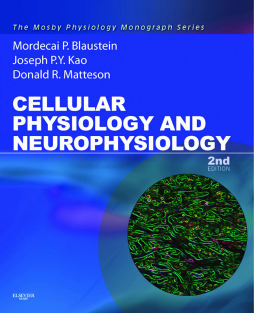
BOOK
Cellular Physiology and Neurophysiology E-Book
Mordecai P. Blaustein | Joseph P. Y. Kao | Donald R. Matteson
(2011)
Additional Information
Book Details
Abstract
Gain a quick and easy understanding of this complex subject with the 2nd edition of Cellular Physiology and Neurophysiology by doctors Mordecai P. Blaustein, Joseph PY Kao, and Donald R. Matteson. The expanded and thoroughly updated content in this Mosby Physiology Monograph Series title bridges the gap between basic biochemistry, molecular and cell biology, neuroscience, and organ and systems physiology, providing the rich, clinically oriented coverage you need to master the latest concepts in neuroscience. See how cells function in health and disease with extensive discussion of cell membranes, action potentials, membrane proteins/transporters, osmosis, and more. Intuitive and user-friendly, this title is a highly effective way to learn cellular physiology and neurophysiology.
- Focus on the clinical implications of the material with frequent examples from systems physiology, pharmacology, and pathophysiology.
- Gain a solid grasp of transport processes—which are integral to all physiological processes, yet are neglected in many other cell biology texts.
- Understand therapeutic interventions and get an updated grasp of the field with information on recently discovered molecular mechanisms.
- Conveniently explore mathematical derivations with special boxes throughout the text.
Test your knowledge of the material with an appendix of multiple-choice review questions, complete with correct answers
- Understand the latest concepts in neurophysiology with a completely new section on Synaptic Physiology.
- Learn all of the newest cellular physiology knowledge with sweeping updates throughout.
- Reference key abbreviations, symbols, and numerical constants at a glance with new appendices.
Table of Contents
Section Title | Page | Action | Price |
---|---|---|---|
Front cover | Cover | ||
Cellular physiology and neurophysiology | iii | ||
Copyright | iv | ||
Preface | v | ||
Acknowledgments | vii | ||
Table of contents | ix | ||
I Fundamental physicochemical concepts | 1 | ||
Introduction: homeostasis and cellular physiology | 1 | ||
Objectives | 1 | ||
Key words and concepts | 5 | ||
Homeostasis enables the body to survive in diverse environments | 1 | ||
The body is an ensemble of functionally and spatially distinct compartments | 2 | ||
The biological membranes that surround cells and subcellular organelles are lipid bilayers | 2 | ||
Biomembranes are formed primarily from phospholipids but may also contain cholesterol and sphingolipids | 3 | ||
Biomembranes are not uniform structures | 3 | ||
Transport processes are essential to physiological function | 4 | ||
Cellular physiology focuses on membrane-mediated processes and on muscle function | 4 | ||
Summary | 5 | ||
Bibliography | 5 | ||
Diffusion and permeability | 7 | ||
Objectives | 7 | ||
Key words and concepts | 18 | ||
Diffusion is the migration of molecules down a concentration gradient | 7 | ||
Fick’s first law of diffusion summarizes our intuitive understanding of diffusion | 7 | ||
Essential aspects of diffusion are revealed by quantitative examination of random, microscopic movements of molecules | 9 | ||
Random movements result in meandering | 9 | ||
The root-mean-squared displacement is a good measure of the progress of diffusion | 10 | ||
Square-root-of-time dependence makes diffusion ineffective for transporting molecules over large distances | 10 | ||
Diffusion constrains cell biology and physiology | 11 | ||
Fick’s first law can be used to describe diffusion across a membrane barrier | 11 | ||
The net flux through a membrane is the result of balancing influx against efflux | 14 | ||
The permeability determines how rapidly a solute can be transported through a membrane | 14 | ||
Summary | 18 | ||
Study problems | 18 | ||
Bibliography | 18 | ||
Osmotic pressure and water movement | 19 | ||
Objectives | 19 | ||
Key words and concepts | 32 | ||
Osmosis is the transport of solvent driven by a difference in solute concentration across a membrane that is impermeable t ... | 19 | ||
Water transport during osmosis leads to changes in volume | 20 | ||
Osmotic pressure drives the net transport of water during osmosis | 20 | ||
Osmotic pressure and hydrostatic pressure are functionally equivalent in their ability to drive water movement through a m ... | 22 | ||
The direction of fluid flow through the capillary wall is determined by the balance of hydrostatic and osmotic pressures, ... | 23 | ||
Only impermeant solutes can have permanent osmotic effects | 27 | ||
Transient changes in cell volume occur in response to changes in the extracellular concentration of permeant solutes | 27 | ||
Persistent changes in cell volume occur in response to changes in the extracellular concentration of impermeant solutes | 29 | ||
The amount of impermeant solute inside the cell determines the cell volume | 29 | ||
Summary | 31 | ||
Study problems | 32 | ||
Bibliography | 32 | ||
Electrical consequences of ionic gradients | 33 | ||
Objectives | 33 | ||
Key words and concepts | 46 | ||
Ions are typically present at different concentrations on opposite sides of a biomembrane | 33 | ||
Selective ionic permeability through membranes has electrical consequences: the nernst equation | 33 | ||
The stable resting membrane potential in a living cell is established by balancing multiple ionic fluxes | 37 | ||
Cell membranes are permeable to multiple ions | 37 | ||
The resting membrane potential can be quantitatively estimated by using the goldman-hodgkin-katz equation | 39 | ||
A permeant ion already in electrochemical equilibrium does not need to be included in the goldman-hodgkin-katz equation | 41 | ||
The nernst equation may be viewed as a special case of the goldman-hodgkin-katz equation | 41 | ||
Ek is the “floor” and ena is the “ceiling” of membrane potential | 42 | ||
The difference between the membrane potential and the equilibrium potential of an ion determines the direction of ion flow | 42 | ||
The cell can change its membrane potential by selectively changing membrane permeability to certain ions | 42 | ||
The donnan effect is an osmotic threat to living cells | 43 | ||
Summary | 45 | ||
Study problems | 46 | ||
Bibliography | 46 | ||
II Ion Channels and Excitable Membranes | 47 | ||
Ion channels | 47 | ||
Objectives | 47 | ||
Key words and concepts | 54 | ||
Ion channels are critical determinants of the electrical behavior of membranes | 47 | ||
Distinct types of ion channels have several common properties | 48 | ||
Ion channels increase the permeability of the membrane to ions | 48 | ||
Ion channels are integral membrane proteins that form gated pores | 49 | ||
Ion channels exhibit ionic selectivity | 49 | ||
Ion channels share structural similarities and can be grouped into gene families | 50 | ||
Channel structure is studied with biochemical and molecular biological techniques | 50 | ||
Structural details of a k+ channel are revealed by x-ray crystallography | 51 | ||
Summary | 54 | ||
Study problems | 54 | ||
Bibliography | 54 | ||
Passive electrical properties of membranes | 55 | ||
Objectives | 55 | ||
Key words and concepts | 64 | ||
The time course and spread of membrane potential changes are predicted by the passive electrical properties of the membrane | 55 | ||
The equivalent circuit of a membrane has a resistor in parallel with a capacitor | 56 | ||
Membrane conductance is established by open ion channels | 56 | ||
Capacitance reflects the ability of the membrane to separate charge | 56 | ||
Passive membrane properties produce linear current-voltage relationships | 57 | ||
Membrane capacitance affects the time course of voltage changes | 57 | ||
Ionic and capacitive currents flow when a channel opens | 57 | ||
The exponential time course of the membrane potential can be understood in terms of the passive properties of the membrane | 59 | ||
Membrane and axoplasmic resistances affect the passive spread of subthreshold electrical signals | 60 | ||
The decay of subthreshold potentials with distance can be understood in terms of the passive properties of the membrane | 61 | ||
The length constant is a measure of how far away from a stimulus site a membrane potential change will be detectable | 63 | ||
Summary | 63 | ||
Study problems | 64 | ||
Bibliography | 65 | ||
Generation and propagation of the action potential | 67 | ||
Objectives | 67 | ||
Key words and concepts | 84 | ||
The action potential is a rapid and transient depolarization of the membrane potential in electrically excitable cells | 67 | ||
Properties of action potentials can be studied with intracellular microelectrodes | 67 | ||
Ion channel function is studied with a voltage clamp | 69 | ||
Ionic currents are measured at a constant membrane potential with a voltage clamp | 69 | ||
Ionic currents are dependent on voltage and time | 71 | ||
Voltage-gated channels exhibit voltage-dependent conductances | 72 | ||
Individual ion channels have two conductance levels | 74 | ||
Na+ channels inactivate during maintained depolarization | 75 | ||
Action potentials are generated by voltage-gated na+ and k+ channels | 76 | ||
The equivalent circuit of a patch of membrane can be used to describe action potential generation | 76 | ||
The action potential is a cyclical process of channel opening and closing | 78 | ||
Both na+ channel inactivation and open voltage-gated k+ channels contribute to the refractory period | 79 | ||
Pharmacological agents that block na+ or k+ channels, or interfere with na+ channel inactivation, alter the shape of the a ... | 79 | ||
Action potential propagation occurs as a result of local circuit currents | 80 | ||
In nonmyelinated axons an action potential propagates as a continuous wave of excitation away from the initiation site | 80 | ||
Conduction velocity is influenced by the time constant, by the length constant, and by na+ current amplitude and kinetics | 81 | ||
Myelination increases action potential conduction velocity | 82 | ||
Summary | 84 | ||
Study problems | 84 | ||
Bibliography | 85 | ||
Ion channel diversity | 87 | ||
Objectives | 87 | ||
Key words and concepts | 100 | ||
Various types of ion channels help to regulate cellular processes | 87 | ||
Voltage-gated ca2+ channels contribute to electrical activity and mediate ca2+ entry into cells | 87 | ||
Ca2+ currents can be recorded with a voltage clamp | 88 | ||
Ca2+ channel blockers are useful therapeutic agents | 90 | ||
Many members of the transient receptor potential superfamily of channels mediate ca2+ entry | 91 | ||
Some members of the trpc family are receptor-operated channels | 91 | ||
K+-selective channels are the most diverse type of channel | 92 | ||
Neuronal k+ channel diversity contributes to the regulation of action potential firing patterns | 92 | ||
Rapidly inactivating voltage-gated k+ channels cause delays in action potential generation | 93 | ||
Ca2+-activated k+ channels are opened by intracellular ca2 | 95 | ||
Atp–sensitive k+ channels are involved in glucose-induced insulin secretion from pancreatic β-cells | 95 | ||
A voltage-gated k+ channel helps to repolarize the cardiac action potential | 97 | ||
Ion channel activity can be regulated by second-messenger pathways | 97 | ||
β-adrenergic receptor activation modulates l-type ca2+ channels in cardiac muscle | 99 | ||
Summary | 99 | ||
Study problems | 100 | ||
Bibliography | 101 | ||
III Solute transport | 103 | ||
Electrochemical potential energy and transport processes | 103 | ||
Objectives | 103 | ||
Key words and concepts | 111 | ||
Electrochemical potential energy drives all transport processes | 103 | ||
The relationship between force and potential energy is revealed by examining gravity | 103 | ||
A gradient in chemical potential energy gives rise to a chemical force that drives the movement of molecules | 104 | ||
An ion can have both electrical and chemical potential energy | 104 | ||
The nernst equation is a simple manifestation of the electrochemical potential | 104 | ||
How to use the electrochemical potential to analyze transport processes | 108 | ||
Summary | 111 | ||
Study problems | 111 | ||
Bibliography | 111 | ||
Passive solute transport | 113 | ||
Objectives | 113 | ||
Key words and concepts | 130 | ||
Diffusion across biological membranes is limited by lipid solubility | 113 | ||
Channel, carrier, and pump proteins mediate transport across biological membranes | 114 | ||
Transport through channels is relatively fast | 114 | ||
Channel density controls the membrane permeability to a substance | 115 | ||
The rate of transport through open channels depends on the net driving force | 115 | ||
Transport of substances through some channels is controlled by ŁŁgatingŁŁ the opening and closing of the channels | 115 | ||
Carriers are integral membrane proteins that open to only one side of the membrane at a time | 115 | ||
Carriers facilitate transport through membranes | 116 | ||
Transport by carriers exhibits kinetic properties similar to those of enzyme catalysis | 116 | ||
Coupling the transport of one solute to the ŁŁdownhillŁŁ transport of another solute enables carriers to move the ... | 119 | ||
Na+/h+ exchange is an example of na+-coupled countertransport | 119 | ||
Na+ is cotransported with a variety of solutes such as glucose and amino acids | 119 | ||
How does the electrochemical gradient for one solute affect the gradient for a cotransported solute? | 121 | ||
Glucose uptake efficiency can be increased by a change in the na+-glucose coupling ratio | 121 | ||
Net transport of some solutes across epithelia is effected by coupling two transport processes in series | 122 | ||
Various inherited defects of glucose transport have been identified | 122 | ||
Na+ is exchanged for solutes such as ca2+ and h+ by countertransport mechanisms | 123 | ||
Na+/ca2+ exchange is an example of coupled countertransport | 124 | ||
Na+/ca2+ exchange is influenced by changes in the membrane potential | 125 | ||
Na+/ca2+ exchange is regulated by several different mechanisms | 125 | ||
Intracellular ca2+ plays many important physiological roles | 126 | ||
Multiple transport systems can be functionally coupled | 126 | ||
Tertiary active transport | 129 | ||
Summary | 130 | ||
Study problems | 131 | ||
Bibliography | 131 | ||
Active transport | 133 | ||
Objectives | 133 | ||
Key words and concepts | 148 | ||
Primary active transport converts the chemical energy from atp into electrochemical potential energy stored in solute gradients | 133 | ||
Three broad classes of atpases are involved in active ion transport | 133 | ||
The plasma membrane na+ pump (na+,k+-atpase) maintains the low na+ and high k+ concentrations in the cytosol | 134 | ||
Nearly all animal cells normally maintain a high intracellular k+ concentration and a low intracellular na+ concentration | 134 | ||
The na+ pump hydrolyzes atp while transporting na+ out of the cell and k+ into the cell | 134 | ||
The na+ pump is “electrogenic” | 135 | ||
The na+ pump is the receptor for cardiotonic steroids such as ouabain and digoxin | 135 | ||
Intracellular ca2+ signaling is universal and is closely tied to ca2+ homeostasis | 136 | ||
Ca2+ storage in the sarcoplasmic/endoplasmic reticulum is mediated by a ca2+-atpase | 139 | ||
Serca has three isoforms | 139 | ||
The plasma membrane of most cells also has an atp–driven ca2+ pump | 140 | ||
The roles of the several ca2+ transporters differ in different cell types | 140 | ||
Different distributions of the ncx and pmca in the plasma membrane underlie their different functions | 140 | ||
Several other plasma membrane transport atpases are physiologically important | 141 | ||
H+,k+-atpase mediates gastric acid secretion | 141 | ||
Two cu2+-transporting atpases play essential physiological roles | 142 | ||
Atp-binding cassette transporters are a superfamily of p-type atpases | 144 | ||
Net transport across epithelial cells depends on the coupling of apical and basolateral membrane transport systems | 145 | ||
Epithelia are continuous sheets of cells | 145 | ||
Epithelia exhibit great functional diversity | 145 | ||
What are the sources of na+ for apical membrane na+-coupled solute transport? | 147 | ||
Absorption of cl− occurs by several different mechanisms | 148 | ||
Substances Can Also Be Secreted by Epithelia | 149 | ||
Net water flow is coupled to net solute flow across epithelia | 150 | ||
Summary | 153 | ||
Study problems | 154 | ||
Bibliography | 154 | ||
IV Physiology of synaptic transmission | 155 | ||
Synaptic physiology i | 155 | ||
Objectives | 155 | ||
Key words and concepts | 178 | ||
The synapse is a junction between cells that is specialized for cell-cell signaling | 155 | ||
Synaptic transmission can be either electrical or chemical | 156 | ||
Electrical synapses are designed for rapid, synchronous transmission | 156 | ||
Most synapses are chemical synapses | 157 | ||
Neurons communicate with other neurons and with muscle by releasing neurotransmitters | 159 | ||
The neuromuscular junction is a large chemical synapse | 160 | ||
Transmitter release at chemical synapses occurs in multiples of a unit size | 162 | ||
Ca2+ plays an essential role in transmitter release | 164 | ||
The synaptic vesicle cycle is a precisely choreographed process for delivering neurotransmitter into the synaptic cleft | 166 | ||
The synaptic vesicle is the organelle that concentrates, stores, and delivers neurotransmitter at the synapse | 167 | ||
Neurotransmitter-filled synaptic vesicles dock at the active zone and become “primed” for exocytosis | 167 | ||
Binding of ca2+ to synaptotagmin triggers the fusion and exocytosis of the synaptic vesicle | 169 | ||
Retrieval of the fused synaptic vesicle back into the nerve terminal can occur through clathrin-independent and clathrin-d ... | 171 | ||
Short-term synaptic plasticity is a transient, use-dependent change in the efficacy of synaptic transmission | 174 | ||
Summary | 177 | ||
Study problems | 179 | ||
Bibliography | 179 | ||
Synaptic physiology ii | 181 | ||
Objectives | 181 | ||
Key words and concepts | 207 | ||
Chemical synapses afford specificity, variety, and fine tuning of neurotransmission | 181 | ||
What is a neurotransmitter? | 181 | ||
Receptors mediate the actions of neurotransmitters in postsynaptic cells | 184 | ||
Conventional neurotransmitters activate two classes of receptors: ionotropic receptors and metabotropic receptors | 184 | ||
Acetylcholine receptors can be ionotropic or metabotropic | 186 | ||
Nicotinic acetylcholine receptors are ionotropic | 186 | ||
Muscarinic acetylcholine receptors are metabotropic | 186 | ||
Amino acid neurotransmitters mediate many excitatory and inhibitory responses in the brain | 187 | ||
Glutamate is the main excitatory neurotransmitter in the brain | 187 | ||
γ-aminobutyric acid and glycine are the main inhibitory neurotransmitters in the nervous system | 188 | ||
Neurotransmitters that bind to ionotropic receptors cause membrane conductance changes | 189 | ||
At excitatory synapses, the reversal potential is more positive than the action potential threshold | 190 | ||
Nmdar and ampar are channels with different ion selectivities and kinetics | 191 | ||
Sustained application of agonist causes desensitization of ionotropic receptors | 192 | ||
At inhibitory synapses, the reversal potential is more negative than the action potential threshold | 193 | ||
Temporal and spatial summation of postsynaptic potentials determine the outcome of synaptic transmission | 195 | ||
Synaptic transmission is terminated by several mechanisms | 196 | ||
Biogenic amines, purines, and neuropeptides are important classes of transmitters with a wide spectrum of actions | 197 | ||
Epinephrine and norepinephrine exert central and peripheral effects by activating two classes of receptors | 197 | ||
Dopaminergic transmission is important for the coordination of movement and for cognition | 198 | ||
Serotonergic transmission is important in emotion and behavior | 199 | ||
Histamine serves diverse central and peripheral functions | 200 | ||
Atp is frequently coreleased with other neurotransmitters | 200 | ||
Neuropeptide transmitters are structurally and functionally diverse | 201 | ||
Unconventional neurotransmitters modulate many complex physiological responses | 202 | ||
Unconventional neurotransmitters are secreted in nonquantal fashion | 202 | ||
Many effects of nitric oxide and carbon monoxide are mediated locally by soluble guanylyl cyclase | 202 | ||
Endocannabinoids can mediate retrograde neurotransmission | 202 | ||
Long-term synaptic potentiation and depression are persistent changes in the efficacy of synaptic transmission induced by ... | 203 | ||
Long-term potentiation is a long-lasting increase in the efficacy of transmission at excitatory synapses | 203 | ||
Long-term depression is a long-lasting decrease in the efficacy of transmission at excitatory synapses | 205 | ||
Summary | 206 | ||
Study problems | 208 | ||
Bibliography | 209 | ||
V Molecular motors and muscle contraction | 211 | ||
Molecular motors and the mechanism of muscle contraction | 211 | ||
Objectives | 211 | ||
Key words and concepts | 227 | ||
Molecular motors produce movement by converting chemical energy into kinetic energy | 211 | ||
The three types of molecular motors are myosin, kinesin, and dynein | 211 | ||
Single skeletal muscle fibers are composed of many myofibrils | 212 | ||
The sarcomere is the basic unit of contraction in skeletal muscle | 212 | ||
Sarcomeres consist of interdigitating thin and thick filaments | 212 | ||
Thick filaments are composed mostly of myosin | 214 | ||
Thin filaments in skeletal muscle are composed of four major proteins: actin, tropomyosin, troponin, and nebulin | 214 | ||
Muscle contraction results from thick and thin filaments sliding past each other (the “sliding filament” mechanism) | 215 | ||
The cross-bridge cycle powers muscle contraction | 216 | ||
In skeletal and cardiac muscles, ca2+ activates contraction by binding to the regulatory protein troponin c | 218 | ||
The structure and function of cardiac muscle and smooth muscle are distinctly different from those of skeletal muscle | 220 | ||
Cardiac muscle is striated | 220 | ||
Cardiac muscle cells require a continuous supply of energy | 220 | ||
To enable the heart to act as a pump, myocytes comprising each chamber must contract synchronously | 220 | ||
Smooth muscles are not striated | 220 | ||
In smooth muscle, elevation of intracellular ca2+ activates contraction by promoting the phosphorylation of the myosin reg ... | 223 | ||
Summary | 226 | ||
Study problems | 227 | ||
Bibliography | 227 | ||
Excitation-contraction coupling in muscle | 229 | ||
Objectives | 229 | ||
Key words and concepts | 247 | ||
Skeletal muscle contraction is initiated by a depolarization of the surface membrane | 229 | ||
Skeletal muscle has a high resting cl− permeability | 230 | ||
A single action potential causes a brief contraction called a twitch | 230 | ||
How does depolarization increase intracellular ca2+ in skeletal muscle? | 230 | ||
Direct mechanical interaction between sarcolemmal and sarcoplasmic reticulum membrane proteins mediates excitation-contrac ... | 231 | ||
In skeletal muscle, depolarization of the t-tubule membrane is required for excitation-contraction coupling | 231 | ||
In skeletal muscle, extracellular ca2+ is not required for contraction | 232 | ||
In skeletal muscle, the sarcoplasmic reticulum stores all the ca2+ needed for contraction | 232 | ||
The triad is the structure that mediates excitation-contraction coupling in skeletal muscle | 233 | ||
In skeletal muscle, excitation-contraction coupling is mechanical | 235 | ||
Skeletal muscle relaxes when ca2+ is returned to the sarcoplasmic reticulum by serca | 235 | ||
Ca2+-induced ca2+ release is central to excitation-contraction coupling in cardiac muscle | 237 | ||
In cardiac muscle, communication between the sarcoplasmic reticulum and sarcolemma occurs at dyads and peripheral couplings | 237 | ||
Cardiac excitation-contraction coupling requires extracellular ca2+ and ca2+ entry through l-type ca2+ channels (dihydropy ... | 238 | ||
Ca2+ that enters the cell during the cardiac action potential must be removed to maintain a steady state | 240 | ||
Cardiac contraction can be regulated by altering intracellular ca2 | 240 | ||
Smooth muscle excitation-contraction coupling is fundamentally different from that in skeletal and cardiac muscles | 241 | ||
Smooth muscles are highly diverse | 241 | ||
The density of innervation varies greatly among different types of smooth muscles | 241 | ||
Some smooth muscles are normally activated by depolarization | 242 | ||
Some smooth muscles can be activated without depolarization by pharmacomechanical coupling | 243 | ||
Ca2+ signaling, ca2+ sensitivity, and ca2+ balance in smooth muscle may be altered under physiological and pathophysiologi ... | 245 | ||
Summary | 246 | ||
Study problems | 247 | ||
Bibliography | 247 | ||
Mechanics of muscle contraction | 249 | ||
Objectives | 249 | ||
Key words and concepts | 268 | ||
The total force generated by a skeletal muscle can be varied | 249 | ||
Whole muscle force can be increased by recruiting motor units | 249 | ||
A single action potential produces a twitch contraction | 249 | ||
Repetitive stimulation produces fused contractions | 251 | ||
Skeletal muscle mechanics is characterized by two fundamental relationships | 252 | ||
The sliding filament mechanism underlies the length-tension curve | 253 | ||
In isotonic contractions, shortening velocity decreases as force increases | 255 | ||
There are three types of skeletal muscle motor units | 255 | ||
The force generated by cardiac muscle is regulated by mechanisms that control intracellular ca2 | 257 | ||
Cardiac muscle generates long-duration contractions | 257 | ||
Total force developed by cardiac muscle is determined by intracellular ca2 | 257 | ||
Mechanical properties of cardiac and skeletal muscle are similar but quantitatively different | 259 | ||
Cardiac and skeletal muscles have similar length-tension relationships | 259 | ||
The contractile force of the intact heart is a function of initial (end-diastolic) volume | 259 | ||
Shortening velocity is slower in cardiac than in skeletal muscle | 260 | ||
Dynamics of smooth muscle contraction differ markedly from those of skeletal and cardiac muscle | 260 | ||
Three key relationships characterize smooth muscle function | 260 | ||
The length-tension relationship in smooth muscles is consistent with the sliding filament mechanism of contraction | 260 | ||
The velocity of shortening is much lower in smooth muscle than in skeletal muscle | 261 | ||
Single actin-myosin molecular interactions reveal how smooth and skeletal muscles generate the same amount of stress despi ... | 261 | ||
Velocity of smooth muscle shortening and the amount of stress generated depend on the extent of myosin light chain phospho ... | 263 | ||
The kinetic properties of the cross-bridge cycle depend on the myosin isoforms expressed in the myocytes | 263 | ||
The relationships among intracelllular ca2+, myosin light chain phosphorylation, and force in smooth muscles is complex | 264 | ||
Tonic smooth muscles can maintain tension with little consumption of atp | 264 | ||
Perspective: Smooth muscles are functionally diverse | 265 | ||
Summary | 267 | ||
Study problems | 268 | ||
Bibliography | 269 | ||
Epilogue | 271 | ||
Bibliography | 272 | ||
A mathematical refresher | 275 | ||
Exponents | 275 | ||
Definition of exponentiation | 275 | ||
Multiplication of exponentials | 275 | ||
Meaning of the number 0 as exponent | 275 | ||
Negative numbers as exponents | 275 | ||
Division of exponentials | 276 | ||
Exponentials of exponentials | 276 | ||
Fractions as exponents | 276 | ||
Logarithms | 276 | ||
Definition of the logarithm | 276 | ||
Logarithm of a product | 277 | ||
Logarithm of an exponential | 277 | ||
Changing the base of a logarithm | 277 | ||
Solving quadratic equations | 277 | ||
Differentiation and derivatives | 278 | ||
The slope of a graph and the derivative | 278 | ||
Derivative of a constant number | 279 | ||
Differentiating the sum or difference of functions | 279 | ||
An example illustrating the computation of a derivative | 280 | ||
What makes the “natural” exponential function natural? | 280 | ||
Differentiating composite functions: the chain rule | 280 | ||
Derivative of the natural logarithm function | 281 | ||
Integration: the antiderivative and the definite integral | 281 | ||
Indefinite integral (also known as the antiderivative) | 281 | ||
Definite integral | 282 | ||
Differential equations | 283 | ||
First-order equations with separable variables | 283 | ||
Exponential decay | 283 | ||
First-order linear differential equations | 284 | ||
Root-mean-squared displacement of diffusing molecules | 287 | ||
Bibliography | 289 | ||
Summary of elementary circuit theory | 291 | ||
Cell membranes are modeled with electrical circuits | 291 | ||
Definitions of electrical parameters | 291 | ||
Electrical potential and potential difference | 291 | ||
Current | 291 | ||
Resistance and conductance | 291 | ||
Capacitance | 292 | ||
Current flow in simple circuits | 292 | ||
A battery and resistor in parallel | 292 | ||
A resistor and capacitor in parallel | 294 | ||
Answers to study problems | 299 | ||
Chapter 2 | 299 | ||
Chapter 3 | 299 | ||
Chapter 4 | 300 | ||
Chapter 5 | 301 | ||
Chapter 6 | 301 | ||
Chapter 7 | 302 | ||
Chapter 8 | 303 | ||
Chapter 9 | 304 | ||
Chapter 10 | 304 | ||
Chapter 11 | 305 | ||
Chapter 12 | 306 | ||
Chapter 13 | 306 | ||
Chapter 14 | 307 | ||
Chapter 15 | 308 | ||
Chapter 16 | 308 | ||
Review examination | 311 | ||
Answers to review examination | 323 | ||
Index | 325 | ||
A | 325 | ||
B | 326 | ||
C | 326 | ||
D | 328 | ||
E | 328 | ||
F | 329 | ||
G | 330 | ||
H | 330 | ||
I | 330 | ||
J | 331 | ||
K | 331 | ||
L | 331 | ||
M | 331 | ||
N | 332 | ||
O | 333 | ||
P | 333 | ||
Q | 334 | ||
R | 334 | ||
S | 335 | ||
T | 336 | ||
U | 337 | ||
V | 337 | ||
W | 337 | ||
X | 337 | ||
Z | 337 |