438 | ||
References | 438 | ||
Chapter 14 Bioinformatics of Genomics in the Assessment of Cancer | 442 | ||
14.1 Introduction | 442 | ||
14.1.1 Classification and Prediction | 445 | ||
14.1.2 Over-represented Pathways, Enriched Gene Sets and Gene Regulatory Networks | 453 | ||
14.1.3 Bioinformatics and Computational Biology for Integrative Genomics | 468 | ||
14.2 The Future can be Now | 473 | ||
Acknowledgments | 475 | ||
References | 475 | ||
Subject Index | 484 |