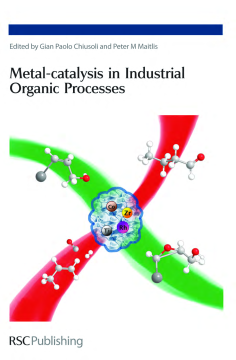
BOOK
Metal-catalysis in Industrial Organic Processes
Fausto Calderazzo | Daniel Carmona | Marta Catellani | Hans Brintzinger | Mario G Clerici | Catherine Dwyer | Gerhard Fink | Jose Fraile | Anthony Haynes | Philip Howard | George Morris | Luis A Oro | Marco Ricci | Giorgio Strukul | Glenn Sunley | Gian Paolo Chiusoli | Peter M Maitlis
(2007)
Additional Information
Book Details
Abstract
Catalysis underpins most modern industrial organic processes. It has become an essential tool in creating a 'greener' chemical industry by replacing more traditional stoichiometric reactions, which have high energy consumption and high waste production, with mild processes which increasingly resemble Nature's enzymes. Metal-Catalysis in Industrial Organic Processes considers the major areas of the field and discusses the logic of using catalysis in industrial processes. The book provides information on oxidation, hydrogenation, carbonylation, C-C bond formation, metathesis and polymerization processes, as well as on the mechanisms involved. In addition two appendices offer a concise treatment of homogeneous and heterogenous catalysis. Numerous exercises referring to problems of catalytic processes, and research perspectives complete the book. This definitive reference source, written by practising experts in the field, provides detailed and up-to-date information on key aspects of metal catalysis.
Table of Contents
Section Title | Page | Action | Price |
---|---|---|---|
Metal-Catalysis in Industrial Organic Processes | 1 | ||
Contents | 8 | ||
Preface | 6 | ||
Glossary | 18 | ||
Chapter 1 Introduction: Catalysis in the Chemical Industry | 22 | ||
1.1 Catalysis in the Chemical Industry | 22 | ||
1.1.1 The Importance of Catalysis | 22 | ||
1.1.2 Chemical Processes | 25 | ||
1.1.3 Evolution of the Catalysis Based Industries | 28 | ||
1.1.4 Applying Catalysis | 29 | ||
1.2 Selection of a Chemical Process: What Does the Catalyst Do? | 30 | ||
1.2.1 Feedstocks: Availability and Cost | 30 | ||
1.2.2 Feedstocks: Thermodynamic and Kinetic Feasibility | 32 | ||
1.2.3 Economics: The Costs of Making a Chemical | 34 | ||
1.2.4 Safety and Environmental Impact | 36 | ||
1.2.5 Product Properties and Value | 36 | ||
1.2.6 What Makes a Successful Process? | 37 | ||
1.3 Developing Metal-Catalysis – the Role of Fundamental Understanding | 38 | ||
1.3.1 Catalytic Cycles | 38 | ||
1.3.2 How we Study What a Catalyst Does | 39 | ||
1.3.3 Reaction Kinetics and the Catalytic Cycle | 39 | ||
1.3.4 Model Studies – Structures and Reactions | 41 | ||
1.3.5 How to Apply Understanding to Discovering and Improving Catalysts | 41 | ||
References | 42 | ||
Chapter 2 Formation of C–O Bonds by Oxidation | 44 | ||
2.1 Review: The Basic Chemistry of Oxygen | 44 | ||
2.1.1 Diradical Nature of the Dioxygen Molecule | 44 | ||
2.1.2 Metal-Oxygen Complexes | 45 | ||
2.1.3 Biomimetic Oxidations | 47 | ||
2.1.4 Hydrogen Peroxide and Alkylhydroperoxides | 48 | ||
2.2 Cyclohexane Oxidation to Cyclohexanol and Cyclohexanone and to Adipic Acid: on the Way to Nylon-6,6 | 49 | ||
2.2.1 KA Oil from Cyclohexane | 50 | ||
2.2.2 Adipic Acid from KA Oil | 51 | ||
2.2.3 Related Processes | 53 | ||
2.3 p-Xylene Oxidation to Terephthalic Acid. Polyethylene Terephthalate: on the Way to Fibres for Shirts | 54 | ||
2.4 Ethylene Oxide by Ag-catalyzed Oxidation of Ethylene: for Antifreeze and Detergents | 56 | ||
2.4.1 Air- and Oxygen-based Industrial Processes | 57 | ||
2.4.2 Proposed Epoxidation Mechanisms on Ag Catalysts | 58 | ||
2.4.3 Is the Epoxidation of Olefins Other than Ethylene Feasible on Silver Catalysts? | 60 | ||
2.5 Propylene Oxide: to Biocompatible Propylene Glycol | 61 | ||
2.6 Hydrogen Peroxide Route to Propylene Oxide | 64 | ||
2.7 Asymmetric Epoxidation, Dihydroxylation and Sulfide Oxidation: New Routes to Chiral Agrochemicals and Pharmaceuticals | 66 | ||
2.7.1 Epoxidation of Allylic Alcohols | 66 | ||
2.7.2 Epoxidation of Simple Olefins | 69 | ||
2.7.3 Vicinal Dihydroxylation of Olefins | 70 | ||
2.7.4 Oxidation of Sulfides to Sulfoxides: an Anti-ulcer Medication | 72 | ||
2.8 Acrolein and Acrylic Acid from Propylene: for Super-Absorbent Polymers, Paints, and Fibres | 73 | ||
2.9 Methacrolein and Methacrylic Acid from Isobutene | 75 | ||
2.10 Ammoxidation Reactions. Propylene to Acrylonitrile: for Engineering Plastics, Polymers | 76 | ||
2.10.1 Isophthalonitrile from m-Xylene | 78 | ||
2.11 Maleic Anhydride and Phthalic Anhydride: for THF, Spandex, Swim-suits and Ladies' Tights | 78 | ||
2.11.1 Maleic Anhydride | 78 | ||
2.11.2 Phthalic Anhydride | 80 | ||
2.12 Silicalite Process to ε-Caprolactam | 81 | ||
2.12.1 Ammoximation of Cyclohexanone on TS-1 | 82 | ||
2.12.2 Gas Phase Rearrangement of Cyclohexanone Oxime to ε-Caprolactam | 82 | ||
2.13 Oxidation of Phenol to Catechol and Hydroquinone | 83 | ||
2.14 Benzene Oxidation to Phenol: Making Phenolic Resins for Building | 85 | ||
2.15 Oxidation Processes in which the Metal Directly Functionalizes the Olefinic Substrate | 86 | ||
2.15.1 Ethylene to Acetaldehyde: the Wacker Synthesis | 86 | ||
2.15.2 Chemical Basis of the Wacker Process | 87 | ||
2.15.3 Wacker Process Operation | 88 | ||
2.15.4 Alternative Catalyst Formulations for Ethylene to Acetaldehyde | 89 | ||
2.15.5 Oxidation of Propylene to Acetone | 90 | ||
2.15.6 Vinyl Acetate Based on Ethylene (Solution Based Processes) | 91 | ||
2.15.7 The Gas-phase Ethylene to Vinyl Acetate Process | 91 | ||
2.15.8 Uses of Vinyl Acetate | 94 | ||
2.16 Enzymatic and Microbiological Oxidations. Microbial Hydroxylation of Progesterone | 94 | ||
2.16.1 Perspectives of Enzymatic and Microbiological Oxidations | 96 | ||
Annex 1 Alkane Feedstocks. Alternative Routes to Acetic Acid and Acrylonitrile | 97 | ||
Annex 2 Adsorption Effects on the Catalytic Performances of TS-1. Zeolites as Solid Solvents | 98 | ||
References | 99 | ||
Chapter 3 Hydrogenation Reactions | 100 | ||
3.1 Introduction and Basic Chemistry: Activation of Hydrogen and Transfer to Substrate | 100 | ||
3.1.1 Hydrides and Dihydrogen Activation | 100 | ||
3.1.2 The Reversible Addition of M–H to C=X Bonds on Model Complexes: Olefin Isomerization Reactions | 101 | ||
3.1.3 A Typical Homogeneously Catalyzed Hydrogenation Cycle: the Wilkinson Catalyst | 103 | ||
3.1.4 Isomerization of Alkenes | 104 | ||
3.1.5 Reactions on Metal Surfaces: Heterogeneously Catalyzed Hydrogenation and Isomerization | 105 | ||
3.2 Hydrotreating in Petroleum Chemistry | 106 | ||
3.2.1 Importance of Hydrotreating in Petroleum Chemistry | 106 | ||
3.2.2 The Catalytic Process | 107 | ||
3.2.3 Composition and Structure of HDS Catalysts | 108 | ||
3.2.4 Mechanistic Studies | 108 | ||
3.2.5 Deep Desulfurization | 110 | ||
3.3 Mono-unsaturated Fatty Esters by Partial Hydrogenation of Natural Oils | 111 | ||
3.3.1 Hydrogenation of Fats | 111 | ||
3.3.2 The Selectivity Problem | 112 | ||
3.3.3 Nickel Catalysts and Catalytic Processes | 112 | ||
3.4 Hydrogenation of Adiponitrile to Hexamethylenediamine | 113 | ||
3.4.1 The Uses of Hexamethylenediamine | 113 | ||
3.4.2 The Hydrogenation of Adiponitrile | 114 | ||
3.4.3 Insights into the Reaction Mechanism | 115 | ||
3.5 Making L-DOPA by Enantioselective Hydrogenation of Acetamidoarylacrylic Acids | 117 | ||
3.5.1 The Development of the Enantioselective Hydrogenation Step | 117 | ||
3.5.2 Mechanism of the Asymmetric Catalytic Hydrogenation | 120 | ||
3.6 Enantioselective Hydrogenation of N-Arylimines in the Synthesis of the Chiral Herbicide, (S)-Metolachlor | 120 | ||
3.6.1 The Synthesis of Metolachlor | 120 | ||
3.6.2 Mechanistic Studies | 123 | ||
3.7 Isomerization Reactions: Diethylgeranylamine and Diethylnerylamine for the Production of (–)-Menthol | 124 | ||
3.7.1 The Synthetic Route to Menthol | 124 | ||
3.7.2 Mechanistic Insights | 127 | ||
3.8 Enantioselective Hydrogen Transfer | 128 | ||
3.9 Ethylbenzene Dehydrogenation to Styrene | 130 | ||
3.9.1 The Styrene Market | 130 | ||
3.9.2 Ethylbenzene Non-Oxidative Dehydrogenation | 130 | ||
3.9.3 Catalysts for Ethylbenzene Dehydrogenation: Mechanism and Deactivation | 131 | ||
3.9.4 Alternatives for Non-Oxidative Dehydrogenation | 132 | ||
Discussion Points | 133 | ||
References | 134 | ||
Chapter 4 Syntheses Based on Carbon Monoxide | 135 | ||
4.1 Introduction | 135 | ||
4.1.1 Carbonylation Reactions: Historical and General Perspectives | 137 | ||
4.1.2 Syngas as a Feedstock | 138 | ||
4.1.3 The Water-Gas Shift Reaction | 139 | ||
4.2 Carbonylation Reactions of Alcohols and Esters | 139 | ||
4.2.1 Manufacture of Acetic Acid from Methanol | 140 | ||
4.2.2 Acetic Acid Historical and Background | 140 | ||
4.2.3 Cobalt-Catalyzed Carbonylation of Methanol | 141 | ||
4.2.4 Rhodium-Catalyzed Carbonylation of Methanol | 142 | ||
4.2.5 Mechanism of Rhodium/Iodide Catalyzed Methanol Carbonylation | 145 | ||
4.2.6 Iridium-Catalyzed Carbonylation of Methanol | 147 | ||
4.2.7 Mechanism of the Iridium/Iodide Catalyzed Methanol Carbonylation | 149 | ||
4.2.8 Rhodium-Catalyzed Carbonylation of Methyl Acetate to Acetic Anhydride | 151 | ||
4.2.9 Carbonylation of Higher Alcohols: Higher Carboxylic Acids | 153 | ||
4.2.10 Carbonylation of Benzyl Alcohol to Phenylacetic Acid; Manufacture of Ibuprofen | 153 | ||
4.3 Hydroxy/Alkoxy-Carbonylation of Alkenes and Dienes | 155 | ||
4.3.1 Ethylene to Propionic Acid; Methyl Propionate and Methyl Methacrylate (MMA) | 156 | ||
4.3.2 Cobalt-Catalyzed Butadiene Dimethoxycarbonylation to Dimethyl Adipate | 158 | ||
4.4 Polyketones | 159 | ||
4.5 Oxidative Carbonylation of Methanol to Dimethyl Carbonate and Dimethyl Oxalate | 160 | ||
4.6 Hydroformylation of Olefins | 162 | ||
4.6.1 Manufacture of n-Butyraldehyde and n-Butanol | 162 | ||
4.6.2 \"Unmodified\" Cobalt Catalysts | 163 | ||
4.6.3 Phosphine-Modified Cobalt Catalysts | 166 | ||
4.6.4 Rhodium-Catalyzed Hydroformylation | 167 | ||
4.6.5 Two-Phase (Water-Soluble) Rhodium Hydroformylation Catalysts | 169 | ||
4.6.6 Rhodium Hydroformylation Catalysts with Bidentate Ligands | 170 | ||
4.6.7 Enantioselective Hydroformylation | 170 | ||
4.7 CO Hydrogenation | 171 | ||
4.7.1 Methanol Synthesis | 172 | ||
4.7.2 Hydrocarbons from the Hydrogenation of CO: the Fischer-Tropsch (F-T) Reaction | 173 | ||
4.7.3 Fischer-Tropsch Technology | 176 | ||
Annex 1 Concerning the Mechanism of the Fischer-Tropsch Reaction | 177 | ||
Annex 1.1 How are 1-alkenes formed from syngas? | 177 | ||
Annex 1.2 Other mechanistic proposals | 180 | ||
Annex 1.3 Homogeneous CO hydrogenation | 181 | ||
Annex 2 Some Hints for Discussion Points | 181 | ||
References | 182 | ||
Chapter 5 Carbon–Carbon Bond Formation | 184 | ||
5.1 Introduction | 184 | ||
5.2 Alkylation and Related Reactions | 185 | ||
5.2.1 Ethylbenzene by Alkylation of Benzene with Ethylene | 185 | ||
5.2.2 Toluene Dealkylation and Methyl Redistribution to Benzene and Xylenes | 187 | ||
5.2.3 Cumene from Benzene and Propylene | 187 | ||
5.2.4 2,6-Di-isopropylnaphthalene | 188 | ||
5.2.5 Other Alkylations of Aromatics | 189 | ||
5.2.6 Alkane Cracking and Isomerization on Solid Acid Catalysts | 190 | ||
5.2.7 o–Pentenyltoluene from o–Xylene and Butadiene | 191 | ||
5.2.8 C–C Bond Formation Through Multifunctional Catalysis By Mixed Metal Oxides | 192 | ||
5.3 Carbon-Carbon Bond Formation through Activation of Aryl- or Vinyl-Halide bonds: Fine Chemicals | 193 | ||
5.3.1 Vinylarenes by Vinylation of Aromatics | 193 | ||
5.3.2 Alkynylarenes by Vinylation of Triple Bonds | 197 | ||
5.3.3 Biaryls by Aryl Coupling | 198 | ||
5.3.4 Considerations on Reactions of Aryl and Vinyl Halides | 201 | ||
5.3.5 Why Palladium? | 201 | ||
5.4 Chemistry of Allyl Compounds. Butadiene as Substrate | 203 | ||
5.4.1 1,4-Hexadiene from Butadiene and Ethylene | 203 | ||
5.4.2 Cyclooctadiene and Cyclododecatriene from Butadiene | 204 | ||
5.4.3 Octadienol from Butadiene | 207 | ||
5.4.4 Adiponitrile by HCN Addition to Butadiene | 208 | ||
5.5 Oligomerization of Olefins | 210 | ||
5.6 Carbene Chemistry and Asymmetric Synthesis: Chrysanthemic Esters | 213 | ||
Annex 1 Devising New Synthetic Pathways | 216 | ||
Annex 2 Hints to Improve or to Develop Alternative Processes for the Synthesis of Aromatics Catalyzed by Transition Metals | 217 | ||
Annex 3 Perspectives in C–C Bond Forming Organic Syntheses | 218 | ||
Annex 3.1 Catalytic Efficiency and Selectivity | 218 | ||
Annex 3.2 Reaction Media for Catalysis | 218 | ||
Annex 3.3 C-H Activation | 219 | ||
Annex 3.4 Multistep Reactions | 219 | ||
References | 220 | ||
Chapter 6 Metathesis of Olefins | 222 | ||
6.1 Introduction: History and Basic Chemistry of Metathesis | 222 | ||
6.2 The Carbene-Metallacyclobutane Mechanism of Metathesis | 224 | ||
6.3 Industrial Applications of Metathesis | 226 | ||
6.3.1 Production of Light Olefins | 226 | ||
6.3.2 The Shell Higher Olefins Process (SHOP) | 229 | ||
6.4 Homogeneous Ruthenium Alkylidene Complexes | 230 | ||
6.5 Speciality Polymers | 231 | ||
6.6 Fine Chemicals and Pharmaceuticals | 233 | ||
6.7 Recent Progress | 235 | ||
6.8 Future Outlook | 236 | ||
References | 237 | ||
Chapter 7 Polymerization Reactions | 239 | ||
7.1 An Introductory Overview | 239 | ||
7.2 Industrial Aspects of Polyolefin Production | 242 | ||
7.2.1 Polyethylene Production | 244 | ||
7.2.2 Polypropylene Production | 245 | ||
7.3 Solid-State Polymerization Catalysts | 247 | ||
7.3.1 Ziegler-Natta Catalysts | 247 | ||
7.3.2 Phillips Catalysts | 250 | ||
7.4 Soluble Olefin Polymerization Catalysts | 251 | ||
7.4.1 Activation Reactions | 252 | ||
7.4.2 Polyolefin Chain Growth | 255 | ||
7.4.3 Stereochemistry of α-Olefin Enchainment | 259 | ||
7.4.4 Chain-Growth Termination and Re-initiation | 262 | ||
7.5 Supported Metallocene Catalysts | 265 | ||
7.6 Copolymerization of Linear and Cyclic Olefins | 267 | ||
7.7 Copolymerisation of Olefins with Polar Monomers and with CO | 269 | ||
Annex 1 Polymer Stereochemistry Studied by 13C NMR Spectroscopy | 271 | ||
Annex 2 Stereospecific Polymerization of Conjugated Diolefins: Butadiene and Isoprene | 272 | ||
Annex 3 Some Hints to Help Start the Discussions | 273 | ||
Acknowledgements | 274 | ||
References | 274 | ||
Appendix 1 Basic Organometallic Chemistry Related to Catalytic Cycles | 276 | ||
A1.1 The Key Steps | 276 | ||
A1.1.1 Metal Complexes | 276 | ||
A1.1.2 Ligands in Coordination Complexes | 277 | ||
A1.1.3 Carbon Monoxide and Metal Carbonyls | 278 | ||
A1.1.4 Making Metal Carbonyls | 278 | ||
A1.1.5 Metal Olefin Complexes | 279 | ||
A1.1.6 Making Metal Complexes of Mono-Olefins and other Unsaturated Organics | 280 | ||
A1.2 Catalytic Cycles | 280 | ||
A1.2.1 Ligand Substitution | 281 | ||
A1.2.2 Formation of Metal-Carbon σ-Bonds by Oxidative Addition | 281 | ||
A1.2.3 Cleavage of Metal-Carbon σ-Bonds by Reductive Elimination | 283 | ||
A1.2.4 Formation of Metal-Carbon σ-Bonds by Nucleophilic Attack or by Migratory Insertion Reactions | 283 | ||
A1.2.5 Cleavage (Transformation) of Metal-Carbon σ-Bonds by β-Elimination | 284 | ||
A1.2.6 Analysis of a Model System: the Monsanto Carbonylation of Methanol to Acetic Acid Catalyzed by Rh/I‾ | 284 | ||
A1.3 Ligands for Asymmetric Catalysis | 285 | ||
A1.4 Metal-Carbene, -Methylene, -Carbyne and -Methylidyne Complexes | 287 | ||
A1.4.1 Synthesis, Structure and Bonding in Schrock Carbenes | 288 | ||
A1.4.2 Synthesis, Structure and Bonding in Fischer Carbenes | 288 | ||
References | 289 | ||
Appendix 2 Some Basic Aspects of Surface Science Related to Heterogeneously Catalyzed Reactions | 290 | ||
A2.1 Background | 290 | ||
A2.2 Active sites | 291 | ||
A2.3 Surface Studies | 291 | ||
A2.4 Classes of Heterogeneous Catalysts | 292 | ||
A2.4.1 Metal Catalysts | 292 | ||
A2.4.2 Metal Oxide Catalysts | 293 | ||
A2.4.3 Metal Sulfides | 296 | ||
A2.5 Catalyst Promoters | 297 | ||
A2.6 Catalyst Poisoning/Deactivation | 297 | ||
A2.7 Supported catalysts | 297 | ||
A2.8 Other Types of Non-homogeneous Catalysts | 298 | ||
A2.9 Special Topics in Heterogeneous Catalysis | 299 | ||
A2.9.1 Microporosity and Shape Selectivity | 299 | ||
A2.9.2 Adsorption and Desorption on Solid Supports and Catalysts | 300 | ||
A2.9.3 Petroleum Refining | 301 | ||
A2.9.3a Hydrocracking | 301 | ||
A2.9.3b Hydrotreating | 301 | ||
A2.9.3c Catalytic Reforming | 302 | ||
References | 302 | ||
Subject Index | 276 |