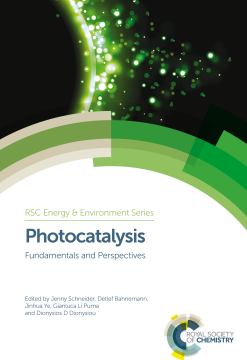
BOOK
Photocatalysis
Jenny Schneider | Detlef Bahnemann | Jinhua Ye | Gianluca Li Puma | Dionysios D Dionysiou
(2016)
Additional Information
Book Details
Abstract
Combining the basic concepts of photocatalysis with the synthesis of new catalysts, reactor and reaction engineering, this book provides a comprehensive resource on the topic. The book introduces the fundamental aspects of photocatalysis including the role of surface chemistry and understanding the chemistry of photocatalytic processes before exploring the theory and experimental studies of charge carrier dynamics. Specific chapters then cover new materials for the degradation of organics; water splitting and CO2 reduction; as well as reactor and reaction engineering. Researchers new to this discipline can learn the first principles, whilst experienced researchers can gain further information about aspects in photocatalysis beyond their area of expertise. Together with Photocatalysis: Applications, these volumes provide a complete overview to photocatalysis.
Detlef Bahnemann is a Professor at the Institute for Technical Chemistry, Gottfried Wilhelm Leibniz University of Hannover, Germany, and Director of the Laboratory for Photoactive Nanocomposite Materials at Saint-Petersburg State University, Russia. He has worked in the field of photocatalysis for over 30 years.
Jenny Schneider is a Researcher at the Institute for Technical Chemistry, Gottfried Wilhelm Leibniz University of Hannover, Germany. She is a specialist in time-resolved investigations of photocatalytic processes.
Jinhua Ye is Managing Director for the Photo-Catalytic Materials Center (PCMC) at the National Institute for Materials Science, Japan. Her research is dedicated to developing new photocatalytic materials for environment preservation.
Gianluca Li Puma is Professor of Chemical and Environmental Engineering at Loughborough University, UK. He is an expert in reaction and reactor engineering, including photochemical and photocatalytic systems.
Dionysios D. Dionysiou is a Professor in the Environmental Engineering and Science Program, Department of Biomedical, Chemical and Environmental Engineering (DBCEE), University of Cincinnati, USA. He has over 20 years of experience in the field of photocatalysis.
Table of Contents
Section Title | Page | Action | Price |
---|---|---|---|
Cover | Cover | ||
Photocatalysis Fundamentals and Perspectives | i | ||
Preface | v | ||
Contents | ix | ||
Part 1 - Fundamental Aspects of Photocatalysis | 1 | ||
Chapter 1 - Photoelectrochemistry: From Basic Principles to Photocatalysis | 3 | ||
1.1 Introduction | 3 | ||
1.2 A Brief Summary of Semiconductor Physics | 4 | ||
1.3 Conventional Semiconductor Photoelectrodes | 6 | ||
1.3.1 Potential and Charge Distribution Across the Semiconductor–Inert Electrolyte Junction | 6 | ||
1.3.2 The Semiconductor–Redox Electrolyte Junction | 9 | ||
1.3.3 The Semiconductor–Electrolyte Junction Under Illumination | 13 | ||
1.3.4 Quasi-Fermi Levels (QFLs) | 15 | ||
1.4 Nanostructured Semiconductor Electrodes and Colloidal Particles in the Dark | 17 | ||
1.4.1 Band Bending in Nanostructures | 17 | ||
1.4.2 Determination of Quasi-Fermi Level Positions in Nanoparticle Suspensions | 19 | ||
1.5 Surface States and Fermi Level Pinning | 20 | ||
1.6 Surface Recombination | 22 | ||
1.7 Charge Compensation and Charge Trapping in Mesoporous Electrodes | 25 | ||
1.8 Conclusions | 25 | ||
References | 26 | ||
Chapter 2 - Understanding the Chemistry of Photocatalytic Processes | 29 | ||
2.1 Thermodynamic Constraints for Photocatalytic Processes | 29 | ||
2.2 Single and Multiple Electron Transfer Reactions | 35 | ||
2.3 Role of the Substrate Structure in the Photocatalytic Process | 37 | ||
2.4 Importance of the Reduction Pathway in Photocatalytic Oxidation Reactions | 40 | ||
2.5 Importance of the Oxidation Pathway in Photocatalytic Reduction Reactions | 45 | ||
2.6 Conclusions | 48 | ||
References | 48 | ||
Chapter 3 - Current Issues Concerning the Mechanism of Pristine TiO2 Photocatalysis and the Effects on Photonic Crystal Nanostructures | 51 | ||
3.1 Photocatalysis and Sustainability | 51 | ||
3.2 The Basic Principle of TiO2 Photocatalysis | 53 | ||
3.3 Current Mechanisms | 57 | ||
3.3.1 Antenna Mechanism | 57 | ||
3.3.2 Deaggregation of Particle Agglomerates | 59 | ||
3.3.3 Band-Gap Coupling: Z-Scheme and Heterojunctions | 61 | ||
3.3.4 Wettability | 64 | ||
3.3.4.1 Creation of OH Surface Groups | 64 | ||
3.3.4.2 Impurities Removal | 66 | ||
3.3.4.3 Adsorbed and Desorbed Water | 68 | ||
3.3.4.4 Some Remarks About Wettability | 69 | ||
3.3.5 Photo-Thermal Desorption of Water | 69 | ||
3.4 TiO2 Photonic Crystal Nanostructures | 70 | ||
3.5 Concluding Remarks | 74 | ||
References | 76 | ||
Chapter 4 - Specificity in Photocatalysis | 80 | ||
4.1 Introduction | 80 | ||
4.2 Mass Transport to the Photocatalyst and Adsorption | 82 | ||
4.2.1 Complexation in the Fluid Phase | 83 | ||
4.2.2 Surface Charge Effects | 84 | ||
4.2.3 Overcoating the Photocatalyst | 85 | ||
4.2.4 Adsorb & Shuttle | 86 | ||
4.2.5 Doping | 93 | ||
4.2.6 Selection by Size | 94 | ||
4.2.7 Molecular Imprinting | 95 | ||
4.3 The Redox Reaction | 99 | ||
4.3.1 Recombination Versus Interfacial Electron Transfer | 99 | ||
4.3.2 Doping as a Means to Control Oxidation Versus Reduction | 99 | ||
4.3.3 Shifting the Location of Energy Bands | 100 | ||
4.3.4 Co-Existing Compounds as a Means to Alter Specificity | 100 | ||
4.3.5 Utilizing Specific Adsorbate–Adsorbent Interactions | 101 | ||
4.3.6 Surface Derivatization | 101 | ||
4.3.7 Sensitization as a Means to Induce Specificity | 102 | ||
4.4 Desorption of Products | 103 | ||
4.4.1 Preferential Desorption from Imprinted Photocatalysts | 103 | ||
4.4.2 Effect of Solvents on the Desorption of Intermediate Products | 104 | ||
4.4.3 Surface Derivatization for Controlling the Distribution of Products | 105 | ||
4.5 Summary and Perspectives | 106 | ||
References | 106 | ||
Chapter 5 - Photoexcitation in Pure and Modified Semiconductor Photocatalysts | 110 | ||
5.1 Band-Gap Excitation of Semiconductor Photocatalysts | 110 | ||
5.2 Photoexcitation of Impurity-Doped Semiconductors | 112 | ||
5.3 Photoexcitation of Coupled Semiconductors | 115 | ||
5.4 Dye-Sensitized Semiconductors and Dye Discoloration | 117 | ||
5.5 LMCT-Sensitized Semiconductors | 119 | ||
5.6 Photoexcitation at Metal/Semiconductor Interfaces | 121 | ||
5.7 Conclusions | 124 | ||
Acknowledgements | 124 | ||
References | 124 | ||
Chapter 6 - New Concepts in Photocatalysis | 129 | ||
6.1 Introduction | 129 | ||
6.2 Graphene | 130 | ||
6.3 Carbon Nitride | 134 | ||
6.4 Z-Scheme Photocatalytic Systems | 139 | ||
6.4.1 Z-Scheme Systems with Redox Mediator | 140 | ||
6.4.2 Z-Scheme Systems Without Redox Mediator | 142 | ||
6.5 Plasmonic Photocatalysis | 147 | ||
6.6 New Applications of Photocatalysis | 153 | ||
6.7 Perspectives | 156 | ||
References | 157 | ||
Part 2 - Primary Processes in Photocatalysis | 163 | ||
Chapter 7 - Kinetic Processes in the Presence of Photogenerated Charge Carriers | 165 | ||
7.1 Outline of the Processes in Photocatalysis | 165 | ||
7.1.1 Environmental Clean-up or Solar Hydrogen Production | 165 | ||
7.1.2 Energy Levels of TiO2 and Water | 166 | ||
7.1.3 Adsorption of Water Molecules | 167 | ||
7.2 Primary Processes of Photogenerated Charge Carriers | 169 | ||
7.2.1 Trapping of Free Charge Carriers | 169 | ||
7.2.2 Trapped Electrons and Reduction of O2 | 171 | ||
7.2.3 Trapped Holes and Oxidation of Alcohols | 172 | ||
7.3 Kinetic Processes at Pure TiO2 Photocatalysts | 172 | ||
7.3.1 O2 Production at Rutile Surfaces | 172 | ||
7.3.2 •OH Radical Formation over Rutile and Anatase Photocatalysts | 173 | ||
7.3.3 Kinetics of Methanol Oxidation | 176 | ||
7.4 Modified TiO2 Photocatalysts for Visible Light Response | 178 | ||
7.4.1 Copper(ii) deposited TiO2 and WO3 | 178 | ||
7.4.2 Iron(iii)-deposited Ru-doped TiO2 | 179 | ||
7.4.3 Platinum Complex Sensitized TiO2 | 180 | ||
7.4.4 Gold-Nanoparticle Deposited TiO2 | 181 | ||
References | 182 | ||
Chapter 8 - Traps and Interfaces in Photocatalysis: Model Studies on TiO2 Particle Systems | 185 | ||
8.1 Introduction | 185 | ||
8.2 The Solid–Gas Interface: Trapping Sites and Spectroscopic Manifestations | 188 | ||
8.2.1 Trapped Electrons | 188 | ||
8.2.2 Trapped Holes | 192 | ||
8.2.3 Trapped Hydrogen | 193 | ||
8.2.4 Trapped Charges and Optical Fingerprints | 194 | ||
8.3 Slow Charge Trapping and Charge Carrier Quantification at the Solid–Gas Interface | 195 | ||
8.4 From Solid (Particle)–Gas to Solid (Particle)–Liquid Interfaces: Changes on Different Size Scales | 199 | ||
8.5 Microstructural Changes of Particle Ensembles and Solid–Solid Interface Formation | 201 | ||
8.6 Charge Separation and Trapping at the Solid–Liquid Interface – Slow Processes | 204 | ||
8.7 Summary and Outlook | 209 | ||
Acknowledgements | 211 | ||
References | 211 | ||
Chapter 9 - Interplay Between Physical and Chemical Events in Photoprocesses in Heterogeneous Systems | 218 | ||
9.1 Introduction | 218 | ||
9.2 Physical and Chemical Relaxation through Surface-Active Centers | 223 | ||
9.3 Photoinduced Defect Formation | 226 | ||
9.4 Interconnection Between the Activity and Selectivity of Photocatalysts | 230 | ||
9.4.1 Activity of Photocatalysts | 230 | ||
9.4.2 Selectivity of Photocatalysts | 235 | ||
9.5 Concluding Remarks | 243 | ||
Acknowledgements | 243 | ||
References | 243 | ||
Part 3 - New Materials | 245 | ||
Chapter 10 - New Materials: Outline | 247 | ||
References | 249 | ||
Chapter 11 - New Materials for Degradation of Organics | 252 | ||
11.1 Basic Characterizations by which to Judge a New Material as Photocatalyst | 253 | ||
11.1.1 Dark and Light Experiments | 253 | ||
11.1.2 Wavelength-Dependence Test | 254 | ||
11.1.3 Evidence for Catalytic Process | 255 | ||
11.2 Typical New Materials for Photodegradation of Organics | 256 | ||
11.2.1 New-Generation TiO2-Based Materials | 256 | ||
11.2.2 Photocatalysts Comprising d-Block Elements | 261 | ||
11.2.2.1 Photocatalysts with d0-Block Elements | 261 | ||
11.2.2.1.1 Ti-Based Materials. | 261 | ||
11.2.2.1.2 V, Nb, Ta-Containing Materials. | 261 | ||
11.2.2.1.3 Mo, W-Containing Materials. | 263 | ||
11.2.2.2 Photocatalysts with d10-Block Elements | 263 | ||
11.2.2.2.1 Cu-Containing Materials. | 263 | ||
11.2.2.2.2 Ag-Based Materials. | 263 | ||
11.2.2.2.3 Zn, Cd-Containing Materials. | 264 | ||
11.2.3 Photocatalysts Containing p-Block Elements | 265 | ||
11.2.3.1 Sn, Pb-Included Materials | 265 | ||
11.2.3.2 Bi-Based Materials | 273 | ||
11.2.4 Organic Photocatalysts | 273 | ||
11.2.4.1 C3N4-Based Materials | 274 | ||
11.2.4.2 Metal–Organic Framework (MOF) Materials | 275 | ||
11.2.4.3 Other Materials | 275 | ||
11.2.5 Composite and Heterojunction Photocatalysts | 275 | ||
11.2.5.1 Photosensitizer@Active Material Composites or Heterojunctions | 275 | ||
11.2.5.2 Band-Structure Matched p–n or n–n Heterojunctions | 281 | ||
11.2.5.3 Conductive Material@Semiconductor Composites or Heterojunctions | 281 | ||
11.3 Photodegradation Mechanism | 281 | ||
11.3.1 Intrinsic Semiconductor-Based Photocatalysis or Dye-Sensitized Photocatalysis | 286 | ||
11.3.2 Reactive Species Analysis | 287 | ||
11.4 Summary and Prospects | 289 | ||
References | 289 | ||
Chapter 12 - New Materials for Water Splitting | 295 | ||
12.1 Introduction | 295 | ||
12.1.1 Research Background | 295 | ||
12.1.2 Basic Principles of Water Splitting on a Semiconductor Particle | 296 | ||
12.1.3 Development of Visible-Light-Responsive Photocatalysts for Overall Water Splitting | 299 | ||
12.1.4 Scope of This Chapter | 301 | ||
12.2 Modified Oxynitrides for Efficient Water Splitting | 301 | ||
12.2.1 Surface Modified Tantalum Oxynitrides with Zr(iv) Species for Enhanced Hydrogen Evolution | 302 | ||
12.2.2 Oxynitrides Modified with Cobalt Oxide for Highly Efficient Water Oxidation | 306 | ||
12.3 Metal Oxide Based Photocatalysts for Overall Water Splitting | 307 | ||
12.3.1 Doped SrTiO3 | 308 | ||
12.3.2 Dye-Sensitized Lamellar Niobate for Z-Scheme Water Splitting | 312 | ||
12.4 Summary and Outlook | 314 | ||
References | 315 | ||
Chapter 13 - New Materials for CO2 Photoreduction | 318 | ||
13.1 Introduction | 318 | ||
13.2 Basic Principles of Photocatalytic Reduction of CO2 | 319 | ||
13.3 Materials for CO2 Photoreduction | 322 | ||
13.3.1 Metal Oxides with d0 and d10 Electronic Configurations | 322 | ||
13.3.2 Metal Sulfides and Phosphides | 324 | ||
13.3.3 Other Materials | 325 | ||
13.4 Strategies for Designing Effective Photocatalytic Materials | 325 | ||
13.4.1 Surface Sites for Reactant Adsorption and Chemical Reaction | 326 | ||
13.4.1.1 Porous Structure with Large Surface Area | 326 | ||
13.4.1.2 Optimized Surface Reactivity via Facet Engineering | 328 | ||
13.4.1.3 Surface Modification | 330 | ||
13.4.1.4 Surface Oxygen Vacancy | 331 | ||
13.4.2 Light Harvesting for Effectively Utilizing Solar Energy | 331 | ||
13.4.2.1 Ion Doping | 331 | ||
13.4.2.2 Solid Solutions | 332 | ||
13.4.2.3 Sensitization | 333 | ||
13.4.3 Charge Separation for Effectively Utilizing Solar Energy | 333 | ||
13.4.3.1 Loading Co-Catalysts | 333 | ||
13.4.3.2 One-Dimensional Nanostructures | 335 | ||
13.4.3.3 Heterojunction Construction | 336 | ||
13.4.3.4 Z-Scheme CO2 Reduction | 336 | ||
13.5 Conclusions and Perspectives | 337 | ||
References | 337 | ||
Part 4 - Reactor and Reaction Engineering | 349 | ||
Chapter 14 - Fundamentals of Radiation Transport in Absorbing Scattering Media | 351 | ||
14.1 Introduction | 351 | ||
14.2 Definitions | 352 | ||
14.2.1 Radiation Intensity | 353 | ||
14.2.2 Incident Radiation | 353 | ||
14.2.3 Local Volumetric Rate of Photon Absorption (LVRPA) | 354 | ||
14.2.4 Net Radiation Flux | 354 | ||
14.2.5 Local Surface Rate of Photon Absorption (LSRPA) | 354 | ||
14.3 The Radiative Transport Equation (RTE) | 355 | ||
14.4 Boundary Conditions for the RTE | 357 | ||
14.4.1 Extended Source with Superficial Emission (ESSE) | 358 | ||
14.4.2 Extended Source with Voluminal Emission (ESVE) | 358 | ||
14.4.3 Solar Radiation | 360 | ||
14.4.4 Chemical Actinometry | 361 | ||
14.5 Solution Methods of the RTE | 363 | ||
Acknowledgements | 364 | ||
References | 365 | ||
Chapter 15 - Photocatalytic Reactor Design | 367 | ||
15.1 Predictive Design of Photocatalytic Reactors | 367 | ||
15.2 Optical Properties of Photocatalytic Suspensions and Films | 369 | ||
15.3 Radiation Field Inside the Reactor | 373 | ||
15.4 Photocatalytic Efficiencies | 373 | ||
15.4.1 Quantum Yield and Quantum Efficiency | 373 | ||
15.4.2 Photonic Yield and Photonic Efficiency | 374 | ||
15.5 Kinetic Modeling | 375 | ||
15.6 Mass Balance Equations | 377 | ||
15.7 Case Study: Photocatalytic Oxidation of Cyanide with TiO2/SiO2 Materials | 378 | ||
15.7.1 Experimental Determination of the Optical Properties of the Materials | 378 | ||
15.7.2 Estimation of the Quantum Efficiency | 379 | ||
15.7.3 Development of a Suitable Intrinsic Kinetic Model | 382 | ||
15.7.4 Scaling-Up of the Process to a Larger Photocatalytic Reactor | 383 | ||
Acknowledgements | 386 | ||
References | 387 | ||
Chapter 16 - Photocatalytic Reactor Modeling | 388 | ||
16.1 Introduction | 388 | ||
16.2 Radiation Field Evaluation | 390 | ||
16.3 Emission Model (Lamps) | 390 | ||
16.4 Solar Emission Model | 391 | ||
16.5 Photon Absorption–Scattering Model: Evaluation of the Local Volumetric Rate of Photon Absorption, LVRPA | 393 | ||
16.5.1 Two-Flux and Six-Flux Absorption–Scattering Models | 394 | ||
16.6 Application of SFM in Flat-Plate Photoreactors | 397 | ||
16.7 Application of SFM in Solar CPC and FPR Reactors | 399 | ||
16.8 Photocatalytic Reaction Kinetics Model | 401 | ||
16.9 Generalized Model | 405 | ||
16.10 Thin-Film Slurry Photocatalytic Reactors | 408 | ||
16.11 Kinetic Parameters Optimization | 412 | ||
16.12 Application of Models to the Photocatalytic Degradation of Organic Compounds in Water | 414 | ||
16.12.1 DCA Solar Photodegradation | 415 | ||
16.12.2 Phenol and 4-Chlorophenol Solar Photodegradation | 416 | ||
16.13 Photodegradation of Organic Mixtures | 418 | ||
References | 420 | ||
Subject Index | 425 |