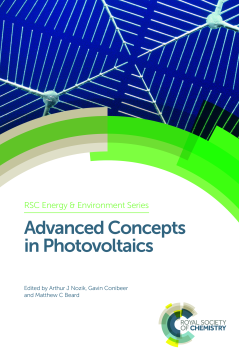
Additional Information
Book Details
Abstract
Photovoltaic systems enable the sun’s energy to be converted directly into electricity using semiconductor solar cells. The ultimate goal of photovoltaic research and development is to reduce the cost of solar power to reach or even become lower than the cost of electricity generated from fossil and nuclear fuels. The power conversion efficiency and the cost per unit area of the phototvoltaic system are critical factors that determine the cost of photovoltaic electricity. Until recently, the power conversion efficiency of single-junction photovoltaic cells has been limited to approximately 33% - the so-called Shockley-Queisser limit.
This book presents the latest developments in photovoltaics which seek to either reach or surpass the Shockley-Queisser limit, and to lower the cell cost per unit area. Progress toward this ultimate goal is presented for the three generations of photovoltaic cells: the 1st generation based on crystalline silicon semiconductors; the 2nd generation based on thin film silicon, compound semiconductors, amorphous silicon, and various mesoscopic structures; and the 3rd generation based on the unique properties of nanoscale materials, new inorganic and organic photoconversion materials, highly efficient multi-junction cells with low cost solar concentration, and novel photovoltaic processes.
The extent to which photovoltaic materials and processes can meet the expectations of efficient and cost effective solar energy conversion to electricity is discussed. Written by an international team of expert contributors, and with researchers in academia, national research laboratories, and industry in mind, this book is a comprehensive guide to recent progress in photovoltaics and essential for any library or laboratory in the field.
Professor Nozik is a Professor Adjoint in the Department of Chemistry and Biochemistry and a Senior Research Fellow Emeritus at the National Renewable Energy Laboratory (NREL) in Golden, Colorado; NREL is one of the National Laboratories of the U.S. Department of Energy. He maintains an active interaction and collaboration with his colleagues at NREL.
Professor Conibeer is deputy director of the School of Photovoltaics and Renewable Energy Engineering at the University of New South Wales, Australia. His research interests include third generation photovoltaics; hot carrier cooling in semiconductors and phonon dispersion modulation in nanostructures.
Table of Contents
Section Title | Page | Action | Price |
---|---|---|---|
Advanced Concepts in Photovoltaics | i | ||
Contents | xii | ||
Chapter 1 Crystalline Silicon Solar Cells with High Efficiency | 1 | ||
1.1 Introduction | 1 | ||
1.2 Efficiency Limitations | 2 | ||
1.2.1 Theoretical Limitations: The Auger Limit | 2 | ||
1.2.2 Practical Limitations | 5 | ||
1.3 Screen-printed Al-BSF Solar Cells on p-type Silicon | 7 | ||
1.3.1 Standard Al-BSF Cell | 9 | ||
1.3.2 Improved Al-BSF Formation by Boron Co-doping | 10 | ||
1.3.3 Improved Emitter | 10 | ||
1.4 Solar Cells with Dielectric Rear Passivation on p-type Silicon | 11 | ||
1.4.1 Rear Passivation Layers | 12 | ||
1.4.2 Contacting Schemes | 13 | ||
1.4.3 Lifetime Limitations in Boron-doped p-type Silicon | 13 | ||
1.5 Solar Cells on n-type Silicon | 14 | ||
1.5.1 n-type BSF Cell Structures | 15 | ||
1.5.2 n-type Cell Structures with Dielectric Rear Passivation | 17 | ||
1.5.3 Heterojunction Solar Cells | 17 | ||
1.5.4 Back-contacted Back-junction Solar Cells | 19 | ||
1.5.5 Back-contacted Back-junction Solar Cells with Passivated Contacts | 20 | ||
1.6 Conclusion | 20 | ||
Acknowledgements | 21 | ||
References | 21 | ||
Chapter 2 Tandem and Multiple-junction Devices Based on Thin-film Silicon Technology | 30 | ||
2.1 Introduction | 30 | ||
2.2 Material Properties | 32 | ||
2.2.1 Hydrogenated Amorphous Silicon (a-Si:H) and its Alloys | 32 | ||
2.2.2 Hydrogenated Microcrystalline Silicon (μc-Si:H) and its Alloys | 34 | ||
2.3 Basis of Thin-film Silicon-based Multiple- junction Devices | 38 | ||
2.3.1 Solar Cells Based on Thin Films of Silicon | 38 | ||
2.3.2 Possible Multiple-junction Devices Based on Thin Films of Silicon | 38 | ||
2.3.3 Matching Considerations | 40 | ||
2.3.4 Combining Light Management and High-quality Absorber Layers | 40 | ||
2.4 State of the Art | 41 | ||
2.5 Current Limitations and Prospective Concepts | 42 | ||
2.5.1 Increasing Light Absorption in the Absorber | 43 | ||
2.5.2 Improvements in Silicon Materials | 48 | ||
2.6 Conclusions and Perspectives | 51 | ||
References | 51 | ||
Chapter 3 Thin-film CdTe Photovoltaic Solar Cell Devices | 61 | ||
3.1 Introduction | 61 | ||
3.1.1 History of CdTe Photovoltaic Devices | 62 | ||
3.1.2 Layer-specific Process Description for Superstrate CdTe Devices | 65 | ||
3.2 Important and Under-reported Processes | 70 | ||
3.2.1 Buffer Layers | 70 | ||
3.2.2 Incorporation of Cu | 72 | ||
3.2.3 Defects and Defect Modeling | 74 | ||
3.2.4 Junction Formation and Location | 79 | ||
3.3 Conclusions | 81 | ||
Acknowledgements | 81 | ||
References | 81 | ||
Chapter 4 III–V Multi-junction Solar Cells | 87 | ||
4.1 Introduction | 87 | ||
4.2 On the Efficiency of III–V Multi-junction Solar Cells | 91 | ||
4.2.1 Photovoltaic Cells and Monochromatic Light: A Perfect Match | 91 | ||
4.2.2 Towards a Match with the Solar Spectrum: Stacking Photovoltaic Cells | 93 | ||
4.3 The Technological Toolbox to Fabricate III–V Multi-junction Solar Cells | 95 | ||
4.3.1 Epitaxial Growth Methods | 96 | ||
4.3.2 Substrates | 98 | ||
4.3.3 Epitaxial Growth Concepts | 99 | ||
4.3.4 Materials | 101 | ||
4.3.5 Post-growth Technological Processing | 103 | ||
4.4 Some Members of the III–V Multi-junction Solar Cell Family | 104 | ||
4.4.1 Upright Metamorphic Devices on Ge Substrates | 104 | ||
4.4.2 Inverted Metamorphic Multi-junction Solar Cells | 105 | ||
4.4.3 III–V on Si | 106 | ||
4.4.4 Wafer-bonded Multi-junction Solar Cells | 108 | ||
4.4.5 Lattice-matched Growth of more than Three Junctions | 108 | ||
4.5 Conclusion | 108 | ||
Acknowledgements | 109 | ||
References | 109 | ||
Chapter 5 Thin-film Photovoltaics Based on Earth-abundant Materials | 118 | ||
5.1 Introduction | 118 | ||
5.1.1 Future Requirements for Photovoltaics: 2050 Scenarios | 118 | ||
5.1.2 Resource Implications for Thin-film Photovoltaics | 119 | ||
5.1.3 Earth-abundant Absorbers | 120 | ||
5.1.4 The Scope of the Chapter | 122 | ||
5.2 Kesterite: a Case Study | 122 | ||
5.2.1 Iso-electronic Substitution: An Introduction to Cu2ZnSnS(Se)4 | 122 | ||
5.2.2 A Comparison of Phase Equilibria in the Cu-In-Se and Cu-Zn-Sn-Se Systems | 128 | ||
5.2.3 Electronic Properties | 131 | ||
5.3 Preparative Routes to Earth-abundant Absorber Films | 136 | ||
5.3.1 Thermodynamic Considerations | 137 | ||
5.3.2 Kinetic Considerations | 142 | ||
5.3.3 Preparative Methods | 144 | ||
5.3.4 Summary | 149 | ||
5.4 Device Fabrication and Characterization | 150 | ||
5.5 Other Earth-abundant Materials | 151 | ||
5.5.1 Phase Equilibria Considerations | 152 | ||
5.5.2 Phase Stability Considerations | 153 | ||
5.5.3 Opto-electronic Considerations | 157 | ||
5.5.4 Application of Criteria of Earth Abundance, Thermodynamics, and Opto-electronic Properties to Other Potential Absorber Materials | 159 | ||
5.6 Summary and Outlook | 169 | ||
Acknowledgements | 169 | ||
References | 170 | ||
Chapter 6 Chemistry of Sensitizers for Dye-sensitized Solar Cells | 186 | ||
6.1 Introduction | 186 | ||
6.2 Ruthenium Sensitizers | 192 | ||
6.2.1 High Molar Extinction Coefficient Sensitizers | 195 | ||
6.2.2 Panchromatic Ruthenium Sensitizers | 195 | ||
6.2.3 Cyclometallated NCS-free Ruthenium Sensitizers | 200 | ||
6.2.4 Cyclometallated NCS-free Ruthenium Dyes with a Com/Con Redox Shuttle | 201 | ||
6.3 Metal-free Organic Sensitizers | 204 | ||
6.3.1 Organic Sensitizers and their Cobalt Electrolyte Compatibility | 206 | ||
6.3.2 Size Effect of the Donor Groups in the Cobalt Electrolyte Compatibility of Dyes | 208 | ||
6.3.3 Towards Cobalt Electrolyte Compatible Panchromatic Organic Dyes | 209 | ||
6.3.4 Donor–Chromophore–Acceptor-based Asymmetric Diketopyrrolopyrrole Sensitizers | 213 | ||
6.3.5 Ullazine-based Sensitizers | 216 | ||
6.4 Porphyrin Sensitizers | 217 | ||
6.4.1 Towards High Effciency and Cobalt Compatible meso-Porphyrin Sensitizers | 219 | ||
6.4.2 Towards Panchromatic, High Effciency and Cobalt Compatible meso-Porphyrin Sensitizers | 221 | ||
6.5 Perovskite Sensitizers for Solid-state Solar Cells | 223 | ||
6.5.1 One-step Precursor Solution Deposition | 225 | ||
6.5.2 Two-step Sequential Deposition Method | 225 | ||
6.5.3 Dual-source Vapour Deposition | 227 | ||
6.6 Conclusion | 229 | ||
Acknowledgements | 230 | ||
References | 231 | ||
Chapter 7 Perovskite Solar Cells | 242 | ||
7.1 Introduction | 242 | ||
7.2 Synthesis of Organolead Halide Perovskite | 244 | ||
7.3 Crystal Structure and Related Properties | 244 | ||
7.4 Opto-electronic Properties | 246 | ||
7.5 Perovskite Solar Cell Fabrication | 249 | ||
7.6 Device Structures and Performances | 250 | ||
7.6.1 CH3NH3PbI3-based Perovskite Solar Cells | 250 | ||
7.6.2 Mixed Halide and Non-iodide Perovskite Solar Cells | 252 | ||
7.6.3 Planar Heterojunction Without Mesoporous Oxide Layers | 254 | ||
7.7 Summary | 255 | ||
Acknowledgements | 255 | ||
References | 255 | ||
Chapter 8 All-oxide Photovoltaics | 258 | ||
8.1 Introduction to All-oxide Photovoltaics | 258 | ||
8.2 Solar Cell Design Rules | 259 | ||
8.2.1 Light Absorption | 259 | ||
8.2.2 Charge Transport | 261 | ||
8.2.3 Selective Contacts | 262 | ||
8.2.4 Optimized Energy Levels at Interfaces | 263 | ||
8.3 Metal Oxides for All-oxide Photovoltaics | 264 | ||
8.3.1 Electronic Properties | 264 | ||
8.3.2 Metal Oxide Light Absorber | 266 | ||
8.3.3 Wide Bandgap Metal Oxides | 267 | ||
8.4 Cu2O-based Photovoltaics | 269 | ||
8.4.1 Cu2O Synthesis | 269 | ||
8.4.2 Electronic and Optical Properties of Cu2O | 271 | ||
8.4.3 Cu2O Schittky Junction Cells | 272 | ||
8.4.4 Cu2O-based Heterojunction Cells | 274 | ||
8.4.5 Cu2O Homojunction Cells | 276 | ||
8.4.6 Nano-structured Cu2O -based Photovoltaic Cells | 276 | ||
8.5 Further Metal Oxide-based Photovoltaics | 277 | ||
8.5.1 ZnO–Fe2O3 Heterojunction Solar Cells | 277 | ||
8.5.2 Bi2O3 Solar Cells | 277 | ||
8.5.3 Ferro-electric BiFeO3 Solar Cells | 278 | ||
8.6 Combinatorial Material Science for Novel Metal Oxides | 279 | ||
8.6.1 Density Functional Theory | 279 | ||
8.6.2 Combinatorial Material and Device Fabrication | 280 | ||
References | 281 | ||
Chapter 9 Active Layer Limitations and Non-geminate Recombination in Polymer–Fullerene Bulk Heterojunction Solar Cells | 287 | ||
9.1 Introduction | 287 | ||
9.2 Active Layer Limitations | 299 | ||
9.3 Charge Transport and Recombination | 301 | ||
9.4 Non-Langevin Bimolecular Recombination | 309 | ||
9.5 Mechanism of Reduced Recombination | 314 | ||
9.6 Summary and Outlook | 318 | ||
References | 319 | ||
Chapter 10 Singlet Fission and 1,3-Diphenylisobenzofuran as a Model Chromophore | 324 | ||
10.1 Introduction | 324 | ||
10.1.1 Singlet Fission | 324 | ||
10.1.2 Singlet Fission Chromophores | 327 | ||
10.1.3 Chromophore Coupling | 328 | ||
10.2 1,3-Diphenylisobenzofuran (1) | 330 | ||
10.2.1 The Chromophore 1 | xvii | ||
10.2.2 Polycrystalline Layers of 1 | xvii | ||
10.2.3 Covalent Dimers of 1 | xvii | ||
10.3 Current and Future Activities | 341 | ||
Acknowledgements | 342 | ||
References | 342 | ||
Chapter 11 Quantum Confined Semiconductors for Enhancing Solar Photoconversion through Multiple Exciton Generation | 345 | ||
11.1 Introduction to Colloidal Quantum Dots | 345 | ||
11.1.1 Tuning of Electronic Properties | 345 | ||
11.1.2 Competition Between MEG and Hot-carrier Cooling via Phonon Emission | 347 | ||
11.1.3 Benefits to Solar Photoconversion | 350 | ||
11.2 Nanocrystal Synthesis and Physical Properties | 352 | ||
11.2.1 Solution Phase Synthesis | 352 | ||
11.2.2 Shape and Composition Control | 354 | ||
11.2.3 Measuring Multiple Exciton Generation | 358 | ||
11.3 Quantum Dot Solar Cells | 361 | ||
11.3.1 Quantum Dot Films | 361 | ||
11.3.2 Quantum Dot Material Selection | 364 | ||
11.3.3 p–n Heterojunction Quantum Dot Solar Cells | 365 | ||
11.3.4 Quantum Junction Solar Cells | 368 | ||
11.3.5 Multiple Exciton Generation in a Quantum Dot Solar Cell | 368 | ||
11.3.6 Multi-junction Solar Cells | 369 | ||
11.4 Conclusions and Future Directions | 370 | ||
Acknowledgements | 372 | ||
References | 372 | ||
Chapter 12 Hot Carrier Solar Cells | 379 | ||
12.1 Introduction to Hot Carrier cells | 379 | ||
12.2 Modelling of Hot Carrier Solar Cells | 380 | ||
12.2.1 Thermodynamic Analysis for the Hot Carrier Cell | 380 | ||
12.2.2 Models for Ideal Hot Carrier Cells | 381 | ||
12.2.3 Detailed Balance Models and Limit of Effciency | 383 | ||
12.2.4 The Mechanisms of Carrier Thermalization | 386 | ||
12.2.5 Modelling of Hot Carrier Solar Cell Efficiency | 387 | ||
12.2.6 Modelling of Non-ideal ESCs | 388 | ||
12.2.7 Monte Carlo Modelling of Real Material Systems | 391 | ||
12.2.8 Summary of Modelling Section | 394 | ||
12.3 Hot Carrier Absorbers: Slowing of Carrier Cooling | 394 | ||
12.3.1 Electron–Phonon Interactions | 395 | ||
12.3.2 Phonon Decay Mechanisms | 396 | ||
12.3.3 Nanostructures for the Absorber | 397 | ||
12.3.4 Hot Carrier Cell Absorber Requisite Properties | 399 | ||
12.4 Hot Carrier Absorber: Choice of Materials | 400 | ||
12.4.1 Analogues of InN | 400 | ||
12.4.2 Modelling Phonon Properties in Group IV and III–V compounds | 403 | ||
12.4.3 Phonon Modulation in Quantum Dot Nanostructure Arrays for Absorbers | 409 | ||
12.5 Contacting Hot Carrier Cells | 414 | ||
12.5.1 Modelling Optimized Materials for Energy Selective Contacts | 414 | ||
12.5.2 Triple Barrier Resonant Tunnelling Structures for Carrier Selection and Rectification | 417 | ||
12.5.3 Optical Coupling for Hot Carrier Cells | 418 | ||
12.6 Summary and Conclusion | 419 | ||
References | 421 | ||
Chapter 13 Intermediate Band Solar Cells | 425 | ||
13.1 Introduction | 425 | ||
13.2 Numerical Analysis of QD-IB Solar Cell Characteristics | 428 | ||
13.3 Fabrication of QD-IB Solar Cells | 431 | ||
13.3.1 Growth and Properties of High-density InAs QD Arrays on High-index Substrate | 431 | ||
13.3.2 InAs/GaAs QD-IB Solar Cells Fabricated on High-index Substrate | 437 | ||
13.3.3 Growth and Properties of InAs/GaAsSb QDs with Type-II Band Alignment | 441 | ||
13.3.4 InAs/GaAsSb QD-IB Solar Cells with Type-II Band Alignment | 444 | ||
13.3.5 Characteristics of QD-IB Solar Cells under Concentrated Sunlight | 448 | ||
13.4 Conclusion and Future Research | 449 | ||
Acknowledgements | 451 | ||
References | 452 | ||
Chapter 14 Spectral Conversion for Thin Film Solar Cells and Luminescent Solar Concentrators | 455 | ||
14.1 Introduction | 456 | ||
14.1.1 Spectral Conversion | 456 | ||
14.1.2 This Chapter | 458 | ||
14.2 Up-conversion for Thin Film Silicon | 459 | ||
14.2.1 Introduction | 459 | ||
14.2.2 Up-conversion Results | 461 | ||
14.3 Luminescent Solar Concentrators | 469 | ||
14.3.1 Operating Principles | 470 | ||
14.3.2 Effciency | 471 | ||
14.3.3 Alternative Luminescent Species | 473 | ||
14.3.4 Re-absorption | 476 | ||
14.4 Conclusion and Outlook | 483 | ||
Acknowledgements | 484 | ||
References | 484 | ||
Chapter 15 Triplet–triplet Annihilation Up-conversion | 489 | ||
15.1 Introduction | 489 | ||
15.2 The Limiting Effiency of a Single Threshold Solar Cell | 490 | ||
15.2.1 Photon Ratchet Model | 490 | ||
15.3 Up-conversion | 492 | ||
15.3.1 Summary | 495 | ||
15.4 Triplet–triplet Annihilation | 495 | ||
15.4.1 Typical TTA Up-conversion Combinations | 496 | ||
15.4.2 Efficiency Considerations | 497 | ||
15.5 Application to Photovoltaics | 499 | ||
15.6 Measurement | 500 | ||
15.7 The Figure of Merit | 502 | ||
15.8 Prospects | 503 | ||
References | 504 | ||
Chapter 16 Quantum Rectennas for Photovoltaics | 506 | ||
16.1 Introduction | 506 | ||
16.2 History of Quantum Antennas for Photovoltaics Research | 507 | ||
16.2.1 Optical and Infrared Rectennas | 507 | ||
16.2.2 Wireless Power Transmission | 511 | ||
16.2.3 Radio-powered Devices | 512 | ||
16.2.4 Radio Astronomy | 512 | ||
16.3 Research Problems Concerning Rectennas for Photovoltaics | 512 | ||
16.3.1 Fundamental Problems | 512 | ||
16.3.2 Practical Problems | 520 | ||
16.4 Thermodynamics of Rectennas | 526 | ||
16.4.1 Broadband Antenna Modeled as a Resistor | 527 | ||
16.4.2 Energetics of Thermal Rectification | 529 | ||
16.5 Quantum Rectification | 531 | ||
16.6 Broadband Rectification Effciency Limit | 534 | ||
16.7 High-frequency Rectifiers | 536 | ||
16.7.1 MIM/MIIM Rectifiers | 536 | ||
16.7.2 New Concepts for High Frequency | 537 | ||
16.8 Summary and Conclusions | 542 | ||
Acknowledgements | 543 | ||
References | 543 | ||
Chapter 17 Real World Effciency Limits: the Shockley–Queisser Model as a Starting Point | 547 | ||
17.1 Introduction | 547 | ||
17.2 Efficiency of Different Single-junction Cells and Performance Analysis Based on Empirical Criteria | 549 | ||
17.2.1 Possibilities for Technological Progress | 551 | ||
17.2.2 Current Effciency (Jsc/Jsc,max Jmp/Jsc,max and Jmp/Jsc) | 553 | ||
17.2.3 Photon Energy Loss: Present Status of Single-junction Solar Cells | 556 | ||
17.3 Fill Factor and Disorder | 563 | ||
17.4 Conclusion and Outlook | 564 | ||
Acknowledgements | 564 | ||
References | 564 | ||
Chapter 18 Grid Parity and its Implications for Energy Policy and Regulation | 567 | ||
18.1 Introduction | 567 | ||
18.1.1 Photovoltaics’ Early Promise and Progress | 567 | ||
18.1.2 Photovoltaics Goes Mainstream | 568 | ||
18.1.3 Where next for Photovoltaics | 570 | ||
18.2 What is Photovoltaics Grid Parity? | 571 | ||
18.2.1 Issues Around ‘Grid Parity’ | 573 | ||
18.3 Past and Projected Photovoltaics and Grid Cost Trajectories | 576 | ||
18.3.1 Photovoltaics Costs | 576 | ||
18.3.2 Grid Costs | 577 | ||
18.3.3 Implications for Residential Photovoltaics Systems | 578 | ||
18.3.4 Implications for Utility-scale Photovoltaics in Wholesale Energy Markets | 580 | ||
18.4 The Broader Context of Photovoltaics Deployment | 581 | ||
18.4.1 Technology | 582 | ||
18.4.2 Market Access | 582 | ||
18.4.3 Social Acceptance | 583 | ||
18.5 A Changing Context for Photovoltaics Policy Support | 583 | ||
18.5.1 The Rationale for Photovoltaics Policy Support | 583 | ||
18.5.2 Photovoltaics Specific Policy Approaches to Date | 584 | ||
18.5.3 Broader Policy Settings | 588 | ||
18.6 Implications of Photovoltaics ‘Grid Parity’ for Energy Markets | 589 | ||
18.6.1 Implications of High Photovoltaics Penetration on Other Stakeholders | 589 | ||
18.6.2 Emerging Issues and Responses | 590 | ||
18.7 Conclusion: Photovoltaics as Part of a Broader Transformation | 592 | ||
References | 593 | ||
Subject Index | 596 |